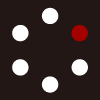
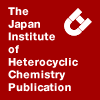
HETEROCYCLES
An International Journal for Reviews and Communications in Heterocyclic ChemistryWeb Edition ISSN: 1881-0942
Published online by The Japan Institute of Heterocyclic Chemistry
e-Journal
Full Text HTML
Received, 26th February, 2011, Accepted, 11th April, 2011, Published online, 12th April, 2011.
DOI: 10.3987/COM-11-12191
■ Synthesis and Properties of anti/syn-Regioisomeric Mixtures of Alkyl-Substituted Tetracenes
Chitoshi Kitamura,* Hiroyuki Kano, Hideki Tsukuda, Takeshi Kawase, Takashi Kobayashi, and Hiroyoshi Naito
Department of Materials Science and Chemistry, Graduate School of Engineering, University of Hyogo, 2167 Shosha, Himeji, Hyogo 671-2201, Japan
Abstract
We prepared anti/syn-regioisomeric mixtures of alkyl-substituted tetracenes via Diels-Alder reaction between asymmetric furans and 2,6-naphthodiyne synthon. The solid-state color of the mixtures changed before and after recrystallization from Et2O, suggesting a difference in the molecular arrangements dependent on the different alkyl substituents as well as the change in the distribution of the anti/syn regioisomers after recrystallization. Slow evaporation of the recrystallized mixtures produced single crystals suitable for X-ray analysis, which revealed that the anti regioisomer was isolated.INTRODUCTION
Preparation and application of organic semiconducting molecules are the subject of recent interest. Although pentacene is an important representative molecule that shows excellent hole mobilities,1 it suffers from instability under light and air and insolubility in common organic solvents.2 On the other hand, tetracene has a more attractive framework because it has the advantages of air stability in its solid state, solubility, and a low melting point though its mobility may be lower than that of pentacene. To develop tetracene-based semiconductors,3 researchers have prepared many functionalized tetracenes.4 In recent years, we have focused on alkyl-substituted tetracenes to obtain high solubilities, low melting points, and good film morphologies. Thus, we have synthesized a series of tetracenes (1,4,7,10-tetraalkyl, 1,4-dialkyl, 2,3-dialkyl, and 2,3,8,9-tetraalkyl derivatives).5 We revealed that these compounds were not only typical semiconductors but also interesting chromophores because of the unique dependences of the solid-state color on their lengths, shapes, numbers, and substitution positions of the alkyl side chains, despite being independent of their optical properties in solution. We found that the alkyl side chains can serve as an adjuster of the molecular arrangements to control the solid-state optical properties. Therefore, introducing a new substitution pattern may allow the development of new optical functionalities. So far, we have investigated single-component alkyl-substituted tetracenes in which all the alkyl groups are the same; however, we did not consider bimolecular mixtures composed of anti 1 and syn 2 regioisomers, in which two different types of groups were located at the 1,4,7,10-positions (Figure 1). In this study, we report the synthesis and characterization of new alkyl-substituted tetracene mixtures, 1,7- and 1,10-dihexyltetracene (1a and 2a, respectively) and 1,7-dihexyl-4,10-diisopropyl- and 1,10-dihexyl-4,7-diisopropyltetracene (1b and 2b, respectively).
RESULTS AND DISCUSSION
We prepared anti/syn mixtures of 1a/2a and 1b/2b via our recently reported procedure,5b by using asymmetric furans (3a and 3b) and 2,6-naphthodiyne precursor 4, as shown in Scheme 1. 2-Hexylfuran (3a) was prepared from a lithiation-nucleophilic substitution sequence. By using the same synthetic procedure employed for 2,5-diisopropylfuran,5d we synthesized 2-hexyl-5-isopropylfuran (3b) via the
reaction of lithiated 3a and acetone followed by hydrogenolysis. Slow generation of 2,6-naphthodiyne synthon by treating 4 with excess asymmetric furans (3a and 3b) in the presence of KF and 18-crown-6 in THF afforded Diels–Alder cycloadducts, which were hydrogenated, dehydrated with conc. HCl and Ac2O, and purified via column chromatography to give tetracene anti/syn-regioisomeric mixtures of 1a/2a and 1b/2b, respectively. The tetracene mixtures were soluble in common organic solvents such as hexane and Et2O. We could not confirm the anti/syn ratio because there were no differences in the 1H and 13C NMR signals as well as the Rf values of the anti 1 and syn 2 regioisomers.
Interestingly, after preparation, 1a/2a and 1b/2b were immediately isolated as an orange powder and a yellow viscous solid, respectively (Figure 2). Furthermore, recrystallization from Et2O produced a visible change in the solid-state colors, and thus 1a/2a and 1b/2b were obtained as yellow and orange powders, respectively. Alkyl side chains do not absorb visible light and have little electronic effect on the tetracene ring. Therefore, the differences in the solid-state colors before and after recrystallization were most likely due to crystallochromy,6,7 i.e., a color change caused by different intermolecular interactions based on different aggregation structures. In the step before recrystallization, we can explain the difference in color as the different molecular arrangements of tetracene rings dependent on the different substitution patterns of 1a/2a and 1b/2b. On the other hand, in the step after recrystallization, the result probably showed an unbalanced distribution of anti/syn regioisomers in which the more crystalline component would dominate the inherent molecular arrangements compared with the other component. This was supported by the difference in the melting points before and after recrystallization: the melting points of 1a/2a and 1b/2b before recrystallization were 75–77 °C and 55–57 °C, respectively; in contrast, those of 1a/2a and 1b/2b after recrystallization were 121–122 °C and 148–150 °C, respectively.
We spectroscopically observed the change in the solid-state optical properties before and after recrystallization with Et2O via absorption (Kubelka-Munk) and fluorescence spectra (Figure 3). The spectral profiles of 1a/2a and 1b/2b before and after recrystallization showed significant differences. In the absorption spectra, 1a/2a and 1b/2b before and after recrystallization showed structured bands. The longest absorption maximum of 1a/2a before recrystallization was 498 nm, whereas that of 1a/2a after recrystallization was 483 nm (Figure 3a). In contrast, the absorption spectrum of 1b/2b before recrystallization was characterized by one broad band around 500 nm, whereas that of 1b/2b after recrystallization showed the longest wavelength absorption band at 489 nm with a shoulder around 520 nm (Figure 3b). The change in the absorption edges before and after recrystallization also correlated well with that of the solid-state colors. Thus, the absorption edges of 1a/2a before and after recrystallization were 545 nm and 530 nm, respectively (Figure 3a), and those of 1b/2b before and after recrystallization were 540 nm and 551 nm, respectively (Figure 3b). We also noted the differences in the fluorescence properties before and after recrystallization. The fluorescence quantum yields of 1b/2b before and after recrystallization (0.31 and 0.27, respectively) were larger than those of 1a/2a before and after recrystallization (0.04 and 0.08, respectively). We also observed a great difference in the spectral shapes between 1a/2a and 1b/2b. The spectrum of 1a/2a before recrystallization exhibited two peaks (551 and 593 nm) and a shoulder (528 nm), and that of 1a/2a after recrystallization displayed three peaks (529, 563, and 602 nm). In contrast, the spectrum of 1b/2b after recrystallization illustrated one strong band at 560 nm, whereas that of 1b/2b before recrystallization displayed two bands at 559 and 569 nm. All the above-mentioned results would be derived from different molecular arrangements of the tetracene moieties.
When we performed slow evaporation of the recrystallized samples from Et2O solutions, we succeeded in obtaining some single crystals suitable for X-ray analysis. Then, we determined their crystal structures (Figure 4).8 The molecules proved to be anti regioisomers 1a and 1b (Figure 4a). Although we attempted recrystallization from the filtrate components under various conditions, we could not obtain any single crystals of the syn regioisomers. In both 1a and 1b, the tetracene rings were essentially planar. Their hexyl groups, except for the terminal methyl groups in 1a, adopted zigzag conformations, and the zigzag planes were almost coplanar with the tetracene ring. The hexyl conformations in 1a and 1b were
completely different from those in 1,4,7,10-tetrahexyltetracene,5b which were perpendicular to the tetracene ring. In both 1a and 1b, there was no π-overlap between the two neighboring molecules along the stacking direction (Figure 4b). The mutual orientations were significantly different in 1a and 1b. The interplanar distances between the tetracene planes were 3.56 Å for 1a and 3.79 Å for 1b. The difference in the slip distance along the long molecular axis was almost 1 Å (7.19 Å for 1a and 8.20 Å for 1b), and the difference in the slip distance along the short molecular axis was above 2 Å (3.17 Å for 1a and 5.33 Å for 1b). In the packing structures, the molecules crystallized in layered structures while 1a and 1b appear to be stacked in a staircase-type fashion and a brickwork-type fashion, respectively (Figure 4d). Within the layer, one molecule of 1a is surrounded by six neighboring molecules, and one molecule of 1b is environed by eight neighboring molecules (Figure 4c). In the crystal of 1a, the hexyl groups were arranged in a head-to-tail regularity, and we observed alternating stripes composed of alkyl and tetracene regions. These arrangements were slightly different from those in the crystal of 1b; the existence of isopropyl groups broadened the intermolecular spacing between neighboring molecules. The differences in these molecular arrangements would probably cause the differences in the solid-state optical properties.
In conclusion, we prepared anti/syn-regioisomeric mixtures of 1a/2a and 1b/2b to examine the effects of the alkyl substitution and the mixed molecules on the solid-state color. Interestingly, the color of 1a/2a changed from orange to yellow by recrystallization from Et2O, whereas that of 1b/2b changed from yellow to orange. The difference in the solid-state color was probably due to the different intermolecular interactions based on the different aggregation structures as well as the unbalanced distribution of the anti/syn regioisomers after recrystallization from Et2O. X-ray analysis of single crystals prepared by slow evaporation from Et2O revealed that anti 1 was isolated as single crystals.
EXPERIMENTAL
All reagents were commercially available and used without further purification. 2,6-Naphthodiyne precursor 4 was prepared according to the literature method.5b All reactions were performed under nitrogen atmosphere. Column chromatography was performed on a Wako C-300 silica-gel column (45–75 µm). Melting points were measured on a Yanaco melting point apparatus. 1H and 13C spectra were measured with a Bruker-Biospin DRX500 FT spectrometer. EI-MS spectra were measured on a Shimadzu GCMS-QP5050A. Elemental analyses were carried out on a Yanaco MT-5 CHN corder. Absorption and fluorescence spectra in solution were recorded with a HITACHI U3500 spectrophotometer and a HITACHI F2500 spectrophotometer (λex = 365 nm), respectively. Kubelka-Munk spectra were measured using a HITACHI U3010 spectrophotometer equipped with a Φ60 integrating sphere attachment. Fluorescence spectra in the solid state were measured on a Hamamatsu Photonics PMA11 calibrated optical multichannel analyzer with a solid-state blue laser (λex = 377 nm). Absolute quantum yields were determined with a Labsphere IS-040-SF integrating sphere.
Preparation of 2-hexylfuran (3a)
To an ice-cooled mixture of furan (2.0 mL, 27.6 mmol) and TMEDA (10 mL), 1.6 M n-BuLi in hexane (20 mL, 32.0 mmol) was added slowly. The mixture was refluxed for 1 h to accomplish mono-lithiation. The mixture, which changed to a brown suspension, was allowed to cool to rt and was then cooled with an ice bath. To the ice-cooled mixture, a solution of 1-bromohexane (4.8 mL, 34.4 mmol) in THF (15 mL) was added dropwise. The mixture was stirred at rt for 16 h. After quenching with water, the crude product was extracted with Et2O, washed with brine, and dried over Na2SO4. After evaporation of the solvents, the residue was subjected to column chromatography (hexane) on silica gel to remove polar components. Distillation under reduced pressure (22 mmHg, > 90 °C) provided 3a a colorless oil (1.82 g, 43%). 1H NMR (500 MHz, CDCl3) δ 0.88 (t, J = 6.8 Hz, 3H), 1.26–1.37 (m, 6H), 1.59–1.65 (m, 2H), 2.60 (t, J = 7.7 Hz, 2H), 5.95 (d, J = 3.0 Hz, 1H), 6.26 (dd, J = 1.8, 3.0 Hz, 1H), 7.27 (d, J = 1.8 Hz, 1H); 13C NMR (126 MHz, CDCl3) δ 13.96, 22.59, 27.97, 28.04, 28.90, 31.62, 104.46, 109.90, 140.50, 156.45.
Preparation of 2-hexyl-5-isopropylfuran (3b)
To an ice-cooled mixture of furan 3a (3.00 g, 19.7 mmol) and TMEDA (6.3 mL), 1.6 M n-BuLi in hexane (18 mL, 28.8 mmol) was added slowly. The mixture was refluxed for 1 h. The mixture was allowed to cool to rt and was then cooled with an ice bath. To the ice-cooled mixture, a solution of acetone (5.0 mL, 68.0 mmol) in THF (7.5 mL) was added dropwise. Then the mixture was stirred at rt for 16 h. After quenching with water, the crude alcohol was extracted with AcOEt, washed with brine, and dried over Na2SO4. After evaporation of the solvents, the residue was roughly purified by column chromatography (CHCl3) on silica gel to give a brown oil (1.88 g). Because of its difficult purification, the product containing some impurities was used in the next reaction. A solution of the brown oil (1.88 g) in CH2Cl2 (50 mL) was hydrogenated over 10% Pd/C (100 mg) under atmospheric pressure at rt for 6 h. The catalyst was removed by filtration and the filtrate was concentrated under reduced pressure. Column chromatography (hexane) on silica gel afforded 3b as a yellow oil (1.29 g, 34% from 3a). 1H NMR (500 MHz, CDCl3) δ 0.88 (t, J = 7.0 Hz, 3H), 1.22 (d, J = 6.9 Hz, 6H), 1.28–1.39 (m, 6H), 1.59–1.63 (m, 2H), 2.56 (t, J = 7.6 Hz, 2H), 2.85–2.90 (m, 1H), 5.81–5.84 (m, 2H); 13C NMR (126 MHz, CDCl3) δ 14.07, 21.20, 22.59, 27.79 (2C), 28.08, 28.92, 31.61, 102.74, 104.57, 154.65, 159.91.
Preparation of an anti/syn mixture of 1,7- and 1,10-dihexyltetracene (1a/2a)
KF (1.12 g, 19.3 mmol) was added to a solution of 2,6-naphthodiyne precursor 4 (2.60 g, 4.57 mmol), furan 3a (1.54 g, 10.1 mmol), and 18-crown-6 (4.80 g, 18.2 mmol) in THF (80 mL). The mixture was stirred at room temperature for 18 h. Water was added, and the resulting mixture was extracted with CHCl3. The combined organic layer was washed with brine and dried over Na2SO4. After removal of the solvents, the residue was roughly purified by column chromatography (CHCl3/hexane = 1/2) to afford a bis(furan) adduct (162 mg) as a yellow oil. Because of its difficult purification, the adduct was then used in the next reaction. A solution of the adduct (162 mg) in EtOH (30 mL) was hydrogenated over 10% Pd/C (33 mg) under atmospheric pressure at rt for 4 h. The catalyst was removed by filtration, and the filtrate was concentrated under reduced pressure. To the residue was added a cold solution of conc. HCl (1 mL) and Ac2O (5 mL) at 0 °C, and the mixture was stirred at rt for 40 min. Water was added, and the resulting mixture was extracted with CHCl3. The combined organic layer was washed with brine and dried over Na2SO4. After removal of the solvents, purification by column chromatography (hexane) on silica gel afforded tetracene 1a/2a as an orange solid (94 mg, 5% from 4). mp 75–77 °C. Recrystallization of the orange solid from Et2O produced a yellow solid (27 mg, 1.5% from 4). mp 121–122 °C. 1H NMR (500 MHz, CDCl3) δ 0.92 (t, J = 7.1 Hz, 6H), 1.36–1.40 (m, 8H), 1.49–1.55 (m, 4H), 1.88–1.91 (m, 4H), 3.22 (t, J = 7.8 Hz, 4H), 7.21 (d, J = 6.5 Hz, 2H), 7.32 (dd, J = 6.5, 8.5 Hz, 2H), 7.88 (d, J = 8.5 Hz, 2H), 8.71 (s, 2H), 8.81 (s, 2H); 13C NMR (126 MHz, CDCl3) δ 14.16, 22.73, 29.65, 30.23, 31.83, 33.37, 122.34, 124.24, 124.85, 126.86, 127.39, 129.76, 130.58, 132.06, 138.74; MS m/z 396 (M+, 100); UV-vis (hexane) λmax (log ε) = 420 (3.56), 446 (3.71), 476 nm (3.71); Fluorescence (hexane) λem = 486, 514, 551 nm (Φ = 0.18). Anal. Calcd for C30H36: C, 90.85; H, 9.15. Found: C, 90.70; H, 9.19.
Preparation of an anti/syn mixture of 1,7-dihexyl-4,10-diisopropyl- and 1,10-dihexyl-4,7- diisopropyltetracene (1b/2b)
The title compound was prepared following the same procedure as described above for 1a/2a except that reagents KF (927 mg, 16.0 mmol), 2,6-naphthodiyne precursor 4 (2.20 g, 3.80 mmol), furan 5b (1.70 g, 9.10 mmol), 18-crown-6 (3.71 g, 13.9 mmol), THF (100 mL), 10% Pd/C (200 mg), and EtOH (50 mL) were used. The title compound was obtained as a yellow viscous solid (400 mg, 22% from 4). mp 55–57 °C. Recrystallization of the yellow solid from Et2O produced an orange solid (350 mg, 19% from 4). mp 148–150 °C. 1H NMR (500 MHz, CDCl3) δ 0.93 (t, J = 6.9 Hz, 6H), 1.32–1.59 (m, 24H), 1.88–1.93 (m, 4H), 3.21 (t, J = 7.8 Hz, 4H), 3.94–4.00 (m, 2H), 7.23–7.26 (m, 4H), 8.86 (s, 2H), 8.94 (s, 2H); 13C NMR (126 MHz, CDCl3) δ 14.20, 22.76, 23.63, 28.68, 29.73, 30.18, 31.88, 33.35, 120.16, 122.71, 123.40, 123.98, 128.93, 130.44, 131.09, 136.57, 142.53; MS m/z 480 (M+, 100); UV-vis (hexane) λmax (log ε) = 424 (3.69), 451 (3.94), 481 nm (3.93); Fluorescence (hexane) λem = 494, 522, 558 nm (Φ = 0.11). Anal. Calcd for C36H48: C, 89.94; H, 10.06. Found: C, 89.88; H, 10.24.
X-Ray crystallographic analysis
Crystal data for 1a: C30H36, Fw = 396.59, T = 223 K, triclinic, space group P−1, a = 7.648(4), b = 8.428(4), c = 10.406(5) Å, α = 67.430(17), β = 74.293(19), γ = 79.68(2)°, V = 594.1(5) Å3, Z = 1, Dcalcd = 1.108 g cm−3, 4735 reflections measured, 2680 independent, 146 parameters refined, GOF = 1.091, R1 = 0.087 [I > 2σ(I)], wR2 = 0.303 (all data). Crystal data for 1b: C36H48, Fw = 480.74, T = 223 K, monoclinic, space group C2, a = 19.570(3), b = 7.5746(11), c = 9.8068(14) Å, β = 99.493(3)°, V = 1433.8(3) Å3, Z = 2, Dcalcd = 1.114 g cm−3, 7041 reflections measured, 3222 independent, 175 parameters refined, GOF = 0.876, R1 = 0.087 [I > 2σ(I)], wR2 = 0.369 (all data). Single crystals were grown from Et2O solutions by slow evaporation. X-Ray diffraction data were collected on a Rigaku Mercury diffractometer for 1a and a Rigaku RAXIS RAPID diffractometer for 1b with a graphite−monochromated Mo-Kα (λ = 0.7107 Å) radiation. The structures were solved by direct methods using SIR2004.9 All non-hydrogen atoms were refined anisotropically by full-matrix least-squares on F2 using SHELXL97.10 All the H atoms were geometrically positioned and refined using a riding model. All calculations were performed using the WinGX program package.11 Crystallographic data for the structures in this paper have been deposited with the Cambridge Crystallographic Data Centre as CCDC 805752 (1a) and 805751 (1b).
ACKNOWLEDGEMENTS
This study was supported by the Grant-in-Aid from JSPS and by the University of Hyogo. We thank the Instrument Center of the Institute for Molecular Science for the X-ray structural analysis and Chisso Petrochemical Corporation for the gift of reagents.
References
1. M. Bendikov, F. Wudl, and D. F. Perepichka, Chem. Rev., 2004, 104, 4891. CrossRef
2. M. D. Curtis, J. Cao, and J. W. Kampf, J. Am. Chem. Soc., 2004, 126, 4318. CrossRef
3. J. E. Anthony, Angew. Chem. Int. Ed., 2008, 47, 452. CrossRef
4. For selected examples, see: a) C.-L. Lin, K.-H. Lin, B. Pal, and L.-D. Tsou, Chem. Commun., 2009, 803; CrossRef b) T. Kimoto, K. Tanaka, Y. Sakai, A. Ohno, K. Yoza, and K. Kobayashi, Org. Lett., 2009, 11, 3658; CrossRef c) T. Seri, H. Qu, L. Zhou, K. Kanno, and T. Takahashi, Chem. Asian J., 2008, 3, 388; CrossRef d) A. S. Paraskar, A. R. Reddy, A. Patra, Y. H. Wijsboom, O. Gidron, L. J. W. Shimon, G. Leitus, and M. Bendikov, Chem. Eur. J., 2008, 14, 10639; CrossRef e) Z. Chen, P. Müller, and T. M. Swager, Org. Lett., 2006, 8, 273; CrossRef f) R. Schmidt, S. Göttling, D. Leusser, D. Stalke, A.-M. Krause, and F. Würthner, J. Mater. Chem., 2006, 16, 3708; CrossRef g) J. Reichwagen, H. Hopf, A. Del Guerzo, J.-P. Desvergne, and H. Bouas-Laurent, Org. Lett., 2005, 7, 971; CrossRef h) J. A. Merio, C. R. Newman, C. P. Gerlach, T. W. Kelley, D. V. Muyres, S. E. Fritz, M. F. Toney, and C. D. Frisbie, J. Am. Chem. Soc., 2005, 127, 3997. CrossRef
5. a) C. Kitamura, T. Ohara, N. Kawatsuki, A. Yoneda, T. Kobayashi, H. Naito, T. Komatsu, and T. Kitamura, CrystEngComm, 2007, 9, 644; CrossRef b) C. Kitamura, Y. Abe, T. Ohara, A. Yoneda, T. Kawase, T. Kobayashi, H. Naito, and T. Komatsu, Chem. Eur. J., 2010, 16, 880; c) C. Kitamura, C. Matsumoto, A. Yoneda, T. Kobayashi, H. Naito, and T. Komatsu, Eur. J. Org. Chem., 2010, 2571; CrossRef d) C. Kitamura, H. Tsukuda, A. Yoneda, T. Kawase, T. Kobayashi, and H. Naito, Eur. J. Org. Chem., 2010, 3033; CrossRef e) C. Kitamura, T. Ohara, A. Yoneda, T. Kawase, T. Kobayashi, and H. Naito, Chem. Lett., 2011, 40, 58. CrossRef
6. G. Klebe, F. Graser, E. Hädicke, and J. Berndt, Acta Crystallogr., 1989, B45, 2511.
7. There were no remarkable differences in the optical properties in solution between 1a/2a and 1b/2b as well as before and after recrystallization.
8. We observed that each molecule had two disordered sets of alkyl groups. For clarity, minor sites are omitted in Figure 4.
9. M. C. Burla, R. Caliandro, M. Camalli, B. Carrozzini, G. L. Cascarano, L. De Caro, C. Giacovazzo, G. Polidori, and R. Spagna, J. Appl. Cryst., 2005, 38, 381. CrossRef
10. G. M. Sheldrick, Acta Crystallogr., 2008, A64, 112.
11. L. J. Farrugia, J. Appl. Cryst., 1999, 32, 837. CrossRef