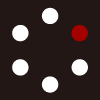
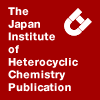
HETEROCYCLES
An International Journal for Reviews and Communications in Heterocyclic ChemistryWeb Edition ISSN: 1881-0942
Published online by The Japan Institute of Heterocyclic Chemistry
e-Journal
Full Text HTML
Received, 29th June, 2011, Accepted, 25th July, 2011, Published online, 2nd August, 2011.
DOI: 10.3987/COM-11-S(P)60
■ Facile Synthesis of 4,9-Dihydro-2H-benz[f]- and 4,11-Dihydro-2H-naphth[2,3-f]isoindoles and Their Utility for Porphyrin Synthesis
Cai Chenxin, Hiroki Uoyama, Mitsunori Nakamura, and Hidemitsu Uno*
Department of Chemistry and Biology, Graduate School or Science and Engineering, Ehime University, Bunkyo-cho 2-5, Matsuyama 790-8577, Japan
Abstract
Esters of 4,9-dihydro-2H-benz[2,3-f]isoindole-1-carboxylate and 4,11-dihydro-2H-naphth[2,3-f]isoindole-1-carboxylate were prepared by the modified Barton-Zard reaction of 2,3-bis(phenylsulfonyl)-1,2,3,4-tetrahydronaphthalene and anthracene with isocyanoacetate esters, respectively. The bis(phenylsulfonyl) derivatives were, in turn, prepared by the pericyclic reactions of the corresponding sultines with trans-1,2-bis(phenylsulfonyl)ethylene. The pyrrole esters were converted to the corresponding mono-naphthoporphyrin and mono-anthraporphyrin in good overall yields.INTRODUCTION
Inspired by the central role in biological systems, porphyrins have been extensively investigated due to their optical and electronic properties ascribed to their chemical structures. The exploitation of more efficient methodologies for the synthesis of porphyrin derivatives has been stimulated by their ever-expanding applications in biological and opto-electronical fields as photodynamic therapy agents,1 solar energy conversion materials,2 materials for nano-molecular devices,3 and nonlinear optical materials.4 π-System extension of porphyrins offered possibility of shifting the major UV-vis absorptions to longer wavelengths, a particularly valuable feature that could have wide range of applications. To gain more insights into the effects due to ring fusion on the porphyrin ring, many efforts have been made toward the synthesis of π-extended porphyrins in the last decade.5 Among the π-extended porphyrinoids, linearly fused aromatic rings exhibited their significant advantages on the red-shifting effect. However, due to the extreme instabilities of pyrroles bearing linearly-fused acenes such as benzene, naphthalene and anthracene, synthesis of the acene-fused porphyrins still required an expedient tactics. So far, two strategies have been exploited for these porphyrins. One is based on the retro-Diels-Alder extrusion of ethylene from porphyrins fused with a bicyclo[2.2.2]octadiene framework developed by our group.6 The other is based on the oxidative aromatization of porphyrins fused with non-aromatic saturated hydrocarbon rings. This has been extensively studied by Vinogradov and Cheprakov’s groups.7 With these two strategies, preparations of benzo-, naphtho-, and anthra-porphyrins have made a progress in the past several years. Nevertheless, it still remains a challenge to conveniently enlarge the conjugated system and introduce versatile functional groups on their periphery for their applications in different fields. On the searching of organic compounds with a long and large π-conjugated system for electronic communication between electrodes in nano-scale devices, we focused our attention on the synthetic methodology of linearly π-extended porphyrins, namely oppositely acene-fused porphyrins. We chose [3+1] strategy for such porphyrin synthesis8 and precursors of acene-fused pyrroles could be used for both preparations of tripyrranes and pyrrole-2,5-dicarbaldehydes. Since o-quinodimethane derivatives have been extensively exploited to extend the aromatic systems,9 we decided to utilize the highly reactive dienes to prepare the precursors. We have already reported the preparation of a bicyclo[2.2.2]octadiene-installed precursor10 of naphth[f]isoindole based on the Diels-Alder reaction of 4,7-dihydro-4,7-ethano-2H-isoindole and α,α’-dibromo-o-xylylene and their application for the synthesis of tetra-anthraporphyrins.11 Although this method is advantageous for the preparation of these acene-fused porphyrins in a highly pure form, the route is rather tedious and lengthy. Here we present a facile route to the other precursors of acene-fused pyrroles, namely 4,9-dihydro-2H-benz[f]isoindole (3a) and 4,11-dihydro-2H-naphth[f]isoindole (3b), and their utilization for the tripyrrane preparation followed by the [3+1] porphyrin synthesis giving mono-naphthoporphyrin and mono-anthraporphyrin (Scheme 1).
RESULTS AND DISCUSSION
We chose sultines as precursors for the generation of o-quinodimethane derivatives, because they were usually stable at room temperature and reacted thermally under mild conditions. As shown in Scheme 1, the modified Barton-Zard synthesis of pyrroles was employed.12 Bis-sulfones 2a and 2b were prepared by the Diels–Alder cycloaddition of trans-1,2-bis(phenylsulfonyl)ethylene and the corresponding o-quinodimethanes generated by the retro-Diels-Alder reaction of benzosultine13 and naphthosultine14 in refluxing solvents in 89 and 67% yields, respectively. Then, the α,β-unsaturated sulfones generated by elimination of a phenylsulfinate anion from the bis-sulfones under the basic conditions of t-BuOK were reacted with isocyanoacetate esters to yield pyrrole esters 3a and 3b in 76 and 63% yields, respectively.
Removal of ester groups from 3 was achieved by heating at 175 °C with KOH in ethylene glycol to give α-free pyrroles 4a and 4b in 80 and 72% yields, respectively.
With α-unsubstituted pyrroles 4a and 4b in hand, dihydronaphtho- and dihydroanthra-porphyrins 5a and 5b were prepared by well-established method via a [3+1] approach.15 Pyrroles 4 and two equivalents of tert-butyl 5-(acetoxymethyl)-3,4-diethylpyrrole-1-carboxylate were reacted under the catalysis of montmorillonite K-10 to afford di-tert-butyl tripyrrane-1,14-dicarboxylates, which were used without purification. The tert-butyl ester group of the tripyrranes was removed by treatment with TFA at room temperature, the α-free tripyrranes were reacted with 3,4-diethylpyrrole-2,5-dicarbaldehyde, and then the resulted tetrapyrrolic compounds were oxidized with 2,3-dichloro-5,6-dicyanobenzoquinone (DDQ) at room temperature to give dihydroporphyrins 5a and 5b in 25 and 13% yields, respectively. These dihydroporphyrins were rather unstable and were gradually oxidized under air. One of the oxidation products from 5a was unambiguously assigned as quinone-fused porphyrin 7 by the X-ray and MS analyses. A plausible mechanism giving 7 is as follows. Auto-oxidation of 5a would afford a hydroperoxylated dihydroporphyrin, which would then give hydroxylated aromatized porphyrin by the fission of the O-O bond. Further oxidation gave quinone 7. The porphyrins 5 were converted to π-extended porphyrins 6a (75%) and 6b (65%) by oxidation with DDQ in refluxing toluene. The similar results were obtained by the direct oxidation after the [3+1] porphyrin synthesis.
The structures of four porphyrins were characterized by 1H NMR, MALDI-TOF MS, and UV-vis spectroscopies. In the NMR spectra, the signals arising from methylene protons connecting arene and porphryin rings appeared at 5.50 and 5.67 ppm in 5a and 5b, respectively. After oxidative dehydrogenation, the naphthalene and anthracene protons adjacent to the porphyrin ring were found at very lower fields of 9.73 and 9.70 ppm in porphyrins 6a and 6b, respectively. In the UV-vis spectra of both dihydroporphyrins 5a and 5b in chloroform, slightly broad Soret bands were observed at 399 nm with the molar extinction coefficients (log ε) of 5.09 and 5.14, respectively. These values are smaller than that (log ε = 5.22) of octaethylporphyrin.16 This fact supports that the effect of dihydroacene fusion on the porphyrin π-system is more than that of alkyl substitution, namely the hyperconjugative effect observed in the methylene-connected diporphyrins.17 The observed red-shifted Q-bands of porphyrins 6a and 6b appeared at 642 (log ε = 3.90) and 659 (log ε = 4.25) nm, respectively. Additionally, the Soret band of porphyrin 6b was split into three strong absorptions at 376 (log ε = 4.80), 416 (log ε = 4.25), and 440 (log ε = 5.05) nm. These observations exhibited the very close characterization of porphyrins 6 to their respective homologous porphyrins.18
Fortunately, single crystals of dihydroacene-fused pyrrole-2-carboxylates 3a and 3b and dihydronaphthoporphyrin 5a were obtained. Ortep drawings of 3a and 3b are illustrated in Figure 2. In the structure of 3a, the molecule is slightly bent at the cyclohexadiene moiety and the torsion angle of the pyrrole and benzene mean planes is 15.6(1)°, while the molecule of 3b is almost flat and the mean plane angle of pyrrole and naphthalene rings in 3b is 1.5(1)°. In both structures, the pyrrole ring and the ester moieties are almost coplanar similarly as reported19 and the torsion angles in 3a and 3b are 2.47(8)° and 2.60(9)°, respectively. In these crystal structures, hydrogen bonding motif is quite different. In 3b, double hydrogen bonding between pyrrole hydrogen and carbonyl oxygen with the neighboring molecule
giving a dimer structure is observed and the N-H···O distance is 2.837(4) Å (Figure 3b). As an inversion center exists between pyrrole rings of the dimeric structure, the torsion angle of pyrrole rings is completely parallel. The torsion angle between the mean planes of five pyrrolic atoms and four hydrogen bonding atoms of nitrogen and oxygen is 3.0(1)°. This means that all atoms in the dimer of 3b are almost in the same plane except for atoms in the tert-butyl group. On the other hand, two kinds of the hydrogen bonds are observed in 3a (Figure 3a). Pyrrolic hydrogen of one molecule bonds to carbonyl oxygen of a neighboring molecule (2.789(4) Å), pyrrolic hydrogen of which bonds to carbonyl oxygen of another molecule (2.876(4) Å). Therefore, the hydrogen bonding shows a zigzag motif. The dihedral angle between the pyrrole rings is 58.9(1)°.
Two kinds of single crystals were obtained by slow diffusion of an i-propanol vapor into a chlorobenzene solution of dihydronaphthoporphyrin 5a. Ortep drawings are illustrated in Figure 4. One crystal A is composed of 5a and 21,26-dioxo-21,26-dihydronaphthoporphyrin 7 in a ratio of 7:1. The latter is unambiguously assigned by the X-ray and MS analyses. Another crystal B consists of 5a and solvent
chlorobenzene. In this crystal B, no other porphyrinic compound is found. In both structures of 5a, porphyrin and benzene rings are almost coplanar, and dihedral angles of these mean planes are 1.49(7)° for A and 7.24(5)° for B. The very small value of the angle in structure A may be due to the contamination of flat 7. Pyrrolic hydrogen atoms are placed at the electron peaks found in the differential Fourier maps. In the structure B of pure 5a, pyrrolic hydrogen atoms are found at the pyrrole moieties adjacent to the dihydrobenz[f]isoindole unit. Although substituent effects on the tautomerism of inner porphyrin protons are rather difficult to discuss,20 the hyperconjugative effect of the coplanar dihydronaphthalene moiety may control the porphyrinic macrocyclic ring currency similarly as found in the aromatic ring fusion.21
In conclusion, we have developed a facile approach for dihydrobenz[f]isoindole and dihydronaphth[f]isoindole by using o-quinodimethane intermediates generated from sultines. These dihydro isoindoles were successfully converted to the π-extended porphyrins. Due to the ready accessibility of sultines, we believe that the current approach could provide a facile route to naphthoporphyrins and anthraporphyrins with various substitutents, and open new possibilities for the engagement of these molecules into applied researches.
EXPERIMENTAL
General
Melting points were measured on a Yanagimoto micromelting point apparatus and are uncorrected. NMR spectra were obtained with a JEOL AL-400 or EX-400 spectrometer at the ambient temperature by using CDCl3 as a solvent and tetramethylsilane as an internal standard for 1H and 13C. IR spectra were measured as a KBr pellet with a Horiba FT-720 infrared spectrophotometer. EI and FAB MS spectra were measured with a JEOL JMS-700 in Integrated Center for Sciences. MALDI TOF MS spectra were measured with a Voyager DE Pro instrument in Venture Business Laboratory. Elemental analysis was performed with a Yanaco MT-5 elemental analyzer in Integrated Center for Sciences. X-Ray diffraction was measured with Rigaku AFC8S Mercury (for 3a) or Rigaku VariMax Saturn-724 (for 3b and 5a). All solvents and chemicals were reagent grade quality, obtained commercially, and used without further purification except for otherwise noted. Dry solvents were purchased from Kanto Chemical Co. Solvents for chromatography were purified by distillation. Thin-layer (TLC) and column chromatography with silica gel were performed on Art. 5554 (Merck KGaA) and Silica Gel 60N (Kanto Chemical Co.), respectively.
trans-2,3-Bis(phenylsulfonyl)-1,2,3,4-tetrahydronaphthalene (2a): Benzosultine (1a, 2.52 g, 15.0 mmol) in 10 mL of toluene was slowly added into the refluxing solution of trans-1,2-bis(phenylsulfonyl)ethylene (4.62 g, 15.0 mmol) in 150 mL of toluene. After addition the solution was refluxed for 3 h. After cooling to room temperature and standing for several hours, the precipitation was collected by vacuum filtration to give 6.0 g of the crude product containing a small amount of 1,3-dihydroisothianaphthene S,S-dioxide and trans-1,2-bis(phenylsulfonyl)ethylene. Recrystallization of the crude product from THF gave 5.5 g (89%) of 1a as a white solid: mp 187.2 ○C; IR /cm-1 1446, 1295, 1145, 1083; 1H NMR δ 3.15 (m, 4H), 4.16 (m, 2H), 7.05 (m, 2H), 7.14 (m, 2H), 7.57 (m, 4H), 7.69 (m, 2H), 7.81 (m, 4H); 13C NMR δ 27.27, 58.13, 127.08, 127.79, 128.80, 129.27, 132.41, 134.13, 137.15; MS (FAB): m/z 413 (M++1). Anal. Calcd for C22H20O4S2: C, 64.05; H, 4.89%. Found: C, 63.81; H, 4.86.
trans-2,3-Bis(phenylsulfonyl)-1,2,3,4-tetrahydroanthracene (2b): Naphthosultine (1b, 0.436 g, 2.00 mmol) in 10 mL of o-dichlorobenzene was slowly added into the refluxing solution of trans-1,2-bis(phenylsulfonyl)ethylene (0.616 g, 2.00 mmol) in 10 mL of o-dichlorobenzene. After addition, the solution was refluxed for 3 h. After cooling down to room temperature and standing for several hours, the precipitates were collected by filtration to give a crude product containing 1,3-dihydronaphthothiphene S,S-dioxide and trans-bis(phenylsulfonyl)ethylene. Recrystallization of the crude product from THF gave 0.62 g (67%) of pure 1b as a white powder: mp 278 °C; IR (KBr) /cm-1 1450, 1304, 1142, 1080; 1H NMR δ 3.25 (dd, J = 20.0, 5.5 Hz, 2H), 3.35 (dd, J = 20.0, 5.5 Hz, 2H), 4.23 (t, J = 5.5 Hz, 2H), 7.42 (m, 2H), 7.54 (m, 6H), 7.71 (m, 2H), 7.73 (m, 2H), 7.82 (m, 4H); 13C NMR δ 28.12, 58.47, 125.71, 126.29, 127.30, 128.93, 129.28, 130.81, 132.73, 134.19, 137.06; MS (FAB) m/z 463 (M++1). Anal. Calcd for C26H22O4S2+1/2H2O: C, 66.22; H, 4.92%. Found: C, 66.24; H, 4.88.
Ethyl 4,9-dihydro-2H-benz[f]isoindole-1-carboxylate (3a): To a stirred suspension of trans-2,3-bis(phenylsulfonyl)-1,2,3,4-tetrahydronaphthalene (2a, 1.65 g, 4.00 mmol) and ethyl isocyanoacetate (0.48 mL, 4.4 mmol) in 50 mL of dry THF, 20 mL of a 0.5 M t-BuOK solution (1.12 g in 20 mL of dry THF) was added slowly at room temperature. After the reaction mixture was stirred overnight, 10 mL of aqueous HCl and 100 mL of CHCl3 were added. The organic phase was separated, washed with water and brine, and dried over Na2SO4. Purification on the silica-gel column eluting with EtOAc/hexane (1/3) gave 0.73 g (76%) of 3a as a pale yellow solid: mp 161.2 °C; IR /cm-1 3301, 1668; 1H NMR δ 1.41 (t, J = 7.0 Hz, 3H), 3.89 (s, 2H), 4.17 (s, 2H), 4.35 (q, J = 7.0 Hz, 2H), 6.81 (s, 1H), 7.19-7.31 (m, 4H), 8.96 (br s, 1H); 13C NMR δ 14.72, 27.00, 28.32, 60.08, 117.42, 117.83, 120.46, 125.82, 125.96, 126.23, 128.87, 129.17, 134.83, 135.09, 161.35; MS (EI+) m/z 241, 212, 194, 167. Anal. Calcd for C15H15NO2: C, 74.67; H, 6.27; N, 5.81%. Found: C, 74.60; H, 6.28; N, 5.82. Single crystals were obtained by recrystallization from CHCl3/hexane. Crystallographic data: C15H15NO2; FW = 241.29, pale yellow block, 0.40 x 0.30 x 0.20 mm, monoclinic, P21, Z = 2 in a cell of dimensions a = 9.060(4) Å, b = 6.753(3) Å, c = 10.688(4) Å, β = 105.894(8)°, V = 628.9(4) Å3, Dcalc = 1.274 g·cm-3, Mo Kα, F(000) = 256, T = 298, 2249 unique reflections, 1281 with F2 > 2σ(F2). Friedel pairs are merged. The final R1 = 0.0451, wR2 (all) = 0.1141, goodness-of-fit = 1.084 for 165 parameters with no restrain refined on F2, CCDC No. 830979.
tert-Butyl 4,11-dihydro-2H-naphth[2,3-f]isoindole-1-carboxylate (3b): To a stirring suspension of 2,3-bis(phenylsulfonyl)-1,2,3,4-tetrahydroanthracene (2b, 0.92 g, 2.0 mmol) and tert-butyl isocyanoacetate (0.30 g, 2.4 mmol) in 50 mL of dry THF, 5 mL of 1 M t-BuOK solution was added slowly at room temperature. After the reaction mixture was stirred overnight, 10 mL of aq. HCl and 100 mL of CHCl3 were poured into. The organic phase was washed with water and brine, and dried over Na2SO4. Purification on silica gel eluting with EtOAc/hexane (1/3) gave 0.40 g (63%) of 3b as a white solid: mp 226 °C; IR /cm-1 3309, 3047, 1658 ; 1H NMR δ 1.64 (s, 9H), 4.05 (s, 2H), 4.33 (s, 2H), 6.82 (d, J = 2.9 Hz, 1H), 7.41 (m, 2H), 7.73-7.79 (m, 4H), 8.86 (br s, 1H); 13C NMR (typical signals) δ 27.80, 28.64, 28.87, 80.73, 116.97, 118.70, 120.98, 125.15, 126.98, 127.04, 132.11, 132.20, 134.38, 134.51, 160.86; MS (FAB) m/z 320 (M++1). Anal. Calcd for C21H21NO2+1/8H2O: C, 78.42; H, 6.66; N, 4.35%. Found: C, 78.20; H, 6.74; N, 4.35. Single crystals were obtained by recrystallization from chloroform/methanol. Crystallographic data: C21H21NO2; FW = 319.40, pale yellow needle, 0.40 x 0.10 x 0.03 mm, monoclinic, P21/c, Z = 4 in a cell of dimensions a = 6.238(2) Å, b = 10.247(3) Å, c = 25.951(9) Å, β = 97.356(6)°, V = 1645.2(9) Å3, Dcalc = 1.289 g·cm-3, Mo Kα, F(000) = 680, T = 100, 3653 unique reflections, 2465 with F2 > 2σ(F2). The final R1 = 0.0700, wR2 (all) = 0.1791, goodness-of-fit = 1.008 for 246 parameters with 126 restrains refined on F2, CCDC No. 830980.
4,9-Dihydro-2H-benz[f]isoindole (4a): A stirring mixture of pyrrole ester 3a (0.96 g 4.0 mmol) and KOH (1.2 g, 20 mmol) in 25 mL of ethylene glycol was heated at 175 °C under N2 for 2 h. The reaction mixture was poured into ice water, and then extracted with CHCl3 (50 mL). The organic phase was washed with saturated aqueous NaHCO3 solution, water and brine, and dried over Na2SO4. After removal of the solvent under the vacuum, the residue was purified by flash chromatography eluting with CHCl3 to give 0.54 g (80%) of 4a as a pale gray powder: mp 97 °C; IR /cm-1 3428, 3378; 1H NMR δ 3.90 (s, 4H), 6.59 (d, J = 2.9 Hz, 2H), 7.15 (m, 2H), 7.24 (m, overlapped with solvent, 2H), 7.91 (br s, 1H); 13C NMR δ 27.63, 112.53, 118.47, 125.57, 128.84, 136.45; MS (FAB) m/z 170 (M++1). Anal. Calcd for C12H11N: C, 85.17; H, 6.55; N, 8.28%. Found: C, 85.08; H, 6.70; N, 8.15.
4,11-Dihydro-2H-naphth[2,3-f]isoindole (4b): A stirred mixture of pyrrole tert-butyl ester 3b (0.32 g 1.0 mmol) and KOH (0.6 g, 10 mmol) in 10 mL of ethylene glycol was heated at 175 °C under N2 for 1 h. After cooling down to room temperature, the reaction mixture was poured into ice water, and then extracted with CHCl3. The organic phase was washed with saturated NaHCO3 solution, water and brine, and then dried over Na2SO4. After concentration under vacuum, the residue was purified by flash chromatography on silica gel to give 0.16 g (72%) of 4b as a beige powder: mp 156 °C; IR /cm-1 3478, 3047; 1H NMR δ 4.06 (s, 4H), 6.68 (d, J = 2.9 Hz, 2H), 7.38 (m, 2H), 7.73-7.77 (m, 4H), 7.96 (br. s, 1H); 13C NMR δ 28.39, 112.58, 119.32, 125.04, 126.47, 127.07, 132.19, 136.05; MS (FAB) m/z 220 (M++1). Anal. Calcd for C16H13N+1/8H2O: C, 86.75; H, 6.32; N, 6.03%. Found: C, 86.85; H, 6.07; N, 6.36.
7,8,12,13,17,18-Hexaethyl-21,26-dihydro-22H,24H-naphtho[2,3-b]porphyrin (5a): A mixture of pyrrole 4a (84 mg, 0.50 mmol), with tert-butyl 5-(acetoxymethyl)-3,4-diethylpyrrole-2-carboxylate (295 mg, 1.00 mmol), and 0.5 g of montmorillonite K-10 clay in 30 mL of CH2Cl2 was stirred under an atmosphere of nitrogen and protection from light for 3 h. After the clay was filtered off, the solution was shaken in the presence of aqueous NaHCO3 and then washed with water and brine, and dried over Na2SO4. Removal of the solvent under vacuum gave tripyrrane di-tert-butyl ester as a crude product. Without further purification, the foregoing tripyrrane ester was dissolved in 3 mL of trifluoroacetic acid under N2 and protection from light. The mixture was stirred at room temperature for 10 min. The solution was diluted with 100 mL of dry CH2Cl2, followed immediately by the addition of 3,4-diethylpyrrole-2,5-dicarbaldehyde (89.6 mg, 0.500 mmol), and the resulting solution was stirred at room temperature for 8 h. The mixture was neutralized by the slowly addition of triethylamine (5 mL) and then DDQ (112 mg 0.500 mmol) was added. The mixture was allowed to stir for an additional 2 h. The mixture was washed with aqueous NaHCO3, water, and brine, and dried over Na2SO4. The solvent was removed under a reduce pressure to give a dark red residue. The residue was purified by flash chromatography on silica gel eluting with CHCl3 to give a dark red powder (73 mg, 25%). 1H NMR δ -3.73 (br. s, 2H), 1.94 (m, 18H), 4.13 (m, 12H), 5.50 (s, 4H), 7.48 (m, 2H), 7.81 (m, 2H), 10.06 (s, 2H), 10.12 (s, 2H); MALDI-TOF MS m/z 580.95 (M+); UV-vis (CHCl3) λmax/nm (log ε) 644 (3.90), 620 (3.72), 565 (3.80), 550 (4.07), 535 (3.91), 498 (4.04), 468 (3.88), 399 (5.09). HRMS (FAB), calcd for C40H44N4+H+: 581.3644. Found: 581.3655. Three morphologically different crystals were obtained by slow diffusion of an i-propanol vapor into a solution of 5a in chlorobenzene: needles, rhombic platelets, and rectangular rods. The former two crystals are proved to be the same structure of A and the last crystals are the structure B. Crystallographic data of structure A: 0.88(C40H44N4)+0.12(C40H40N4O2); FW = 584.18, red needle, 0.40 x 0.10 x 0.06 mm, triclinic, P-1, Z = 2 in a cell of dimensions a = 9.013(4) Å, b = 11.974(4) Å, c = 15.361(7) Å, α = 75.524(15)°, β = 84.475(15)°, γ = 81.002(15)°, V = 1582.5(11) Å3, Dcalc = 1.226 g·cm-3, Mo Kα, F(000) = 626.88, T = 100, 7199 unique reflections, 4729 with F2 > 2σ(F2). The final R1 = 0.0722, wR2 (all) = 0.1965, goodness-of-fit = 1.090 for 440 parameters with 129 restrains refined on F2, CCDC No. 831582. Similar composition (5a:7 = 7:1) and structures are obtained by the analysis of rhombic platelet crystals (crystal of 0.20 x 0.15 x 0.05 mm: R1 = 0.0677, wR2 (all) = 0.1852, goodness-of-fit = 1.075, and crystal of 0.15 x 0.10 x 0.01 mm: R1 = 0.0672, wR2 (all) = 0.1938, goodness-of-fit = 1.069). These analysis data as cif files are also deposited (CCDC No. 831583 and 831584, respectively). In order to confirm the contamination of 7,8,12,13,17,18-hexaethyl- 21,26-dioxo-21,26-dihydro-22H,24H-naphtho[2,3-b]porphyrin (7), the crystals were subject to MS analysis. In addition to a large peak of m/z = 581 due to 5a+H+, a peak of m/z = 609 due to 7+H+ was observed in the FAB-MS spectrum. The HRMS measurement proved that the composition of m/z = 609 was C40H40N4O2+H+ (Calcd: 609.3230. Found: 609.3225). Crystallographic data of structure B: C40H44N4+C6H5Cl; FW = 693.34, red block, 0.20 x 0.10 x 0.10 mm, triclinic, P-1, Z = 2 in a cell of dimensions a = 10.092(3) Å, b = 12.233(4) Å, c = 16.792(5) Å, α = 91.446(3)°, β = 102.979(4)°, γ = 114.055(3)°, V = 1828.7(10) Å3, Dcalc = 1.259 g·cm-3, Mo Kα, F(000) = 740, T = 100, 8314 unique reflections, 6575 with F2 > 2σ(F2). The final R1 = 0.0507, wR2 (all) = 0.1501, goodness-of-fit = 1.074 for 466 parameters with no restrain refined on F2, CCDC No. 831585.
7,8,12,13,17,18-Hexaethyl-21,28-dihydro-22H,24H-anthra[2,3-b]porphyrin (5b): The reaction was conducted in the similar manner as described above. Flash chromatography on silica gel eluting with CHCl3 gave 85 mg (13%) of 5b as a dark purple powder. 1H NMR δ -3.71 (s, 2H), 1.92 (m, 18H), 4.09 (m, 12H), 5.67 (s, 4H), 7.56 (m, 2H), 8.00 (m, 2H), 8.31 (s, 2H), 10.11 (s, 2H), 10.14 (s, 2H); MS (FAB) m/z 631 (M++1); UV-vis (CHCl3) λmax/nm (log ε) 620 (3.71), 600 (3.20), 566 (3.87), 535 (4.01), 498 (4.08), 399 (5.14). HRMS (FAB), calcd for C44H46N4+H+: 631.3801. Found: 631.3797.
7,8,12,13,17,18-Hexaethyl-22H,24H-naphtho[2,3-b]porphyrin (6a): Dihydronaphtho[2,3-b] porphyrin 5a (11.6 mg, 0.020 mmol) was dissolved in 10 mL of toluene. To this solution DDQ (0.020 mmol) was added, and the solution was heated at reflux for 5 min. After cooling, the solvent was evaporated under vacuum, and the residue was washed with copious methanol to give 9 mg (75%) of the title porphyrin as a purple solid. 1H NMR δ -3.29 (s, 2H), 1.89 (t, J = 7.0 Hz, 6H), 1.95 (t, J = 7.0 Hz, 6H), 2.01 (t, J = 7.0 Hz, 6H), 4.01 (q, J = 7.0 Hz, 4H), 4.19 (q, J = 7.0 Hz, 4H), 4.23 (q, J = 7.0 Hz, 4H), 7.81 (m, 2H), 8.55 (m, 2H), 9.73 (s, 2H), 10.06 (s, 2H), 10.42 (s, 2H); 13C NMR δ 18.36, 18.41, 18.66, 19.63, 19.68, 19.87, 92.90, 97.56, 118.92, 125.80, 129.17, 132.87, 134.30, 135.89, 137.47, 139.13, 139.83, 143.78, 150.27 and one sp2 carbon is not seen; MALDI-TOF MS m/z 578.90; UV-vis (CHCl3) λmax/nm (log ε) 642 (3.90), 557 (4.22), 518 (3.62), 423 (5.11), 354 (4.25). HRMS (FAB), calcd for C40H42N4+H+: 579.3488. Found: 579.3486.
7,8,12,13,17,18-Hexaethyl-22H,24H-anthra[2,3-b]porphyrin (6b): The reaction was conducted in the similar manner as described above to give 12 mg (65%) of the title porphyrin as a dark red powder. 1H NMR δ 1.90 (m, 18H), 3.97 (m, 4H), 4.14 (m, 8H), 7.61 (m, 2H), 8.21 (m, 2H), 9.04 (s, 2H), 9.70 (s, 2H), 9.97 (s, 2H), 10.21 (s, 2H); MALDI-TOF MS m/z 628.79; UV-vis (CHCl3) λmax/nm (log ε) 659 (4.25), 601 (3.66), 562 (4.58), 529 (3.85), 440 (5.05), 416 (4.80), 376 (4.80). HRMS (FAB), calcd for C44H44N4+H+: 629.3644. Found: 629.3646.
ACKNOWLEDGEMENTS
This work was partially supported by Grant-in-Aid for Scientific Research B (23350020) from Ministry of Education, Culture, Sports, Science and Technology of Japan.
References
1. M. R. Detty, S. L. Gibson, and S. J. Wagner, J. Med. Chem., 2004, 47, 3897; CrossRef I. J. Macdonald and T. J. Dougherty, J. Porphyrins Phthalocyanines, 2001, 5, 105. CrossRef
2. H. Imahori, T. Umeyama, and S. Ito, Acc. Chem. Res., 2009, 42, 1809; CrossRef W. M. Campbell, A. K. Burrell, D. L. Officer, and K. W. Jolley, Coord. Chem. Rev., 2004, 248, 1363; CrossRef M.-S. Choi, T. Yamazaki, I. Yamazaki, and T. Aida, Angew. Chem. Int. Ed., 2004, 43, 150. CrossRef
3. H. Yamada, T. Okujima, and N. Ono, Chem. Commun., 2008, 2957; CrossRef S. U. Lee, R. V. Belosludov, H. Mizuseki, and Y. Kawazoe, Small, 2008, 4, 962; CrossRef H. L. Anderson, Chem. Commun., 1999, 2323; CrossRef D. Holten, D. F. Bocian, and J. S. Lindsey, Acc. Chem. Res., 2002, 35, 57. CrossRef
4. M. O. Senge, M. Fazekas, E. G. A. Notaras, W. J. Blau, M. Zawadzka, O. B. Locos, and E. M. Ni Mhuircheartaigh, Adv. Mater., 2007, 19, 2737; CrossRef N. Aratani, D. Kim, and A. Osuka, Chem. Asian J., 2009, 4, 1172. CrossRef
5. For review, see: T. D. Lash, J. Porphyrins Phthalocyanines, 2001, 5, 267. CrossRef
6. S. Ito, T. Murashima, H. Uno, and N. Ono, Chem. Commun., 1998, 1661; CrossRef S. Ito, N. Ochi, T. Murashima, N. Ono, and H. Uno, Chem. Commun., 2000, 893. CrossRef
7. For the recent examples, see: O. S. Finikova, A. V. Cheprakov, P. J. Carroll, and S. A. Vinogradov, J. Org. Chem., 2003, 68, 7517; CrossRef O. Finikova, A. Galkin, V. Rozhkov, M. Cordero, C. Hägerhäll, and S. Vinogradov, J. Am. Chem. Soc., 2003, 125, 4882; CrossRef O. S. Finikova, A. V. Cheprakov, and S. A. Vinogradov, J. Org. Chem., 2005, 70, 9562; CrossRef O. S. Finikova, S. E. Aleshchenkov, R. P. Brinas, A. V. Cheprakov, P. J. Carroll, and S. A. Vinogradov, J. Org. Chem., 2005, 70, 4617; CrossRef L. Jiao, E. Hao, F. R. Fronczek, M. G. H. Vicente, and K. M. Smith, Chem. Commun., 2006, 3900; CrossRef A. V. Cheprakov and M. A. Filatov, J. Porphyrins Phthalocyanines, 2009, 13, 291. CrossRef
8. T. D. Lash, Chem. Eur. J., 1996, 2, 1197. CrossRef
9. For recent examples, see: G. S. Tulevski, Q. Miao, M. Fukuto, R. Abram, B. Ocko, R. Pindak, M. L. Steigerwald, C. R. Kagan, and C. Nuckolls, J. Am. Chem. Soc., 2004, 126, 15048; CrossRef T. Okamoto, M. L. Senatore, M.-M. Ling, A. B. Mallik, M. L. Tang, and Z. Bao, Adv. Mater., 2007, 19, 3381; CrossRef C. R. Swartz, S. R. Parkin, J. E. Bullock, J. E. Anthony, A. C. Mayer, and G. G. Malliaras, Org. Lett., 2005, 7, 3163. CrossRef
10. H. Uoyama, C. Cai, H. Tahara, Y. Shimizu, H. Hagiwara, Y. Hanasaki, H. Yamada, T. Okujima, and H. Uno, Heterocycles, 2010, 80, 1187. CrossRef
11. H. Yamada, D. Kuzuhara, T. Takahashi, Y. Shimizu, K. Uota, T. Okujima, H. Uno, and N. Ono, Org. Lett., 2008, 10, 2947; CrossRef H. Yamada, K. Ohkubo, D. Kuzuhara, T. Takahashi, A. S. D. Sandanayaka, T. Okujima, K. Ohara, O. Ito, H. Uno, N. Ono, and S. Fukuzumi, J. Phys. Chem. B, 2010, 114, 14717. CrossRef
12. T. Okujima, Y. Hashimoto, G. Jin, H. Yamada, H. Uno, and N. Ono, Tetrahedron, 2008, 64, 2405. CrossRef
13. M. D. Hoey and D. C. Dittmer, J. Org. Chem., 1991, 56, 1947. CrossRef
14. A.-T. Wu, W.-D. Liu, and W.-S. Chung, J. Chin. Chem. Soc., 2002, 49, 77; K. Sambasivarao and G. A. Kumar, Synthesis, 2004, 558. CrossRef
15. A. Boudif and M. Momenteau, J. Chem. Soc., Chem. Commun., 1994, 2069; CrossRef A. Boudif and M. Momenteau, J. Chem. Soc., Perkin Trans. 1, 1996, 1235. CrossRef
16. “Organic Electronic Spectral Data, VII” ed. by J. P. Phillips, J. C. Dacons, and R. G. Rice, John Wiley & Sons, New York, 1964-1965, p. 1244.
17. J. B. Paine III, D. Dolphin, and M. Gouterman, Can. J. Chem., 1978, 56, 1712; CrossRef H. Uno, K. Nakamoto, K. Kuroki, A. Fujimoto, and N. Ono, Chem. Eur. J., 2007, 13, 5773. CrossRef
18. S. Ito, N. Ochi, H. Uno, T. Murashima, and N. Ono, Chem. Commun., 2000, 893; CrossRef H. Yamada, D. Kuzuhara, K. Ohkubo, T. Takahashi, T. Okujima, H. Uno, N. Ono, and S. Fukuzumi, J. Mater. Chem., 2010, 20, 3011. CrossRef
19. H. Uno, S. Ito, M. Wada, H. Watanabe, M. Nagai, A. Hayashi, T. Murashima, and N. Ono, J. Chem. Soc., Perkin Trans. 1, 2000, 4347. CrossRef
20. M. J. Crossley, M. M. Harding, and S. Sternhell, J. Am. Chem. Soc., 1986, 108, 3608. CrossRef
21. H. Uno, Y. Shimizu, H. Uoyama, Y. Tanaka, T. Okujima, and N. Ono, Eur. J. Org. Chem., 2008, 87. CrossRef