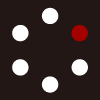
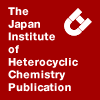
HETEROCYCLES
An International Journal for Reviews and Communications in Heterocyclic ChemistryWeb Edition ISSN: 1881-0942
Published online by The Japan Institute of Heterocyclic Chemistry
e-Journal
Full Text HTML
Received, 16th February, 2011, Accepted, 21st April, 2011, Published online, 16th May, 2011.
DOI: 10.3987/COM-11-12177
■ A Novel Conjugated Donor-Acceptor System Based on Tetrathiafulvalene Merging Pyrene Unit: Synthesis, Physical Properties and Theoretical Calculations
Chunyang Jia,* Jiaqiang Zhang, Ligong Zhang, and Xiaojun Yao
State Key Laboratory of Electronic Thin Films and Integrated Devices, School of Microelectronics and Solid-State Electronics, University of Electronic Science and Technology of China, Chengdu 610054, China
Abstract
A novel conjugated donor-acceptor compound (1) based on tetrathiafulvalene has been synthesized and fully characterized. Intramolecular charge transfer (ICT) in compound 1, arising from HOMO-LUMO singlet transitions, has been experimentally evidenced through absorption/fluorescence spectra and theoretical calculations.INTRODUCTION
Among all kinds of molecular electron donor moieties, tetrathiafulvalene (TTF) and its derivatives have been received much attention as strong π-electron donors which are capable of forming persistent cation radical and dication species sequentially.1 The molecular systems of D-A (donor-acceptor) compounds have been widely investigated especially in the last few years because of their potential applications in organic conducting materials,2 molecular electronics,3 optoelectronics,4 photovoltaics,5 and nonlinear optics.6 Undoubtedly, the important feature in such compounds is the property of intramolecular charge transfer (ICT), which clearly depends on the nature of the linkage between the redox active units. In practice, D and A components are usually linked by saturated flexible or rigid σ spacers (D-σ-A).7 Thereby, the structural D and A moieties on which the HOMO and LUMO are localized and kept apart from each other, respectively. This hinders their strong coupling in the electronic ground and excitated states. However, only a few compounds described so far have a direct link between the TTF unit and the acceptor, such as TTF-dipyridophenazine,8 TTF-phenanthroline,9 TTF-TCNQ type bithienoquinoxaline,10 TTF-perylenediimide,11 thus leading to a more efficient intramolecular charge transfer and planar structures.
Pyrene skeleton has been attracted much interest for its electron-accepting and unique luminescence properties.12 As a continuous work,8,13 this paper presents the synthesis of a conjugated D-A compound 1 (Scheme 1), containing tetrathiafulvalene and pyrene unit, together with experimental and theoretical evidences for intramolecular charge transfer.
RESULTS AND DISCUSSIONS
The synthetic8,14 pathway of compound 1 is outlined in Scheme 1. In order to assure solubility, the TTF with dithiodecyl chains is employed. Compound 1 can be synthesized via direct condensation of pyrene-4,5-dione with 5,6-diamino-2-[4,5-bis(decylthio)-1,3-dithio-2-ylidene]-benzo[d]-1,3-dithiole (3) in AcOH. 1H NMR spectroscopy, MS spectrometry and elemental analysis confirmed the structure of the D-A conjugated system.
The electrochemical properties of compound 1 are investigated by cyclic voltammetry together with reference compound R1 for comparison (Figure 1). By comparing with compound R1, the first oxidation potential of compound 1 is shifted from 0.48 to 0.65 V, and the second oxidation potential is shifted from 0.80 to 1.0 V. However, the oxidation waves of pyrene-4',5',2,3-quinoxaline and the reduction waves are absent maybe due to the measuring limitation in our experimental condition. These results can be rationalized in terms of electronic interaction between donor and acceptor moieties within the conjugated D-A system. Since TTF functional group of the molecule is involved in a delocalization path with pyrene-4',5',2,3-quinoxaline group, strong intramolecular electron interaction made the electron donating and electron accepting ability of molecule 1 decrease dramatically.
Figure 2(a) (green line) shows the UV-Vis absorption spectra of compound 1. By comparison with the spectra of compound 3 and pyrene-4',5',2,3-quinoxaline, new electronic transitions (450-650nm, centered at 519 nm) can only be observed in 1, which can be attributed to ICT transitions from donor to acceptor. The broad absorption band in this compound suggests a good electronic delocalization throughout the whole D-A system.15 By oxidation (Figure 2(b)), the generated radical cation 1•+ and dication 12+ were characterized by the disappearance of the broad ICT band at 519 nm and the emergence of new bands centered at 454, 564 and 915 nm. The low-energy absorption at 915 nm is now assigned to an intrinsic absorption of the TTF radical cation (TTF•+), which is red-shifted compared with those of other TTF derivatives.16
As comparing with pyrene-4',5',2,3-quinoxaline, compound 1 shows much weaker fluorescence (Figure 3). The fluorescence of compound 1 is quenched maybe due to the photo-induced electron transfer between TTF and pyrene-4',5',2,3-quinoxaline unit.17 After addition of Fe3+, then the TTF unit in compound 1 is oxidized to its corresponding cation and further lead to the photo-induced electron transfer between TTF and pyrene-4',5',2,3-quinoxaline unit is arrested, then the fluorescence of compound 1 starts to increase. The result suggesting that compound 1 is one of good candidates for redox-driven fluorescence molecular switch and maybe has some potential applications in future molecular devices.18
To get further insights into the molecular structure and electronic distribution of the D-A system, density functional theory (DFT) calculations are performed in gas phase on a B3LYP/6-31 G (d, p) level with Gaussian 09.19 The peripherally longer alkyl groups are removed for simplification as they are not expected to have significantly affects on the conformation and energy levels. The simplified molecule is labeled as model 1.
The optimized structure afforded by the calculations is displayed in Figure 4, which shows that the whole molecule is nearly in an identical plane with a dihedral angle between pyrene-4',5',2,3-quinoxaline and TTF ring as approximate 14°. The frontier molecular orbitals are given in Figure 5. For the HOMO of model 1, the orbital is mainly located over the TTF moiety with small contribution of pyrene-4',5',2,3-quinoxaline group; while for the LUMO, the orbital is mainly located on pyrene-4',5',2,3-quinoxaline group, both in good agreement with their expected electron-donor and electron-acceptor properties. This is a prerequisite to ICT transition and the positive shift of the oxidation potentials of the donor subunit.20
In summary, a novel D-A compound (1) based on tetrathiafulvalene has been synthesized and characterized. Cyclic voltammetry experiment demonstrates that the oxidation potentials of compound 1 are higher than those of R1, which suggests a good electronic communication between the donor and acceptor. Intramolecular charge transfer (ICT) in compound 1, arising from HOMO-LUMO singlet transitions, has been experimentally evidenced through UV-Vis spectra and theoretical calculations. The fluorescence properties of compound 1 suggest that this system has attractive potential application as molecular switch.
EXPERIMENTAL
1.General
Unless otherwise indicated, all reagents were purchased from commercial sources and used without additional purification. All reactions were performed under N2. Toluene and triethylphosphite were dried and distilled over Na. Compound 2,8 pyrene-4,5-dione14 were prepared as previously described. 1H NMR spectra were obtained on a Bruker AC 300 spectrometer operating at 300 MHz; Chemical shifts δ were calibrated against TMS as an internal standard. Mass spectrum was recorded on a FTMS 4.7T BioAPEX II for MALDI ionisation method. Elemental analyses were performed on an EA1110 Elemental Analyzer CHN Carlo Erba Instruments. FT-IR data were collected on a Perkin Elmer One FT-IR spectrometer. Absorption spectra were measured using a Hitachi (model U-3010) UV-Vis spectrophotometer. Fluorescence measurements were carried out using a Hitachi (model F-4500) spectrophotometer in a 1cm quartz cell. Cyclic voltammetry measurements were carried out on a CHI660 system with platinum as the working and counter electrodes, Ag/AgCl as the reference electrode and n-Bu4NPF6 as the supporting electrolyte.
2. Synthesis of 4’,5’-bis(decylthio)tetrathiafulvenyl-3,4-(pyrene-4',5',2,3-quinoxaline) 1:
A solution of compound 3 (100 mg, 0.16 mmol) and pyrene-4,5-dione (36.9 mg, 0.23 mmol) in AcOH (20 mL) was refluxed for 3 h under N2. After filtration, the precipitate was collected and purified by chromatography (CH2Cl2) to give compound 1 as a mauve solid (123 mg, 90%). 1H NMR (300MHz, CDCl3) δ: 0.86 (T, J = 6.6, 6H, 2×CH3), 1.26 (M, 24H, 12×CH2), 1.43 (M, 4H, 2×CH2), 1.60 (M, 4H, 2×CH2), 2.86 (T, J = 7.4, 4H, 2×SCH2), 8.00 (S, 2H, aromatic), 8.25 (M, 2H, aromatic), 8.05 (M, 4H, aromatic), 9.42 (T, 2H, aromatic). Selected IR data (cm-1, KBr pellet): 2916, 2849, 1624, 1441, 1424, 1352, 1205, 1168, 1103, 858, 831, 718; MS (MALDI-TOF) calcd for C46H52N2S6: 824.25, found 824.25 [M]+; Anal. Calcd for C46H52N2S6: C, 66.94; H, 6.35; N, 3.39. Found: C, 67.29; H, 6.50; N, 3.26.
3. Synthesis of 5,6-diamino-2-(4,5-bis(decylthio)-1,3-dithio-2-ylidene)benzo[d]-1,3-dithiole 3:
Triethylphosphite (15 mL) was added to a solution of 4,5-bis(decylthio)-1,3-dithiole-2-one (214 mg, 1.0 mmol) and 5,6-diaminobenzene-1,3-dithiole-2-thione (2) (926 mg, 2.0 mmol) in toluene (15 mL) under N2. The mixture was heated to 120 ºC and stirred for 3 h. After the reaction, the excess solvent was removed under vacuum to afford an oily red residue, which was subjected to chromatography (silica gel, CH2Cl2/EtOAc (1/8, v/v)). The crude product was further purified by recrystallization from EtOAc to give compound 3 as a yellow solid (220 mg, 35%). mp 100-102 ºC; 1H NMR (DMSO-d6): δ = 0.78-0.83 (t, 6H), 1.19-1.35 (m, 28H), 1.47-1.54 (m, 4H), 2.78-2.83 (t, 4H), 4.68 (s, 4H), 6.52 (s, 2H); Selected IR data (cm-1, KBr pellet): 3378, 2955, 2919, 2850, 1483, 1291, 859; EI-MS m/z (relative intensity): calcd for C30H48N2S6: 628, found 628 [M+]; Anal. Calcd for C30H48N2S6: C, 57.28; H, 7.69; N, 4.45. Found: C, 57.45; H, 7.77; N, 4.36.
ACKNOWLEDGEMENTS
This work was supported by the National Natural Science Foundation of China (Grant No. 20602005, 20873015).
References
1. (a) D. F. Perepichka, M. R. Bryce, E. J. L. McInnes, and J. P. Zhao, Org. Lett., 2001, 3, 1431; CrossRef (b) G. Cosquer, F. Pointillart, Y. Le Gal, S. Golhen, O. Cador, and L. Ouahab, Dalton Trans., 2009, 3495; CrossRef (c) M. Bendikov, F. Wudl, and D. F. Perepichka, Chem. Rev., 2004, 104, 4891; CrossRef (d) F. Dumur, N. Gautier, N. Gallego-Planas, Y. Şahin, E. Levillain, N. Mercier, P. Hudhomme, M. Masino, A. Girlando, V. Lloveras, J. Vidal-Gancedo, J. Veciana, and C. Rovira, J. Org. Chem., 2004, 69, 2164; CrossRef (e) N. Gautier, F. Dumur, V. Lloveras, J. Vidal-Gancedo, J. Veciana, C. Rovira, and P. Hudhomme, Angew. Chem. Int. Ed., 2003, 42, 2765; CrossRef (f) B. T. Zhao, W. B. Guo, and P. Z. Hu, Heterocycles, 2010, 81, 1661. CrossRef
2. M. Iyoda, M. Hasegawa, and Y. Miyake, Chem. Rev., 2004, 104, 50. CrossRef
3. (a) J. K. Sørensen, M. Vestergaard, A. Kadziola, K. Kilså, and M. B. Nielsen, Org. Lett., 2006, 8, 1173; CrossRef (b) D. Canevet, M. Salle, G. Zhang, D. Zhang, and D. Zhu, Chem. Commun., 2009, 2245; CrossRef (c) K. A. Nielsen, G. H. Sarova, L. Martín-Gomis, F. Fernández-Lázaro, P. C. Stein, L. Sanguinet, E. Levillain, J. L. Sessler, D. M. Guldi, Á. Sastre-Santos, and J. O. Jeppesen, J. Am. Chem. Soc., 2007, 130, 460. CrossRef
4. (a) D. C. Magri, New J. Chem., 2009, 33, 457; CrossRef (b) H. Imahori, Org. Biomol. Chem., 2004, 2, 1425. CrossRef
5. (a) N. Martín, L. Sánchez, M. Á. Herranz, B. Illescas, and D. M. Guldi, Acc. Chem. Res., 2007, 40, 1015; CrossRef (b) S. Wenger, P.-A. Bouit, Q. Chen, J. l. Teuscher, D. D. Censo, R. Humphry-Baker, J.-E. Moser, J. L. Delgado, N. Martín, S. M. Zakeeruddin, and M. Grätzel, J. Am. Chem. Soc., 2010, 132, 5164. CrossRef
6. (a) B. Insuasty, C. Atienza, C. Seoane, N. Martín, J. Garín, J. Orduna, R. Alcalá, and B. Villacampa, J. Org. Chem., 2004, 69, 6986; CrossRef (b) M. González, J. L. Segura, C. Seoane, N. Martín, J. Garín, J. Orduna, R. Alcalá, B. Villacampa, V. Hernández, and J. T. López Navarrete, J. Org. Chem., 2001, 66, 8872; CrossRef (c) A. I. de Lucas, N. Martín, L. Sánchez, C. Seoane, R. Andreu, J. Garín, J. Orduna, R. Alcalá, and B. Villacampa, Tetrahedron, 1998, 54, 4655; CrossRef (d) R. Andreu, A. I. de Lucas, J. Garín, N. Martín, J. Orduna, L. Sánchez, and C. Seoane, Synth. Met., 1997, 86, 1817; CrossRef (e) J. F. Lamère, I. Malfant, A. Sournia-Saquet, P. G. Lacroix, J. M. Fabre, L. Kaboub, T. Abbaz, A.-K. Gouasmia, I. Asselberghs, and K. Clays, Chem. Mater., 2007, 19, 805. CrossRef
7. (a) Y. Zhang, L.-Z. Cai, C.-Y. Wang, G.-Q. Lai, and Y.-J. Shen, New J. Chem., 2008, 32, 1968; CrossRef (b) J. Baffreau, F. Dumur, and P. Hudhomme, Org. Lett., 2006, 8, 1307; CrossRef (c) E. Tsiperman, J. Y. Becker, V. Khodorkovsky, A. Shames, and L. Shapiro, Angew. Chem. Int. Ed., 2005, 44, 4015; CrossRef (d) D. F. Perepichka, M. R. Bryce, C. Pearson, M. C. Petty, E. J. L. McInnes, and J. P. Zhao, Angew. Chem. Int. Ed., 2003, 42, 4636; CrossRef (e) R. B. Hou, H. Qiu, T. Chen, and B. Z. Yin, Heterocycles, 2009, 78, 1799. CrossRef
8. C. Jia, S.-X. Liu, C. Tanner, C. Leiggener, A. Neels, L. Sanguinet, E. Levillain, S. Leutwyler, A. Hauser, and S. Decurtins, Chem. Eur. J., 2007, 13, 3804. CrossRef
9. (a) C. Goze, N. Dupont, E. Beitler, C. Leiggener, H. Jia, P. Monbaron, S.-X. Liu, A. Neels, A. Hauser, and S. Decurtins, Inorg. Chem., 2008, 47, 11010; CrossRef (b) L. K. Keniley, L. Ray, K. Kovnir, L. A. Dellinger, J. M. Hoyt, and M. Shatruk, Inorg. Chem., 2010, 49, 1307. CrossRef
10. X. Guégano, Alexander L. Kanibolotsky, C. Blum, S. F. L. Mertens, S.-X. Liu, A. Neels, H. Hagemann, P. J. Skabara, S. Leutwyler, T. Wandlowski, A. Hauser, and S. Decurtins, Chem. Eur. J., 2009, 15, 63.
11. M. Jaggi, C. Blum, N. Dupont, J. Grilj, S.-X. Liu, J. Hauser, A. Hauser, and S. Decurtins, Org. Lett., 2009, 11, 3096. CrossRef
12. Z. Ning, Q. Zhang, W. Wu, H. Pei, B. Liu, and H. Tian, J. Org. Chem., 2008, 73, 3791. CrossRef
13. (a) C. Jia, D. Zhang, W. Xu, and D. Zhu, Org. Lett., 2001, 3, 1941; CrossRef (b) C. Jia, D. Zhang, W. Xu, X. Shao, and D. Zhu, Synth. Met., 2003, 137, 1345; CrossRef (c) C. Jia, D. Zhang, C.-M. Liu, W. Xu, H. Hu, and D. Zhu, New J. Chem., 2002, 26, 490; CrossRef (d) C. Jia, S.-X. Liu, C. Tanner, C. Leiggener, L. Sanguinet, E. Levillain, S. Leutwyler, A. Hauser, and S. Decurtins, Chem. Commun., 2006, 1878; CrossRef (e) C. Jia, S.-X. Liu, A. Neels, G. Labat, and S. Decurtins, Polyhedron, 2005, 24, 3032; CrossRef (f) C. Jia, S.-X. Liu, C. Ambrus, A. Neels, G. Labat, and S. Decurtins, Inorg. Chem., 2006, 45, 3152. CrossRef
14. J. Hu, D. Zhang, and F. W. Harris, J. Org. Chem., 2005, 70, 707. CrossRef
15. Y.-P. Zhao, L.-Z. Wu, G. Si, Y. Liu, H. Xue, L.-P. Zhang, and C.-H. Tung, J. Org. Chem., 2007, 72, 3632. CrossRef
16. (a) Y. Zeng, G. Zhang, D. Zhang, and D. Zhu, J. Org. Chem., 2009, 74, 4375; CrossRef (b) H. Wu, D. Zhang, L. Su, K. Ohkubo, C. Zhang, S. Yin, L. Mao, Z. Shuai, S. Fukuzumi, and D. Zhu, J. Am. Chem. Soc., 2007, 129, 6839. CrossRef
17. G. Zhang, D. Zhang, X. Guo, and D. Zhu, Org. Lett., 2004, 6, 1209. CrossRef
18. S. Leroy-Lhez, J. Baffreau, L. Perrin, E. Levillain, M. Allain, M.-J. Blesa, and P. Hudhomme, J. Org. Chem., 2005, 70, 6313. CrossRef
19. R. A. Gaussian 09, M. J. Frisch, G. W. Trucks, H. B. Schlegel, G. E. Scuseria, M. A. Robb, J. R. Cheeseman, G. Scalmani, V. Barone, B. Mennucci, G. A. Petersson, H. Nakatsuji, M. Caricato, X. Li, H. P. Hratchian, A. F. Izmaylov, J. Bloino, G. Zheng, J. L. Sonnenberg, M. Hada, M. Ehara, K. Toyota, R. Fukuda, J. Hasegawa, M. Ishida, T. Nakajima, Y. Honda, O. Kitao, H. Nakai, T. Vreven, J. A. Montgomery, Jr., J. E. Peralta, F. Ogliaro, M. Bearpark, J. J. Heyd, E. Brothers, K. N. Kudin, V. N. Staroverov, R. Kobayashi, J. Normand, K. Raghavachari, A. Rendell, J. C. Burant, S. S. Iyengar, J. Tomasi, M. Cossi, N. Rega, J. M. Millam, M. Klene, J. E. Knox, J. B. Cross, V. Bakken, C. Adamo, J. Jaramillo, R. Gomperts, R. E. Stratmann, O. Yazyev, A. J. Austin, R. Cammi, C. Pomelli, J. W. Ochterski, R. L. Martin, K. Morokuma, V. G. Zakrzewski, G. A. Voth, P. Salvador, J. J. Dannenberg, S. Dapprich, A. D. Daniels, O. Farkas, J. B. Foresman, J. V. Ortiz, J. Cioslowski, and D. J. Fox, Gaussian, Inc., Wallingford CT, 2009.
20. Q.-Y. Zhu, L.-B. Huo, Y.-R. Qin, Y.-P. Zhang, Z.-J. Lu, J.-P. Wang, and J. Dai, J. Phys. Chem. B, 2010, 114, 361. CrossRef