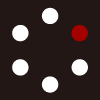
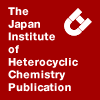
HETEROCYCLES
An International Journal for Reviews and Communications in Heterocyclic ChemistryWeb Edition ISSN: 1881-0942
Published online by The Japan Institute of Heterocyclic Chemistry
e-Journal
Full Text HTML
Received, 25th February, 2011, Accepted, 30th March, 2011, Published online, 13th April, 2011.
DOI: 10.3987/COM-11-12189
■ Synthesis of 1-Deoxyeldecalcitol, a Biologically Interesting Analog of 1α,25-Dihydroxy-2β-(3-hydroxypropoxy)vitamin D3 (Eldecalcitol)
Hirotaka Sasaki, Kohei Eto, Keisuke Takahashi, Jun Ishihara, Susumi Hatakeyama, and Noboru Kubodera*
Chugai Pharmaceutical Company, Ltd., 2-1-1, Nihonbashi-Muromachi, Chuo-ku, Tokyo 103-8324, Japan
Abstract
A synthesis of biologically interesting 1-deoxyeldecalcitol, a dehydroxylated analog at the 1α-position of 1α,25-dihydroxy-2β-(3-hydroxy- propoxy)vitamin D3 (eldecalcitol), using Trost’s coupling methodology is described.It is well-established that vitamin D3 (cholecalciferol 1) ingested into foods or synthesized in the skin is metabolized to 25-hydroxyvitamin D3 (calcifediol 2) in the liver, which is further hydroxylated at the 1α-position in the kidney to produce the active form, 1α,25-dihydroxyvitamin D3 (calcitriol 3).2 Calcitriol (3) is well recognized as a potent regulator of calcium and phosphorous metabolism while also possessing regulatory effects on cell proliferation and differentiation processes.3 In Japan, calcitriol (3) and its synthetic prodrug, 1α-hydroxyvitamin D3 (alfacalcidol 4), which is also activated to 3 in the body (liver and bone), have been widely used for the treatment of osteoporosis for more than a quarter-century.4 Calcitriol (3) and alfacalcidol (4) have been recognized as very safe medicines that show mild or moderate increase in bone mineral density (BMD) in osteoporotic patients. There exists intense interest in obtaining active vitamin D3 analogs more potent than 3 and 4 in increasing BMD and preventing bone fracture with less calcemic activity for treating osteoporosis. 1α,25-Dihydroxy-2β-(3-hydroxypropoxy)vitamin D3 (eldecalcitol 5, developing code; ED-71), which possesses a hydroxypropoxy substituent at the 2β-position of the A-ring of 3, is such an analog that shows potent effects on bone therapy.5-7 Recent completion of a phase III clinical trial of 5 for bone fracture prevention and increase in BMD in comparison with alfacalcidol (4) produced excellent results.8,9 Eldecalcitol (5) is now ready for marketing as an excellent medicine for the treatment of osteoporosis in Japan (Scheme 1).
Figure 1 illustrates the basic relationship between serum calcium level (calcemic activity) and the targeted effect on bone (BMD increase) with cholecalciferol (1), calcitriol (3)/alfacalcidol (4), and eldecalcitol (5).10 The potent effect on bone is highest with 5 followed by 3 and 4 and then 1, at doses that induce approximately the same level of serum calcium (Figure 1). The question is what the different mode-of-action between calcitriol (3)/alfacalcidol (4) and eldecalcitol (5) is. Although specific physiological properties and mechanism of action of 5 are constantly being investigated, the long duration of 5 in the blood stream arises from its strong affinity for vitamin D binding protein (DBP) and might explain, in part, the enhanced activity of 5 over 3 and 4 toward bone.11,12 Interestingly, an idea was presented recently that calcitriol (3) is responsible for calcemic activity whereas the strong binding of calcifediol (2) to DBP and the long existence of 2 in blood are responsible for an anabolic effect on bone such as BMD increase.13 Since the structural relationship between 3 and 2 corresponds to that between eldecalcitol (5) and 25-hydroxy-2β-(3-hydroxypropoxy)vitamin D3 (1-deoxyeldecalcitol 6), we have been very interested in the biological actions of 6, e.g. possible hydroxylation of 6 to 5 in the kidney, affinity for DBP, duration in blood stream, and anabolic effect on bone (Scheme 1). Therefore, in this paper we describe the synthesis of 1-deoxyeldecalcitol (6) to explore structure-biological activity relationships between medicinally important eldecalcitol (5) and related analogs including 6.11,14-18
The synthesis of 1-deoxyeldecalcitol (6) was envisioned using Trost’s methodology involving palladium-catalyzed coupling between A-ring fragment 15 and C/D-ring fragment 16 (Scheme 2).19,20 The synthesis of A-ring fragment 15 started from epimeric alcohol 821 which was developed in our previous synthesis of eldecalcitol analogs from C2-symmetrical epoxide 7.22 Thus, alcohol 8 was acetylated to acetate 9, which was treated with tetrakis(triphenylphosphine)palladium (0) [(PPh3)4Pd] and ammonium formate (HCO2NH4) to give deacetoxy product 10 in 91% yield from 8.23 Diol 11 was obtained in 94% yield after deprotection of the acetonide moiety in 10 using 60% acetic acid. Mitsunobu reaction24 of 11 with diethyl azodicarboxylate (DEAD) and triphenylphosphine (PPh3) in 1,4-dioxane afforded epoxide 12 in 71% yield as a sole product. Reaction of 12 with lithium trimethylsilylacetylide in the presence of boron trifluoride diethyl etherate (BF3-OEt2) at -78 °C gave the enyne 13 in 86% yield, which was then converted to A-ring fragment 15 by saponification with 3 M sodium hydroxide (NaOH) and subsequent protection of the hydroxyl groups as their triethylsilyl (TES) ether in 93% overall yield.
With A-ring fragment 15 in hands, we next coupled it to C/D-ring fragment 16 using Trost’s methodology.19,20 Thus, A-ring fragment 15 was coupled with C/D-ring fragment 16, which was readily secured by our method25 from commercially available calcifediol (2), in the presence of (PPh3)4Pd and triethylamine (Et3N) in boiling toluene to produce the desired coupling product 17, although the coupling reaction conditions were not optimized. Desilylation of 17 with 47% hydrofluoric acid (HF) in acetonitrile (MeCN) at room temperature gave rise to 1-deoxyeldecalcitol (6) in 10% yield from 15 (Scheme 2).
Based on the Trost’s coupling methodology involving A-ring fragment 15 and C/D-ring fragment 16, we completed the synthesis of 1-deoxyeldecalcitol (6). The detailed biological actions of 6 in comparison with eldecalcitol (5) and related analogs will be investigated and reported in due course.
EXPERIMENTAL
Anhydrous THF was purchased from Kanto Chemical Co., Inc. MeOH and EtOH were distilled from sodium, toluene was distilled from phosphorus pentoxide, and CH2Cl2, Et3N, DMF, and pyridine were distilled from calcium hydride. All other purchased solvents and reagents were used without further purification. All reactions were carried out under an atmosphere of argon unless otherwise noted. Celite 545 was purchased from Nacarai Tesque Inc. All extracts were dried over magnesium sulfate and evaporated under reduced pressure with a rotary evaporator. Chromatographic purification was carried out with Silica Gel 60N Cat. No. 37560-84 purchased from Kanto Chemical Co., Inc., and flash column chromatography with Silica Gel 60N Cat. No. 37563-84 from Kanto Chemical Co., Inc. Optical rotations were measured with JASCO DIP-370 polarimeter. 1H and 13C NMR spectra were recorded on VARIAN Gemini-300 and Gemini-400 spectrometers using CDCl3 as a solvent. Chemical shifts are reported in parts per million (ppm) calibrated from CHCl3 (7.26 ppm) or tetramethylsilane (0.00 ppm) for 1H NMR and CDCl3 (77.1 ppm) for 13C NMR. Infrared (FTIR) spectra were obtained using JASCO FT/IR-5300, JEOL JIR-6000, and Hitachi 270-30 spectrophotometers. Mass spectra (MS) were measured with JEOL JMS-DX303 instrument. High resolution mass spectra (HRMS) were recorded on JEOL JMS-AX-500 and VG Auto Spec Q instruments.
3-((S)-1-((R)-2,2-Dimethyl-1,3-dioxolan-4-yl)but-3-enyloxy)propyl Pivalate (10): To a stirred solution of 8 (450 mg, 1.36 mmol), pyridine (0.26 mL, 3.26 mmol), and DMAP (1.6 mg, 0.013 mmol) in CH2Cl2 (4 mL) at rt, was added Ac2O (0.19 mL, 1.96 mmol). The resulting mixture was stirred at rt, diluted with Et2O, washed with 1 M HCl, saturated NaHCO3, and saturated NaCl, evaporated, and chromatographed on silica gel short column. Elution with n-hexane-AcOEt (12:1) gave 9 (510 mg, 100%), which was used for the next reaction without further purification. 1H NMR (CDCl3): δ 1.20 (9H, s), 1.33 (3H, s), 1.42 (3H, s), 1.88 (2H, quint, J = 6.1 Hz), 2.09 (1.5H, s), 2.11 (1.5H, s), 3.52-4.16 (8H, m), 5.24-5.34 (2.5H, m), 5.54 (0.5H, d, J = 5.6 Hz), 5.80-5.89 (1H, m). To a stirred and degassed solution of 9 (0.3 g, 0.81 mmol) and Et3N (0.6 mL, 4.3 mmol) in THF (8 mL), were added HCO2NH4 (0.5 g, 7.93 mmol) and (PPh3)4Pd (2.7 mg, 0.023 mmol). The resulting mixture was refluxed for 2 h. To the mixture, was added additional (PPh3)4Pd (2.7 mg, 0.023 mmol). The resulting mixture was refluxed for 40 min, diluted with AcOEt, washed with saturated NaCl, evaporated, and chromatographed on silica gel. Elution with n-hexane-AcOEt (9:1) gave 10 (227 mg, 91%) as a colorless oil. [α]D27 +20.8° (c 1.00, CHCl3). FTIR (neat): ν 1729, 1472, 1374, 1281, 1216, 1158, 1071 cm-1. 1H NMR (CDCl3): δ 1.25 (9H, s), 1.41 (3H, s), 1.43 (3H, s), 1.87 (2H, quint, J = 6.4 Hz), 2.25-2.37 (2H, m), 3.38-3.40 (1H, m), 3.57-3.68 (2H, m), 3.87 (1H, m), 4.03 (2H, q, J = 4.6 Hz), 4.15 (2H, t, J = 6.4 Hz), 5.06-5.14 (2H, m), 5.84 (1H, dt, J = 7.1, 10.0 Hz). 13C NMR (CDCl3): δ 25.3, 26.6, 27.1, 29.3, 35.5, 38.7, 61.2, 66.2, 66.8, 76.7, 79.6, 109.0, 117.4, 134.1, 178.4. EIMS m/z 314 (M+), 143 (100%). HRMS (EI) calcd for C17H36O5 (M+) 314.2093, found 314.2080.
3-((2R,3S)-1,2-Dihydroxyhex-5-en-3-yloxy)propyl Pivalate (11): A solution of 10 (380 mg, 1.21 mmol) in 60% AcOH (15 mL) was stirred at rt for 8 h, basified with saturated NaHCO3, extracted with CH2Cl2, washed with saturated NaCl, evaporated, and chromatographed on silica gel. Elution with n-hexane-AcOEt (3:2 then 1:1) gave 11 (311 mg, 94%) as a colorless oil. [α]D28 +19.1° (c 1.00, CHCl3). FTIR (neat): ν 3437, 1725, 1287, 1166, 1107 cm-1. 1H NMR (CDCl3): δ 1.20 (9H, s), 1.88 (2H, quint, J = 6.1 Hz), 2.30-2.40 (3H, m), 2.79 (1H, m), 3.46-3.50 (2H, m), 3.66-3.78 (4H, m), 4.10 (1H, quint, J = 6.1 Hz), 4.27 (1H, quint, J = 6.1 Hz), 5.08-5.16 (2H, m), 5.78-5.88 (1H, m). 13C NMR (CDCl3): δ 27.1, 29.3, 34.7, 61.1, 63.2, 66.4, 72.3, 80.5, 117.4, 134.2, 178.7. EIMS m/z 274 (M+), 143 (100%). HRMS (EI) calcd for C14H26O5 (M+) 274.1780, found 274.176
3-((S)-1-((R)-Oxiran-2-yl)but-3-enyloxy)propyl Pivalate (12): To a stirred solution of 11 (311 mg, 1.13 mmol) in 1,4-dioxane (11 mL) at rt, were added PPh3 (0.86 g, 3.4 mmol) and DEAD (40% in toluene, 1.56 mL, 5.16 mmol). The resulting mixture was refluxed for 24 h, cooled to rt, evaporated, and chromatographed on silica gel. Elution with n-hexane-AcOEt (10:1) gave 12 (206 mg, 71%) as a colorless oil. [α]D26 +7.61° (c 1.00, CHCl3). IR (neat): ν 1724, 1476, 1284, 1161 cm-1. 1H NMR (CDCl3): δ 1.20 (9H, s), 1.86 (2H, quint, J = 6.3 Hz), 2.30-2.44 (2H, m), 2.73-2.80 (2H, m), 2.94-2.96 (1H, m), 3.18-3.23 (1H, m), 3.46-3.52 (1H, m), 3.64-3.68 (1H, m), 4.14 (2H, t, J = 6.3 Hz), 5.07-5.16 (2H, m), 5.82-5.92 (1H, dt, J = 7.1, 10.3 Hz). 13C NMR (CDCl3): δ 27.1, 29.3, 37.0, 38.7, 45.3, 53.0, 61.2, 66.6, 78.4, 117.3, 133.9, 178.4. EIMS m/z 256 (M+), 143 (100%). HRMS (EI) calcd for C14H24O4 (M+) 256.1675, found 256.1672.
3-((4S,5R)-5-Hydroxy-8-(trimethylsilyl)oct-1-en-7-yn-4-yloxy)propyl Pivalate (13): To a stirred solution of trimethylsilylacetylene (423 μl, 2.99 mmol) in THF (8 mL) at -78 °C, was added n-BuLi (2.66 M solution in n-hexane, 1.0 mL, 2.66 mmol) and stirring was continued at -78 °C for 4 h. To the stirred mixture at -78 °C, were added 12 (150 mg, 0.586 mmol) in THF (8 mL) and BF3-OEt2 (384 µL, 0.434 mmol). The resulting mixture was allowed to warm to rt, stirred at rt for 14 h, quenched with saturated NaHCO3 (1.0 mL), extracted with CH2Cl2, washed with saturated NaCl, evaporated, and chromatographed on silica gel. Elution with n-hexane-AcOEt (20:1) gave 13 (178 mg, 86%) as a colorless oil. [α]D28 +17.0° (c 1.00, CHCl3). FTIR (neat): ν 3500, 1728, 1477, 1402, 1286, 1252, 1162, 1106 cm-1. 1H NMR (CDCl3): δ 0.15 (9H, s), 1.19 (9H, s), 1.87 (2H, quint, J = 6.1 Hz), 2.32-2.35 (2H, m), 2.51 (2H, d, J = 6.1 Hz), 3.37 (1H, q, J = 5.6 Hz), 3.52-3.63 (2H, m), 3.76 (1H, q, J = 5.6 Hz), 4.09-4.20 (2H, m), 5.05-5.14 (2H, m), 5.80-5.89 (1H, m). 13C NMR (CDCl3): δ 0.00, 24.3, 27.2, 29.3, 34.1, 38.7, 61.2, 66.4, 70.4, 80.9, 87.5, 103.0, 117.2, 134.6, 178.5. EIMS m/z 354 (M+), 57 (100%). HRMS (EI) calcd for C19H34O4Si (M+) 354.2227, found 354.2224.
(4R,5S)-5-(3-Hydroxypropoxy)oct-7-en-1-yn-4-ol (14): To a stirred solution of 13 (77.5 mg, 0.219 mmol) in MeOH (2 mL) at 0 °C, was added 3 M NaOH (1 mL). The resulting mixture was stirred at rt for 8 h, neutralized with 1 M HCl (0.5 mL), evaporated, and chromatographed on silica gel. Elution with n-hexane-AcOEt (20:1) gave 14 (43.1 mg, 99%) as a colorless oil. [α]D28 +23.5° (c 1.00, CHCl3). FTIR (neat): ν 3296, 1641, 1423, 1004 cm-1. 1H NMR (CDCl3): δ 1.81 (2H, quint, J = 2.7 Hz), 2.06 (1H, s), 2.34-2.38 (2H, m), 2.51 (3H, m), 3.43-3.46 (1H, m), 3.73-3.80 (5H, m), 5.10-5.17 (2H, m), 5.83-5.90 (1H, m). 13C NMR (CDCl3): δ 22.9, 32.5, 34.6, 61.1, 69.1, 70.7, 70.9, 81.2, 81.4, 117.7, 134.7. EIMS m/z 198 (M+), 59 (100%). HRMS (EI) calcd for C11H18O3 (M+) 198.1256, found 198.1242.
(4S,5R)-4-(3-(Trimethylsilyloxy)propoxy)-5-(trimethylsilyloxy)oct-1-en-7-yne (15): To a stirred mixture of 14 (50 mg, 0.252 mmol) in CH2Cl2 (2 mL) at -40 °C, were added 2,6-lutidine (283 µL, 1.26 mmol) and TESOTf (240 µL, 1.26 mmol). The resulting mixture was stirred at the same temperature for 9 h, quenched with saturated NaHCO3 (2 mL), extracted with CH2Cl2, washed with saturated NaHCO3 and saturated NaCl, evaporated, and chromatographed on silica gel. Elution with n-hexane-CH2Cl2 (6:1) containing 2% Et3N gave 15 (100 mg, 93%) as a colorless oil. [α]D24 -3.7° (c 1.06, CHCl3). FTIR (neat): ν 1463, 1416, 1238, 1100, 1010 cm-1. 1H NMR (CDCl3): δ 0.60 (12H, q, J = 8.1 Hz), 0.93 (18H, t, J = 8.1 Hz), 1.77 (2H, quint, J = 6.4 Hz), 1.97 (1H, s), 2.27-2.47 (4H, m), 3.38 (1H, q, J = 5.1 Hz), 3.61 (2H, q, J = 6.3 Hz), 3.68 (2H, t, J = 6.3 Hz), 3.78 (1H, q, J = 5.1 Hz), 5.03-5.12 (2H, m), 5.82-5.93 (1H, m). 13C NMR (CDCl3): δ 4.4, 5.0, 6.7, 6.8, 23.8, 33.4, 34.8, 59.9, 67.4, 70.0, 72.2, 81.3, 81.6, 116.6, 135.3. EIMS m/z 426 (M+), 115 (100%). HRMS (EI) calcd for C23H46O3Si2 (M+) 426.2986, found 426.2997.
(1R,2S,5Z)-2-(3-Hydroxypropoxy)-5-((E)-2-((1R,3aS,7aR)-hexahydro-1-((R)-6-hydroxy-6-methylheptan-2-yl)-7a-methyl-1H-inden-4(7aH)-ylidene)ethylidene-4-methylenecyclohexanol (6): To a stirred and degassed solution of 15 (90 mg, 0.211 mmol), 16 (90 mg, 0.253 mmol), and Et3N (4.7 mL) in toluene (3.2 mL) at rt, was added (PPh3)4Pd (20 mg, 0.017 mmol). The resulting mixture was refluxed for 22 h, diluted with Et2O, and filtrated with Celite. The filtrate was diluted with AcOEt, washed with H2O and saturated NaCl, evaporated, and chromatographed on silica gel. Elution with n-hexane-AcOEt (20:1) containing 2% Et3N gave crude 17 (64 mg), which was used for the next reaction without further purification. To a stirred mixture of crude 17 (64 mg) in MeCN (16 mL) at 0 °C, was added 47% HF (100 µL). The resulting mixture was stirred at the same temperature for 10 min, neutralized with NaHCO3 (3 g), extracted with Et2O, washed with saturated NaCl, evaporated, and chromatographed on silica gel. Elution with AcOEt gave 6 (10.2 mg, 10%) as a white solid. [α]D20 +19.9° (c 0.32, CHCl3). FTIR (neat): ν 3387, 2942, 1710, 1374, 1081 cm-1. 1H NMR (CDCl3): δ 0.54 (3H, s), 0.93 (3H, d, J = 6.6 Hz), 1.03-1.06 (1H, m), 1.25 (3H, s), 1.27-1.35 (4H, m), 1.41-1.54 (10H, m), 1.65-1.67 (2H, m), 1.83-1.87 (2H, m), 1.95-2.05 (2H, m), 2.26 (1H, br s), 2.37 (2H, m), 2.49-2.57 (2H, m), 2.81 (1H, m), 3.58 (1H, quint, J = 4.2 Hz), 3.68-3.79 (5H, m), 4.01 (1H, br s), 4.92 (1H, s), 5.11 (1H, s), 6.02 (1H, d, J = 11.5 Hz), 6.26 (1H, d, J = 11.5 Hz). 13C NMR (CDCl3): δ 12.0, 18.8, 20.8, 22.3, 23.6, 27.7, 29.0, 29.2, 29.3, 32.2, 36.1, 36.4, 40.5, 41.0, 44.4, 45.8, 56.3, 56.5, 61.6, 67.2, 68.9, 71.1, 79.2, 114.7, 117.5, 122.9, 133.6, 141.6, 142.6. EIMS m/z 474 (M+), 134 (100%). HRMS (EI) calcd for C30H50O4 (M+) 474.3709, found 474.3710.
ACKNOWLEDGMENTS
We are grateful to Professor David Horne of Division of Molecular Medicine, City of Hope for reading of the manuscript and helpful suggestions.
References
1. This forms part 39 of ‘synthetic studies on vitamin D analogs’ by N. Kubodera at Chugai Pharmaceutical Co., Ltd. Part 38; S. Hatakeyama, M. Yoshino, K. Eto, K. Takahashi, J. Ishihara, Y. Ono, H. Saito, and N. Kubodera, J. Steroids Biochem. Mol. Biol., 2010, 121, 25. CrossRef
2. R. L. Horst, T. A. Reinhardt, and G. S. Reddy, ‘Vitamin D metabolism’ Vitamin D Second Edition, ed. by D. Feldman, J. W. Pike, and F. H. Glorieux, Elsevier Academic Press, Burlington, 2005, pp. 15-36. CrossRef
3. R. Bouillon, W. H. Okamura, and A. Norman, Endocrine Rev., 1995, 16, 200.
4. R. Eastell and B. L. Riggs, ‘Vitamin D and osteoporosis’ Vitamin D Second Edition, ed. by D. Feldman, J. W. Pike, and F. H. Glorieux, Elsevier Academic Press, Burlington, 2005, pp. 1101-1120. CrossRef
5. K. Miyamoto, E. Murayama, K. Ochi, H. Watanabe, and N. Kubodera, Chem. Pharm. Bull., 1993, 41, 1111.
6. Y. Ono, H. Watanabe, A. Shiraishi, S. Takeda, Y. Higuchi, K. Sato, N. Tsugawa, T. Okano, T. Kobayashi, and N. Kubodera, Chem. Pharm. Bull., 1997, 45, 1626.
7. Y. Ono, A. Kawase, H. Watanabe, A. Shiraishi, S. Takeda, Y. Higuchi, K. Sato, T. Yamauchi, T. Mikami, M. Kato, N. Tsugawa, T. Okano, and N. Kubodera, Bioorg. Med. Chem., 1998, 6, 2517. CrossRef
8. T. Matsumoto, M. Ito, Y. Hayashi, T. Hirota, Y. Tanigawara, T. Sone, M. Fukunaga, M. Shiraki, and T. Nakamura, in preparation for submission.
9. T. Matsumoto, T. Takano, S. Yamakido, F. Takahashi, and N. Tsuji, J. Steroids Biochem. Mol. Biol., 2010, 121, 261. CrossRef
10. N. Kubodera, Mini-Reviews Med. Chem., 2009, 9, 1416.
11. K. Eto, A. Fujiyama, M. Kaneko, K. Takahashi, J. Ishihara, S. Hatakeyama, Y. Ono, and N. Kubodera, Heterocycles, 2009, 77, 323. CrossRef
12. T. Okano, N. Tsugawa, S. Masuda, A. Takeuchi, T. Kobayashi, Y. Takita, and Y. Nishii, Biochem. Biophys. Res. Commun., 1989, 163, 1444. CrossRef
13. A. G. Need and B. E. C. Nordin, Bone, 2008, 42, 1021. CrossRef
14. S. Hatakeyama, S. Nagashima, N. Imai, K. Takahashi, J. Ishihara, A. Sugita, T. Nihei, H. Saito, F. Takahashi, and N. Kubodera, Bioorg. Med. Chem., 2006, 14, 8050. CrossRef
15. A. Fujiyama, M. Kaneko, K. Takahashi, J. Ishihara, S. Hatakeyama, and N. Kubodera, Heterocycles, 2007, 71, 2263. CrossRef
16. N. Kubodera and S. Hatakeyama, Anticancer Res., 2009, 29, 3571.
17. M. Yoshino, K. Eto, K. Takahashi, J. Ishihara, S. Hatakeyama, Y. Ono, H. Saito, and N. Kubodera, Heterocycles, 2010, 81, 381. CrossRef
18. S. Honzawa, Y. Yamamoto, A. Yamashita, T. Sugiura, M. Kurihara, M. A. Arai, S. Kato, and A. Kittaka, Bioorg. Med. Chem., 2008, 16, 3002. CrossRef
19. B. M. Trost and J. Dumas, J. Am. Chem. Soc., 1992, 114, 1924. CrossRef
20. B. M. Trost, J. Dumas, and M. Villa, J. Am. Chem. Soc., 1992, 114, 9836. CrossRef
21. S. Hatakeyama, A. Kawase, Y. Uchiyama, J. Maeyama, Y. Iwabuchi, and N. Kubodera, Steroids, 2001, 66, 267. CrossRef
22. K. C. Nicolaou, D. P. Papahatjis, D. A. Claremon, R. L. Magolda, and R. E. Dolle, J. Org. Chem., 1985, 50, 1440. CrossRef
23. J. Tsuji, I. Minami, and I. Shimizu, Synthesis, 1986, 623. CrossRef
24. O. Mitsunobu, Synthesis, 1981, 1. CrossRef
25. J. Maeyama, H. Hiyamizu, K. Takahashi, J. Ishihara, S. Hatakeyama, and N. Kubodera, Heterocycles, 2006, 70, 295. CrossRef