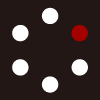
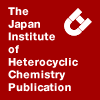
HETEROCYCLES
An International Journal for Reviews and Communications in Heterocyclic ChemistryWeb Edition ISSN: 1881-0942
Published online by The Japan Institute of Heterocyclic Chemistry
e-Journal
Full Text HTML
Received, 16th February, 2011, Accepted, 23rd March, 2011, Published online, 25th March, 2011.
DOI: 10.3987/COM-11-12178
■ Regioselective One-Pot Synthesis of 2-Aryl-6-bromobenzothiazole from Arylaldehyde and 2-Aminothiophenol with Phenyltrimethylammonium Tribromide in the Presence of a Catalytic Amount of Antimony(III) Bromide
Shinsei Sayama*
Department of Chemistry, Fukushima Medical University, Hikarigaoka, Fukushima, 960-1295, Japan
Abstract
Various 2-aryl-6-bromo-1,3-benzothiazoles were regioselectively afforded in good yields by the reaction of arylaldehydes and 2-aminothiophenol with phenyltrimethylammonium tribromide in the presence of a catalytic amount of SbBr3 in CH2Cl2 at room temperature.2- or 6-Substituted 1,3-benzothiazoles are important class of biologically active compounds in medicinal chemistry.1,2 Therefore, there have been many reports for the synthetic procedures of 2- and 6-substituted 1,3-benzothiazoles.2,3 The synthesis of 6-halogenated benzothiazoles such as 2-aryl-6- chloro-1,3-benzothiazoles employing Suzuki-Miyaura coupling reaction was especially well studied by Heo and co-workers.3d As 6-halogenated 1,3-benzothiazoles are useful as key intermadiates for the syntheses of other intricate structure of 6-substitutd 1,3-benzothiazoles,1a there has been considerable interest in continuing investigating regioselective synthesis of 6-halogenated 1,3-benzothiazoles.
On the other hand, phenyltrimethylammonium tribromide (PTAB) and pyridinium hydrobromide perbromide (PHPB) were reported to be useful for the esterification of aldehyde and for the syntheses of 2-substituted imidazolines and oxazolines from aldehyde in water.4a,4b PTAB and PHPB were also useful for the chemoselective conversion of 3-alkoxyfurans to 2-alkoxy-3(2H)-furanones.4c,4d Further, the PTAB-SbBr3–pyridine (Py) system was also reported to be convenient and chemoselective for the oxidation of secondary alcohols5a and for the oxidative conversion of aromatic epoxide and 1,2-diol to 1,3-dioxane derivatives.5b Therefore, there has been much interest in further applications for the alternative one-pot synthesis of 6-bromo-1,3-benzothiazoles with PTAB in the presence of SbBr3.6-8 We would like to report the results of our studies concerning the regioselective one-pot synthesis of 2-aryl-6-bromo-1,3-benzothiazoles from arylaldehydes and 2-aminothiophenol with PTAB-SbBr3-Py in CH2Cl2.
At first, the reaction of 2-aminothiophenol (1) and p-bromobenzaldehyde (2), chosen as a representative aldehyde for this study, was carried out with various molar ratios of PTAB, SbBr3 and Py over 2 for obtaining bromobenzothiazole. The results are summarized in Table 1. At 4.0 molar ratio of PTAB and Py over 2 in the presence of 0.2 molar equivalent of SbBr3, 6-bromo-1,3-benzothiazole (2a) was predominantly afforded (run 1). The reaction of 2 at 2.0 or 3.0 molar equivalents of PTAB over 2, was not brought about the desired yields of 2a, accompanied by benzothiazole (2b) and recovered 2 (runs 2, 3). In the present experiments, there is need to use 4.0 molar equivalent of PTAB over 2 for obtaining 6-bromo-2-(4-bromophenyl)-1,3-benzothiazole 2a in good yield.
To examine the solvent effect of CH2Cl2 in this method, the reaction of 1 and 2 was carried out in various solvents under the same reaction conditions. In hexane the reaction of 1 and 2 did not give 2a in high yield, accompanied by a mixture of benzothiazole 2b and 2 (run 9). Accordingly it was suggested that polar solvents such as MeOH, acetonitrile, DMSO promoted bromination of benzothiaoles. In MeOH or DMSO, bromobenzothiazole 2a was afforded, but with less satisfactory yields of 2a (runs 10, 11). The reaction of 1 and 2 in acetonitrile, took place to give 2a in good yield (run 12). CH2Cl2 and MeCN were found to be more effective for the one-pot conversion of p-bromobenzaldehyde 2 to 6-bromo-2- (4-bromophenyl)-1,3-benzothiazole 2a with PTAB-SbBr3-Py than that of other solvents.
To clarify the effects of PTAB, SbBr3 and Py in this system, the reaction of aminothiophenol 1 and p-bromobenzaldehyde 2 without using PTAB, SbBr3, or Py was carried out respectively. Obviously bromobenzothiazole 2a was not afforded without PTAB (run 4). Similarly the yields of 2a were not fully satisfactory without Py (runs, 6, 8). Further, the satisfactory yields of 2a without SbBr3 were not observed accompanied by benzothiazole 2b (runs 7, 8). This one-pot synthesis of 6-bromobenzothiazole was suggested to rest on the complemental function of PTAB, SbBr3, and Py.
To clarify the limitations for this conversion of aldehyde to 6-bromo-1,3-benzothiazole, the reaction of various aldehydes and aminothiophenol 1 with PTAB-SbBr3-Py was examined under the same reaction conditions. The results are summarized in Table 2. Benzaldehyde (3) was converted to 6-bromobenzo- thiazole (3a) in good yield (run 1). The reaction of o-, m-, p-chlorobenzaldehydes (4), (5), and (6) took place to give the corresponding bromobenzothiazoles (4a), (5a), and (6a) (runs 2, 3, 4). o-, m- Bromobenzaldehydes (7) and (8) were also converted to corresponding 6-bromobenzothiazoles (7a) and (8a) (runs 5, 6), as expected. 6-Bromobenzothiazoles (9a), (10a), (11a) were easily afforded from o-, m-, p-tolualdehydes (9), (10), and (11) respectively (runs 7, 8, 9). 3,4-Dimethylbenzaldehyde (12) was similarly converted to bromobenzothiazole (12a) in good yield (run 10). Bromobenzothiazoles (13a) and (14a) were easily obtained from 4-methoxybenzaldehyde (13) and 3,4-dimethoxybenzaldehyde (14) (runs 11, 12). The reaction of naphthaldehyde (15) also afforded bromobenzothiazole (15a) (run 13). Thus, various arylaldehydes were found to be regioselectively converted to corresponding 6-bromobenzothiazoles in good yields.
In contrast, alkylaldehydes (16), (17), (18), and (19) were also converted to corresponding 6-bromobenzothiazoles (16a), (17a), (18a), and (19a), but less satisfactory yields of 6-bromobenzothiazoles (runs 14-17).9
Further, to examine the superiority of SbBr3 in this method, the reaction of 1 and p-chlorobenzaldehyde 6 was carried out with other metal halides such as SbCl3, CuBr2, NiBr2, ZnBr2 under the same reaction conditions. The yield of 6-bromobenzothiazole 6a with SbCl3 was satisfactory as expected (79%). On the contrary, the yields of 6a with other metal halides such as CuBr2, NiBr2, ZnBr2 were less than 60% under the same reaction conditions. Accordingly, antimony halides SbBr3 and SbCl3 were ascertained to be essential and effective for regioselective synthesis of 6-bromobenzothiazoles from arylaldehydes in CH2Cl2 at room temperature. Consequently, the system PTAB-SbBr3-Py was confirmed to be a regioselective one-pot procedure for synthesis of 2-aryl-6-bromo-1,3-benzothiazoles from various arylaldehydes without overbromination of benzothiazole ring.10
The combination of PTAB and SbBr3 appeared to generate Br+ and SbBr4- via SbBr5.11 This selective bromination at 6-position of 1,3-benzothiazole ring was accounted for by a Br+ substitution of aromatic ring on benzothiazoline intermediate or 2-aminothiophenol as follows. The reaction of 2-methyl-1,3- benzothiazole 21 with PTAB-SbBr3-Py recovered 21 unchanged.12 In contrast, the reaction of 2-aminothiophenol 1 under the same reaction conditions took place to give a complex mixture of bromo derivatives and related compounds. Therefore, it can be assumed that an electrophilic attack of aromatic ring on benzothiazoline intermediate or 2-aminothiophenol by Br+ possibly causes selective bromination at 6-position of 1,3-benzothiazole ring in this method, and successively produces a π complex, a σ cmplex, and bromo derivative after loss of proton by SbBr4-. Loss of proton from the intermediate bromine-containing cation results in an additional formation of HBr and SbBr3 as a catalyst.
In addition 6-bromo-1,3-benzothiazole nucleus is of particular interest in organic synthesis and medicinal chemistry for applications in drug discovery.1,3d,3l Since 6-halogenated 1,3-benzothiazoles as intermediates are seemed to be useful for the syntheses of those biologically active 6-substituted 1,3-benzothiazoles via Suzuki-Miyaura coupling reaction or aromatic substitution reactions, the system PTAB-SbBr3-Py provides an alternative significant method for the key intermediates syntheses of various biologically active 1,3-benzothiazoles.
ACKNOWLEDGEMENTS
This work was supported by a grant-in-aid from the Foundation for Japanese Chemical Research (No. 383R).
References
1. (a) C. A. Mathis, Y. Wang, D. P. Holt, G.-F. Huang, M. L. Debnath, and W. E. Klunk, J. Med. Chem., 2003, 46, 2740; CrossRef (b) I. Hutchinson, M.-S. Chua, H. L. Browne, V. Trapani, T. D. Bradshaw, A. D. Westwell, and M. F. G. Stevens, J. Med. Chem., 2001, 44, 1446; CrossRef (c) Y. Kumar, R. Green, K. Z. Borysko, D. S. Wise, L. L. Wotring, and L. B. Townsend, J. Med. Chem., 1993, 36, 3843; CrossRef (d) M. Chakrabarty, T. Kundu, S. Arima, and Y. Harigaya, Tetrahedron Lett., 2005, 46, 2865. CrossRef
2. (a) H. Ulrich, ‘Science of Synthesis’, Thieme, Stuttgart, 2001, Vol. 11, p. 835; (b) A. K. Chakraborti, S. Rudrawar, G. Kaur, and L. Sharma, Synlett, 2004, 1533, and references cited therein; CrossRef (c) A. K. Chakraborti, C. Selvam, G. Kaur, and S. Bhagat, Synlett, 2004, 851; CrossRef (d) C. M. So, C. P. Lau, and F. Y. Kwong, Angew. Chem. Int. Ed., 2008, 47, 8059; CrossRef (e) C. M. So, H. W. Lee, C. P. Lau, and F. Y. Kwong, Org. Lett., 2009, 11, 317; CrossRef (f) Y. Yin, L. Lin, C. Ruiz, M. D. Cameron, J. Pocas, W. Grant, T. Schroter, W. Chen, D. Duckett, S. Schurer, P. LoGrasso, and Y. Feng, Bioorg. Med. Chem. Lett., 2009, 19, 6686; CrossRef (g) A. Akwabi-Ameyaw, J. Y. Bass, R. D. Caldwell, J. A. Caravella, L. Chen, K. L. Creech, D. N. Deaton, K. P. Madauss, H. B. Marr, R. B. McFadyen, A. B. Miller, F. Navas III, D. J. Parks, P. K. Spearing, D. Todd, S. P. Williams, and G. B.Wisely, Bioorg. Med. Chem. Lett., 2009, 19, 4733; CrossRef (h) S. D. Barchechath, R. I. Tawatao, M. Corr, D. A. Carson, and H. B. Cottam, J. Med. Chem., 2005, 48, 6409. CrossRef
3. (a) D. S. Bose, M. Idress, and B. Srikanth, Synthesis, 2007, 819; CrossRef (b) D. S. Bose and M. Idress, Tetrahedron Lett., 2007, 48, 669; CrossRef (c) K. Ikegai, W. Pluempanupat, and T. Mukaiyama, Chem. Lett., 2005, 34, 638; CrossRef (d) Y. Heo, Y. S. Song, B. T. Kim, and J.-N. Heo, Tetrahedron Lett., 2006, 47, 3091; CrossRef (e) D. Bernardi, L. A. Ba, and G. Kirsch, Synlett, 2007, 2121; CrossRef (f) C. Mukhopadhyay and A. Datta, Heterocycles, 2007, 71, 1837; CrossRef (g) H. Ma, X. Han, Y. Wang, and J. Wang, Heterocycles, 2007, 71, 1821; CrossRef (h) L. E. Kaim, M. Gizolme, L. Grimaud, and J. Oble, Synlett, 2007, 465; CrossRef (i) F. Ge, Z. Wang, W. Wan, W. Lu, and J. Hao, Tetrahedron Lett., 2007, 48, 3251; CrossRef (j) Y. Kawashita, C. Ueba, and M. Hayashi, Tetrahedron Lett., 2006, 47, 4231; CrossRef (k) Y. Li, Y.-L.Wang, and J.-Y.Wang, Chem. Lett., 2006, 35, 460; CrossRef (l) D. Alagille, R. M. Baldwin, G. D. Tamagnan, Tetrahedron Lett., 2005, 46, 1349; CrossRef (m) B. C. Ranu, R. Jana, and S. S. Dey, Chem. Lett., 2004, 33, 274; CrossRef (n) R. M. F. Batista, S. P. G. Costa, and M. M. M. Raposo, Tetrahedron Lett., 2004, 45, 2825; CrossRef (o) T. Itoh, K. Nagata, H. Ishikawa, and A. Osawa, Heterocycles, 2004, 62, 197; CrossRef (p) M. Kodomari, Y. Tamaru, and T. Aoyama, Synth. Commun., 2004, 34, 3029; CrossRef (q) C. Benedi, F. Bravo, P. Uriz, E. Fernandez, C. Claver, and S. Castillon, Tetrahedron Lett., 2003, 44, 607; CrossRef (r) R. H. Tale, Org. Lett., 2002, 4, 1641; CrossRef (s) K. Bougrin, A. Loupy, and M. Soufiaoui, Tetrahedron, 1998, 54, 8055; CrossRef (t) A. Ben-Alloum, S. Bakkas, and M. Soufiaoui, Tetrahedron Lett., 1997, 38, 6395; CrossRef (u) K. Takagi, Chem. Lett., 1986, 265. CrossRef
4. (a) S. Sayama, Synlett, 2006, 1479; CrossRef (b) S. Sayama and T. Onami, Synlett, 2004, 2739; CrossRef (c) S. Sayama, Heterocycles, 2005, 65, 1347; CrossRef (d) S. Sayama, Synth. Commun., 2007, 37, 3067. CrossRef
5. (a) S. Sayama and T. Onami, Synlett, 2004, 2369; CrossRef (b) S. Sayama, Tetrahedron Lett., 2006, 47, 4001. CrossRef
6. (a) S. Sayama and Y. Inamura, Bull. Chem. Soc. Jpn., 1991, 64, 306; CrossRef (b) S. Sayama and Y. Inamura, Chem. Lett., 1996, 633; CrossRef (c) S. Sayama, Synth. Commun., 2005, 35, 2115. CrossRef
7. (a) E. Mondal, P. R. Sahu, G. Bose, and A. T. Khan, Tetrahedron Lett., 2002, 43, 2843; CrossRef (b) V. Kavala, S. Naik, and B. K. Patel, J. Org. Chem., 2005, 70, 4267; CrossRef (c) M. K. Chaudhuri, A. T. Khan, B. K. Patel, D. Dey, W. Kharmawophlang, T. R. Lakshmiprabha, and G. Mandal, Tetrahedron Lett., 1998, 39, 8163; CrossRef (d) W. P. Reeves and R. M. King, II, Synth. Commun., 1993, 23, 855; CrossRef (e) G. S. Bates and J. O’Doherty, J. Org. Chem., 1981, 46, 1745. CrossRef
8. (a) W. Wang, L. Shi, and Y. Huang, Tetrahedron, 1990, 46, 3315; CrossRef (b) I. Cepanec, M. Litvic, M. Filipan-Litvic, and I. Grungold, Tetrahedron, 2007, 63, 11822; CrossRef (c) G. Maiti and P. Kundu, Synth. Commun., 2007, 37, 2309; CrossRef (d) Q. Wu, Y. Wang, W. Chen, and H. Liu, Synth. Commun., 2006, 36, 1361; CrossRef (e) F. Alonso, I. P. Beletskaya, and M. Yus, Chem. Rev., 2002, 102, 4009. CrossRef
9. The structure of 6-bromo-1,3-benzothiazole was determined on the basis of 1H and 13C NMR spectral data. For example, the structure of 6-bromo-2-methyl-1,3-benzothiazole (19a) was confirmed by spectral data in comparison with that of authentic 5-bromo-2-methyl-1,3-benzothiazole (20) as follows; 19a: IR (neat, cm-1) 3046, 2918, 1542, 1518, 1434, 1587, 1400, 1373, 1303, 1269, 1234, 1173, 1078, 1048, 994, 848, 810, 744. 1H NMR (CDCl3) δ 2.82 (3H, s), 7.54 (1H, dd, J = 8.1, 2.7 Hz), 7.80 (1H, d, J = 8.1), 7.95 (1H, d, J = 2.7). 13C NMR (CDCl3) δ 20.07, 118.21, 123.47, 129.32, 129.36, 137.30, 152.24, 167.50. Anal. Calcd for C8H6NSBr: C, 42.12; H, 2.65; N, 6.14. Found: C, 42.16; H, 2.69; N, 6.00. 20: 1H NMR (CDCl3) δ 2.83 (3H, s), 7.45 (1H, dd, J = 8.1, 2.7 Hz), 7.67 (1H, d, J = 8.1), 8.09 (1H, d, J = 2.7). 13C NMR (CDCl3) δ 20.15, 119.47, 122.37, 125.30, 127.77, 134.41, 154.53, 168.71..
10. Typical procedure: To a solution of PTAB (376 mg, 1.0 mmol), SbBr3 (18 mg, 0.05 mmol), and Py (80μL, 1.0 mmol) in CH2Cl2 (6 mL) were added 3-chlorobenzaldehyde (5, 35 mg, 0.25 mmol) and 2-aminothiophenol [1, 41 mg (35 μL), 0.3 mmol]. After stirring for 16 h at rt, the reaction mixture was treated with 0.5 M aq Na2S2O3 and extracted with EtOAc. The organic layer was washed with 0.5 M aq Na2S2O3 and successively washed with sat. aq. NaCl, and dried over MgSO4. After removal of solvent in vacuo, the residue was purified by column chromatography on silica gel (Wakogel C-200) with CCl4. 6-bromo-2-(3-chlorophenyl)-1,3-benzothiazole (5a) (67 mg, 0.206 mmol) was obtained in 82% yield. 5a: IR (KBr, cm-1) 3054, 1584, 1568, 1541, 1508, 1458, 1438, 1424, 1398, 1305, 1238, 1227, 1090, 1078, 997, 895, 853, 819, 779, 732. 1H NMR (CDCl3) δ 7.40-7.49 (2H, m), 7.60 (1H, dd, J = 8.1, 2.7 Hz), 7.90-7.93 (2H, m), 8.04-8.10 (2H, m). 13C NMR (CDCl3) δ 119.19, 124.23, 124.50, 125.68, 127.40, 130.08, 130.32, 131.16, 134.81, 135.36, 136.66, 152.82, 166.85. Anal. Calcd for C13H7NSBrCl: C, 48.09; H, 2.17; N, 4.31. Found: C, 48.17; H, 2.25; N, 4.25.
11. (a) J. Yamamoto, S. Ito, T. Tsuboi, T. Tsuboi, and K. Tukihara, Bull. Chem. Soc. Jpn., 1985, 58, 470; CrossRef (b) K.-y. Akiba, A. Shimizu, H. Ohnari, and K. Ohkata, Tetrahedron Lett., 1985, 26, 3211; CrossRef (c) K.-y. Akiba, H. Ohnari, and K. Ohkata, Chem. Lett., 1985, 1577; CrossRef (d) Y. Huang, Y. Shen, and C. Chen, Synthesis, 1985, 651; CrossRef (e) K. Ishihara, ‘Lewis Acids in Organic Synthesis’, Vol. 2, ed. by H. Yamamoto, Wiley-VCH, New York, 2000, p. 523. CrossRef
12. A reaction of 2-methyl-1,3-benzothiazole (21) with pyridinium hydrobromide perbromide in H2O afforded a mixture of 6-, 5-, and 4-bromo-2-methyl-1,3-benzothiazoles; S. Sayama, presented at 40th Congress of Heterocyclic Chemistry, Sendai, Japan, 2010, Abstr., p. 133.