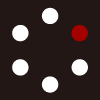
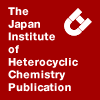
HETEROCYCLES
An International Journal for Reviews and Communications in Heterocyclic ChemistryWeb Edition ISSN: 1881-0942
Published online by The Japan Institute of Heterocyclic Chemistry
e-Journal
Full Text HTML
Received, 5th July, 2011, Accepted, 8th August, 2011, Published online, 16th August, 2011.
DOI: 10.3987/COM-11-S(P)77
■ Deprotection of 3,4-Dimethoxybenzyl (3,4DMB) Group on γ-Lactam Nitrogen Using Phenyliodine(III) Bis(trifluoroacetate) (PIFA): Application to Isoindolinone Compounds
Kazuhiro Watanabe, Hiroaki Shibata, Yū Imai, and Tadashi Katoh*
Department of Synthetic Organic Chemistry, Tohoku Pharmaceutical University, 4-4-1 Komatsushima, Aoba-ku, Sendai 981-8558, Japan
Abstract
The secondary amide (γ-lactam) moiety of an isoindolinone ring exhibits high polarity in an unprotected condition. Problems with solubility make handling difficult; therefore, introduction of a protective group is necessary. We discovered that the 3,4-dimethoxybenzyl (3,4DMB) group is an optimal protective group, and that the 3,4DMB group alone could be deprotected under mild conditions with the use of a hypervalent iodine(III) reagent, phenyliodine(III) bis(trifluoroacetate) (PIFA).Protection and deprotection reactions represent an extremely important process in basic research for organic synthesis chemistry, and numerous examples of these reactions have been reported.1 In particular, in the field of total synthesis research, these protection and deprotection reactions can be sufficiently critical to influence subsequent synthetic plans.
Previously, in our total synthesis research for (+)-stachyflin (1) with anti-influenza A virus activity, we reported on the synthesis of the isoindolinone ring (D and E in Scheme 1).2 In syntheses where the amide moiety (γ-lactam) at position 16 on the isoindolinone ring was unprotected, we learned that solubility in the reaction solvent presented a problem, because the compound itself had high polarity. Consequently, we synthesized isoindolinone compound 2, protecting the amide moiety (γ-lactam) with a 3,4-dimethoxybenzyl (3,4DMB or veratryl)1,3 group, and performed a deprotection reaction at room temperature with the hypervalent iodine(III) reagent phenyliodine(III) bis(trifluoroacetate) (PIFA)4 in CH2Cl2 solvent. Although the yield was moderate, the deprotected product 3 was successfully obtained with the 3,4DMB group selectively removed. In the deprotection reaction for 2, the 3,4DMB group is a protective benzyl group located at position 16, but benzyl positions also exist at C10 and C17. Thus, ordinary debenzylation conditions such as general catalytic reduction conditions (Pd-C, H2, etc.) or oxidizing agents (such as 2,3-dichloro-5,6-dicyano-1,4-benzoquinone (DDQ)3b,5 and ceric ammonium nitrate (CAN)6) do not allow the reaction to proceed, or else generate complex mixtures. We discovered that this deprotection reaction worked only when PIFA was used. No previous reports have discussed a deprotection reaction for the 3,4DMB group using PIFA.2 This deprotection reaction is thus expected to be very useful in the field of organic synthesis chemistry.
For this detailed report, we studied the optimal conditions for the deprotection reaction believed to be useful in the synthesis of isoindolinone compounds containing a secondary amide (γ-lactam). Hence, isoindolinone compounds 4a-d2 containing benzyl-type protective groups were synthesized and reacted with the hypervalent iodine(III) reagent PIFA, and detailed reaction conditions were studied in order to efficiently remove the benzyl-type protective groups (Scheme 2).
From previous research, we know that catalytic reduction conditions (such as H2-Pd/C and H2-Raney Ni) or usage of DDQ as an oxidizing agent applied to isoindolinone compounds 4a-d do not allow the expected debenzylation reaction to proceed at all, and instead, starting materials are recovered. Nonetheless, we learned that 4b containing the PMB group and 4c containing the 3,4DMB group afforded isoindolinone 5a in moderate yield when CAN was used as an oxidizing agent. Subsequently, we studied in detail the deprotection reaction of PIFA, the hypervalent iodine(III) reagent found to be effective in the total synthesis of (+)-stachyflin (1) (Scheme 2, Table 1).
First, for substrate 4a containing the benzyl group and substrate 4b containing the PMB group, 10 equivalents of PIFA were employed to perform deprotection reactions at room temperature in CH2Cl2 or benzene solvent. Trace amounts of the deprotection target isoindolinone 5a were obtained (entries 3, 4) from substrate 4b, but the reaction scarcely progressed for 4a and starting materials were recovered (entries 1, 2). For isoindolinone 4c containing the 3,4DMB group, a protective group more rich in electrons than the PMB group and believed to be more easily removed in deprotection, phenyliodine(III) diacetate (PIDA), a hypervalent iodine(III) reagent, was allowed to react in CH2Cl2 solvent for 24 hours or more, but 5a was not obtained (entry 5). A deprotection reaction for 4c performed in CH2Cl2 solvent employing 10 equivalents of PIFA was completed in 8 hours, and successfully provided the desired deprotection target isoindolinone 5a at 83% yield (entry 6). Based on these reaction conditions, the optimization of PIFA equivalents and reaction solvent was studied (entries 6 to 14). First, the study of PIFA equivalents revealed that a decrease from 5 to 2 equivalents led to extended reaction time and decreased yield, while the use of 10 equivalents of PIFA gave satisfactory results (entries 6 to 8).
Next, the study of reaction solvents showed that 1,2-dichloroethane (CH2ClCH2Cl), a halogenated hydrocarbon solvent similar to CH2Cl2 extended the reaction time (18 h) with a moderate yield of 5a (entry 9), but the nonpolar solvent benzene caused the reaction to conclude in 7 hours and the deprotected target isoindolinone 5a was successfully obtained at 93% yield (entry 10). As compared with the reaction performed in CH2Cl2 solvent, the yield improved and reaction time was slightly shorter. In contrast, the reaction made no progress in protic solvent EtOH (entry 11), and yielded a complex mixture in aprotic solvent MeCN (entry 12). Furthermore, in solvents 2,2,2-trifluoroethanol (TFEA) and 1,1,1,3,3,3-hexafluoro-2-propanol (HFIP) used extensively for PIFA reactions, disappearance of starting materials was observed in a short period of time, but similarly to MeCN solvent, decomposition products were obtained (entries 13,14). These results made it clear that the use of the nonpolar solvent benzene was optimal for this deprotection reaction.
Under the optimized reaction condition, a wide range of deprotective reaction of isoindolinone 4d containing acetonide was investigated using PIFA (10 equiv.) and the results are shown in Table 1 (entries 15, 16). Not surprisingly, due to the instability of acetonide moiety under acidic conditions, the TFA formed from reduction of the PIFA reagent cleaved the acetonide, and provided diol 5b as the major product from 4d. In the case of entry 16, the reaction of 4d was carried out under usual condition (PIFA, benzene, rt) in the presence of K2CO3 (20 equiv.) as a base, to increase the selectivity of deprotected product 5c (77% yield).
The present deprotection reaction mechanism was elucidated based on the following: i) Yonemitsu et al. 3a reacted DDQ with a compound containing an alcohol protected by a PMB group and a 3,4DMB group within the same molecule, and reported that the 3,4DMB group with a higher electron density than the PMB group was deprotected earlier; and ii) the deprotection reaction for isoindolinone 4c employing PIFA was confirmed to generate 3,4-dimethoxybenzaldehyde together with 5a (Scheme 3). PIFA was probably involved in the same sort of deprotection mechanism of the 3,4DMB group as DDQ. In other words, PIFA formed a charge transfer (CT) complex under a single-electron-transfer (SET) mechanism for the 3,4DMB group that had a higher electron density within its aromatic ring and that subsequently cleaved as 3,4-dimethoxybenzaldehyde from trace amounts of water in post-processing or in the reaction system after the hydrogen at the benzyl position was removed. The desired isoindolinone 5a with secondary amide was thus obtained, in accordance with the above supposition.
As described above, the 3,4DMB group represents the optimal protective group for the secondary amide (γ-lactam) moiety of an isoindolinone ring with an extraordinarily high polarity and difficult handling characteristics. This protective element also has good solubility towards solvents and can be handled relatively stably against general acidic or alkaline conditions. In addition, experimental results showed that the 3,4DMB group of isoindolinone 4c had sufficient capacity to endure hydrogenation and oxidizing agents such as DDQ. Moreover, the use of PIFA for deprotection of the 3,4DMB group of 4c enables simple removal under mild reaction conditions (in benzene solvent at room temperature), and deprotected isoindolinone 5a can be obtained in high yield.
EXPERIMENTAL
Routine monitorings of reaction were carried out using glass- supported Merck silica gel 60 F254 TLC plates. Flash column chromatography was performed on Kanto Chemical Silica Gel 60N (spherical, neutral 40–50 nm) with the solvents indicated. Measurements of optical rotations were performed with a JASCO DIP-370 automatic digital polarimeter. 1H and 13C NMR spectra were measured with a JEOL AL-400 (400 MHz) spectrometer. Chemical shifts were expressed in ppm using Me4Si (δ = 0) as an internal standard. The following abbreviations is used: singlet (s) and broad singlet (brs). Infrared (IR) spectral measurements were carried out with a JASCO FT/IR-4100 spectrometer. Low- and High-resolution mass (HRMS) spectra were measured on a JEOL JMS-DX 303/JMA-DA 5000 SYSTEM high resolution mass spectrometer.
4-Acetoxy-5-acetoxymethyl-6-methoxyisoindolin-1-one (5a)
General procedure (Table 1, entry 10): Phenyliodine(III) bis(trifluoroacetate) (PIFA) (194 mg, 0.45 mmol) was added in small portions to a stirred solution of 4c (20 mg, 45 μmol) in dry benzene (5 mL) at room temperature. After 7 h, the reaction was quenched with 10% aqueous Na2S2O3 (10 mL) at room temperature, and the mixture was extracted with CHCl3 (3 x 10 mL). The combined extracts were washed with brine (10 mL), then dried over Na2SO4. Concentration of the solvent in vacuo afforded a residue, which was purified by column chromatography (hexane/AcOEt 3:1) to give 5a (12.3 mg, 93%) as a colorless amorphous powder; IR (KBr) 1767, 1738, 1699, 1474, 1433, 1368, 1336, 1110, 1024, 769, 728 cm−1; 1H NMR (400 MHz, CDCl3): δ 2.04 (s, 3H), 2.36 (s, 3H), 3.94 (s, 3H), 4.28 (s, 2H), 5.23 (s, 2H), 6.74 (brs, 1H), 7.31 (s, 1H); 13C NMR (100 MHz, CDCl3): δ 20.5, 20.8, 43.9, 55.3, 56.5, 103.5, 120.9, 128.3, 134.6, 145.6, 159.9, 168.2, 170.7, 170.8; HRMS (EI): m/z: calcd for C14H15NO6, 293.2720, found 293.2718 [M]+.
4-Hydroxy-5-(hydroxymethyl)-6-methoxyisoindolin-1-one (5b)
Table 1, entry 15: Phenyliodine(III) bis(trifluoroacetate) (PIFA) (215 mg, 0.5 mmol) was added in small portions to a stirred solution of 4d (20 mg, 50 μmol) in dry benzene (5 mL) at room temperature. After 8 h, the reaction was quenched with 10% aqueous Na2S2O3 (10 mL) at room temperature, and the mixture was extracted with CHCl3 (3 x 10 mL). The combined extracts were washed with brine (10 mL), then dried over Na2SO4. Concentration of the solvent in vacuo afforded a residue, which was purified by column chromatography (hexane/AcOEt 1:5 to 0:1) to give 5b (7.1 mg, 68%) as a colorless solid; Recrystallization from EtOAc afforded colorless prisms, mp 197–199 °C; IR (KBr) 1738, 1699, 1474, 1433, 1336, 1110, 1024, 769, 728 cm−1; 1H NMR (400 MHz, CDCl3): δ 3.53 (s, 1H), 3.84 (s, 3H), 4.34 (s, 2H), 4.88 (s, 2H), 6.13 (brs, 1H), 6.92 (s, 1H), 8.73 (s, 1H); 13C NMR (100 MHz, CDCl3): δ 43.9, 54.4, 56.1, 98.3, 123.0, 127.6, 134.6, 151.4, 156.1, 173.7; HRMS (EI): m/z: calcd for C10H11NO4, 209.0688, found 209.0684 [M]+.
5-Methoxy-2,2-dimethyl-8,9-dihydro-[1,3]dioxino[4,5-e]isoindol-7(4H)-one (5c)
Table 1, entry 16: Phenyliodine(III) bis(trifluoroacetate) (PIFA) (215 mg, 0.5 mmol) was added in small portions to a stirred solution of 4d (20 mg, 50 μmol) and K2CO3 (138 mg, 1.0 mmol) in dry benzene (5 mL) at room temperature. After 12 h, the reaction was quenched with 10% aqueous Na2S2O3 (10 mL) at room temperature, and the mixture was extracted with CHCl3 (3 x 10 mL). The combined extracts were washed with brine (10 mL), then dried over Na2SO4. Concentration of the solvent in vacuo afforded a residue, which was purified by column chromatography (hexane/AcOEt 1:1 to 0:1) to give 5c (9.6 mg, 77%) as a pale yellow solid; Recrystallization from EtOAc afforded colorless prisms, mp 205–207 °C; IR (KBr) 3200, 2227, 1696, 1628, 1607, 1477, 1374, 1354, 1108, 839, 733 cm−1; 1H NMR (400 MHz, CDCl3): δ 1.56 (s, 6H), 3.87 (s, 3H), 4.31 (s, 2H), 4.84 (s, 2H), 6.93 (s, 1H), 7.27 (brs, 1H); 13C NMR (100 MHz, CDCl3): δ 24.6 (2C), 42.8, 55.5, 58.5, 66.9, 96.3, 111.9, 123.9, 132.3, 146.9, 156.5, 171.9; HRMS (FAB): m/z: calcd for C13H15NO4, 249.1001, found 249.0989 [M]+.
ACKNOWLEDGEMENTS
This work was supported by the Ministry of Education, Culture, Sports, Science, and Technology of Japan (MEXT): a Grant-in-Aid for Scientific Research on Priority Area “Creation of Biologically Functional molecules” (No. 17035073 and 18032065), a Grant-in-Aid for Scientific Research (No. 20790016 and 23790018) and a Grant-in-Aid for matching Fund Subsidy for Private Universities.
References
1. T. W. Greene and P. G. M. Wuts, 'Protective Groups in Organic Synthesis, 4 th ed., John Wiley & Sons, New York, 2007.
2. (a) K. Watanabe, J. Sakurai, H. Abe, and T. Katoh, Chem. Commun., 2010, 46, 4055; CrossRef (b) J. Sakurai, T. Kikuchi, O. Takahashi, K. Watanabe, and T. Katoh, Eur. J. Org. Chem., 2011, 2948. CrossRef
3. (a) Y. Oikawa, T. Tanaka, K. Horita, T. Yoshioka, and O. Yonemitsu, Tetrahedron Lett., 1984, 25, 5393; CrossRef (b) S. Chandrasekhar, G. Sumlthra, and J. S. Yadav, Tetrahedron Lett., 1996, 37, 1645; CrossRef Deprotection of N-2,4DMB maleimides: (c) D. J. Watson, E. D. Dowdy, W.-S. Li, J. Wang, and R. Polniaszek, Tetrahedron Lett., 2001, 42, 1827. CrossRef
4. For recent reviews and publications focusing on their use in organic synthesis, see: (a) A. Varvoglis, Tetrahedron, 1997, 53, 1179; CrossRef (b) V. V. Zhdankin and P. J. Stang, Chem. Rev., 2002, 102, 2523; CrossRef (c) T. Wirth, M. Ochiai, V. V. Zhdankin, G. F. Koser, H. Tohma, and Y. Kita, 'Hypervalent Iodine Chemistry-Modern Developments in Organic Synthesis: Topics in Current Chemistry, Springer, Berlin, 2003; (d) T. Wirth, Angew. Chem. Int. Ed. 2005, 44, 3656; CrossRef (e) V. V. Zhdankin and P. J. Stang, Chem. Rev., 2008, 108, 5299; CrossRef (f) R. M. Moriarty and O. Prakash, 'Hypervalent Iodine in Organic Chemistry: Chemical Transformations, John Wiley & Sons, New York, 2007; (g) V. V. Zhdankin, ARKIVOC, 2009, 1; (h) T. Dohi and Y. Kita, Chem. Commun., 2009, 2073. CrossRef
5. (a) H.-D. Becker, 'Chemistry of the Quinoid Compounds, Wiley, New York, 1974, p. 335; (b) A. B. Turner, 'Synthetic Reagents, Wiley, New York, 1977, p. 193; (c) Y. Oikawa, T. Yoshioka, and O. Yonemitsu, Tetrahedron Lett., 1982, 23, 885; CrossRef (d) K. Horita, T. Yoshioka, T. Tanaka, Y. Oikawa, and O. Yonemitsu, Tetrahedron, 1986, 42, 3021. CrossRef
6. R. Johansson and B. Samuelsson, J. Chem. Soc., Perkin Trans. 1, 1984, 2371. CrossRef