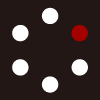
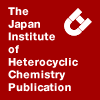
HETEROCYCLES
An International Journal for Reviews and Communications in Heterocyclic ChemistryWeb Edition ISSN: 1881-0942
Published online by The Japan Institute of Heterocyclic Chemistry
e-Journal
Full Text HTML
Received, 30th July, 2011, Accepted, 5th September, 2011, Published online, 13th September, 2011.
DOI: 10.3987/COM-11-S(P)88
■ Design and Synthesis of G-Quadruplex Ligands Bearing Macrocyclic Hexaoxazoles with Four-Way Side Chains
Keisuke Iida, Satoki Majima, Terumi Ohtake, Masayuki Tera, Kazuo Shin-ya, and Kazuo Nagasawa*
Division of Biotechnology and Life Science, Institute of Symbiotic Science and Technology, Tokyo University of Agriculture and Technology, 2-24-16 Nakamachi, Koganei, Tokyo 184-8588, Japan
Abstract
Macrocyclic hexaoxazole compounds bearing four-way side chains L2H2-(4M)6OTD derivatives 3 and 4 were synthesized as new G-quadruplex ligands. Stabilization of G-quadruplex forming oligonucleotides in human telomeres, c-kit, bcl-2, and c-myc with those compounds was evaluated by the FRET (fluorescence resonance energy transfer) assay. These compounds showed less stabilizing ability compared to the ligand possessing two-way side chain of L2H2-(4M)6OTD (2d).G-quadruplex, located throughout the human genome, is one of the secondary structures of DNA consisting of G-quartets, which comprises a coplanar structure associated with four guanine residues forming Hoogsteen-type hydrogen bonds (Figure 1).1 Recently, G-quadruplex has been realized to play a critical role in the regulation of biological processes.2 Since G-quadruplex structure is conformationally flexible, an artificial stabilization of the G-quadruplex is recognized as one of the promising approaches for treatment of G-quadruplex related diseases. For example, telomeric G-quadruplex3 is known to inhibit telomerase,3h a specific enzyme in cancer cells, as well as dissociate the telomere related proteins including TRF2 and Pot1, and stabilization of the G-quadruplex results in the induction of certain cancer cell lines into apoptosis.4 Therefore, a number of small compounds for stabilizing G-quadruplex structures (G-quadruplex ligands)5 have been developed, and some of them show telomerase inhibitory activity and/or transcriptional inhibition of oncogenes (e.g., c-kit,6 bcl-27 and c-myc8) in vitro as well as in vivo. These molecules usually have aromatic rings of similar size to the G-quartet plane, and interact with it through π-π stacking. One of the representative G-quadruplex ligands in nature is telomestatin (1), which was reported by Seto and Shin-ya in 2001.9 Telomestatin (1) has a macrocyclic polyoxazole structure, and shows very potent telomeric G-quadruplex stabilization activity by binding the DNA with an end-stacking mode.10 Over the past decade, telomestatin (1) has widely been used for the discovery and elucidation of telomeric G-quadruplex functions.11 Recently, we have designed and synthesized macrocyclic hexaoxazoles of 6OTDs (6 oxazoles telomestatin derivatives; 2) as G-quadruplex ligands.12 The 6OTDs (2) have the same size and similar planarity with 1, and are supposed to interact with the G-quartet through π-π interaction with end-stack mode (Figure 1). The 6OTDs (2) also have two side chains with amine, guanidine, or hydroxyl related functional groups, and these side chains were also expected to contribute to the stabilization of G-quadruplex by interacting with the backbone of the DNA, specifically with phosphate (Figure 2). Indeed, L2H2-(4M)6OTD; 2d12d shows potent stabilization activity with G-quadruplex forming oligonucleotides (GFOs). With these results, we examined further structural development of the 2d; i.e., introduction of four-way side chains in the structure of 3 was planned. Since G-quadruplex structure consists of four backbones as shown in Figure 1, each of the four side chains in 3 was expected to interact with these backbones efficiently,13 and increment of the G-quadruplex stabilization activity was anticipated. In this paper, we describe the synthesis of 6OTD derivatives with four-way side chains of 3 and 4 bearing hydroxyethyl group on the oxazole at C9 and C21, and C6 and C18 positions, respectively (Figure 2). The stabilizing ability of the G-quadruplexes with these new ligands was examined by fluorescence resonance energy transfer (FRET) melting assays.
Syntheses of 6OTD derivatives 3 and 4 were described in Schemes 1-4. Firstly, trioxazoles 15 and 22, key synthetic intermediates for 3 and 4, were synthesized (Schemes 1 and 2). α-Amino-β-ketoester 515 was reduced with sodium borohydride to give alcohol, which was then subjected with 2,2-dimethoxypropane in the presence of p-toluenesulfonic acid to give ester 7. Hydrolysis of ester 7 with lithium hydroxide gave carboxylic acid 8 in 74% yield. Then, the carboxylic acid 8 was reacted with L-serine methylester (9) in the presence of N-methylmorpholine (NMM) and 4-(4,6-dimethoxy-1,3,5-triazin-2-yl)-4-methylmorpholinium chloride (DMT-MM)16 to give β-hydroxy amide 10, which was subsequently reacted with diethylaminosulfurtrifluoride (DAST)17 followed by treatment with bromotrichloromethane in the presence of 1,8-diazabicyclo[5.4.0]undec-7-ene (DBU)18 to give oxazole 11 in 91% yield from 10. Deprotection of both N,O-acetonide and the Boc group in 11 was employed with 4N HCl, and the resulting amine 12 was reacted with the carboxylic acid 1312d in the presence of NMM and DMT-MM to give β-hydroxyamide 14 in 98% yield from 12. Cyclodehydration of 14 with DAST followed by oxazole formation with bromotrichloromethane in the presence of DBU gave trioxazole 15 bearing methyl and hydroxyethyl group at C6 and C9, respectively, in 51% yield from 14. Synthesis of trioxazole 22 was also performed in a similar way with 15 from carboxylic acid 16 and amine 17 (Scheme 2). β-Hydroxyamide 18 was synthesized from carboxylic acid 16 and amine 17 in the presence of NMM and DMT-MM in 75% yield. Oxazole ring formation of 18 was carried out by treatment with DAST followed by bromotrichloromethane in the presence of DBU, and 19 was obtained in 71% yield. After hydrolysis of the ester of 19 with lithium hydroxide, the resulting carboxylic acid was reacted with amine 20 in the presence of NMM and DMT-MM, and oxazole ring was constructed from β-hydroxyamide 21 to give trioxazole 22 bearing methyl and hydroxyethyl group at C9 and C6 positions, respectively, in 51% yield.
Trioxazoles 15 and 22 in hand, synthesis of 3 and 4 was carried out. Methyl ester group in trioxazole 15 was hydrolyzed with lithium hydroxide to give carboxylic acid 23, which was subsequently reacted with amine 24, obtained from 15 by deprotection of Alloc group in the presence of Pd(PPh3)4 and morphorine, by using NMM and DMT-MM to give 25 in 50% yield from 15. Deprotection of the Alloc group in 25 with catalytic amount of Pd(PPh3)4 followed by hydrolysis of methyl ester with lithium hydroxide gave amino acid 26, which was further subjected to macrocyclization using diphenylphosphoryl azide (DPPA) and i-Pr2NEt in the presence of 4-dimethylaminopyridine (DMAP) under high dilution conditions (3 mM) in CH2Cl2-DMF (2:1) to give 27 in 31% yield from 25. Finally, deprotection of benzyl group and Boc group in 27 was conducted with hydrogenolysis in the presence of Pd(OH)2 followed by treatment with TFA to give 3 in 80% yield from 27 (Scheme 3). 6OTD derivative 4 was also synthesized from 29 and 30 with a similarity to 3 via 31, 32, and 33 (Scheme 4).19
The G-quadruplex stabilizing ability of 3 and 4 was examined by FRET melting assay,20 and these abilities were evaluated by comparison with 2d. In this assay, a ligand-induced stabilization of a folded G-quadruplex is evaluated by an incremental measurement of the melting temperature as ΔTm. The ΔTm values of the four kinds of GFOs (0.2 µM); i.e., telo21, kit22, bcl27, and myc22, in the presence of 2d, 3, 4 at a concentration of 2 µM are summarized in Table 1.21 The ΔTm value of telo21 in the presence of 2d, 3, 4 were 21.1, 18.1, 17.8 ºC, respectively, and 3 and 4 showed less potent G-quadruplex stabilizing activity than 2d. Similar tendencies with telo21 were obtained to the other GFOs of kit22, bcl27 and myc22 by these ligands (Table 1).22
As shown in these results, newly designed four-way type 6OTD derivatives did not show significant improvement of G-quadruplex stabilizing ability. Since the planarity of macrocyclic structure of 6OTDs, which is one of the important factor for interacting with GFOs, of 3 and 4 are the same with that of 2d, it is obvious that the newly introduced side chains in 3 and 4 have no effect on the interaction with G-quadruplex DNA backbone. Since the slight decline of ΔTm values of G-quadruplex stabilizing activity of 3 and 4 were observed, newly introduced hydroxyethyl group only affected via steric hindrance. A functional group for stronger interaction with phosphate should be required in the four-way type 6OTD derivatives for the improvement of stabilization of G-quadruplex structure.
In conclusion, we have designed new G-quadruplex ligands of 3 and 4 bearing four-way side chains with amine and hydroxyl groups based upon the interaction mode of G-quadruplex with 6OTDs, our previously reported two-way type of G-quadruplex ligands. Unfortunately, these new ligands did not show significant improvement of stabilizing ability with some G-quadruplex forming DNA sequences compared to 2d. This might be due to the lower interaction of phosphate with hydroxyl group than amine. Further structural development of four-way type 6OTD derivatives, focusing on the functional groups in the side chain, is in progress.
ACKNOWLEDGEMENTS
This work was supported in part by a Grant-in-Aid for Scientific Research (B) from JSPS (No. 23310158) and a grant under the Industrial Technology Research Grant Program (01A04006b) from the New Energy and Industrial Technology Development Organization (NEDO) of Japan. Dr. M. Tera and K. Iida are grateful for a JSPS Research Fellowship for Young Scientists.
References
1. For selected reviews see: (a) D. J. Patel, A. T. Phan, and V. Kuryavyi, Nucleic Acids Research, 2007, 35, 7429; CrossRef (b). S. Neidle and G. N. Parkinson, Biochimie, 2008, 90, 1184. CrossRef
2. For selected reviews see: (a) S. Balasubramanian, L. H. Hurley, and S. Neidle, Nat. Rev. Drug. Discov., 2011, 10, 261; CrossRef (b) S. Neidle, FEBS J., 2010, 277, 1118. CrossRef
3. Intramolecular telomeric G-quadruplex structures in the presence of sodium cation, see: (a) Y. Wang and D. J. Patel, Structure, 1993, 1, 263; CrossRef Intramolecular telomeric G-quadruplex structures in the presence of potassium cation, see: (b) Y. Xu, Y. Noguchi, and H. Sugiyama, Bioorg. Med. Chem., 2006, 14, 5584; CrossRef (c) A. Ambrus, D. Chen, J. Dai, T. Bialis, R. A. Jones, and D. Yang, Nucleic Acids Res., 2006, 34, 2723; CrossRef (d) K. N. Luu, A. T. Phan, V. Kuryavyi, L. Lacroix, and D. J. Patel, J. Am. Chem. Soc., 2006, 128, 9963; CrossRef (e) A. T. Phan, V. Kuryavyi, K. N. Luu, and D. J. Patel, Nucleic Acids Res., 2007, 35, 6517; CrossRef Crystal structure of intramolecular telomeric G-quadruplex structure in the presence of potassium cation, see: (f) G. N. Parkinson, M. P. Lee, and S. Neidle, Nature, 2002, 417, 876; CrossRef Intramolecular telomeric G-quadruplex structure in crowded solution, see: (g) B. Heddi and A. T. Phan, J. Am. Chem. Soc., 2011, 133, 9824; CrossRef Telomerase inhibition by G-quadruplex, see: (h) A. M. Zahler, J. R. Williamson, T. R. Cech, and D. M. Prescott, Nature, 1991, 350, 718. CrossRef
4. (a) H. Tahara, K. Shin-ya, H. Seimiya, H. Yamada, T. Tsuruo, and T. Ide, Oncogene, 2006, 25, 1955; CrossRef (b) D. Gomez, T. Wenner, B. Brassart, C. Douarre, M.-F. O’Donohue, V. E. Khoury, K. Shin-ya, H. Morjani, C. Trantesaux, and J. F. Riou, J. Biol. Chem., 2006, 281, 38721. CrossRef
5. For selected reviews see: (a) A. Arora, N. Kumar, T. Agarwal, and S. Maiti, FEBS J., 2010, 277, 1345; CrossRef (b) T. Ou, Y. Lu, J. Tan, Z. Huang, K.-Y. Wong, and L. Gu, ChemMedChem, 2008, 3, 690. CrossRef
6. G-quadruplex structure of c-kit promoter region, see: (a) S. Rankin, A. P. Reszka, J. Huppert, M. Zloh, G. N. Parkinson, A. K. Todd, S. Ladame, S. Balasubramanian, and S. Neidle, J. Am. Chem. Soc., 2005, 127, 10584; CrossRef For c-kit transcription, see: (b) M. Bejugam, S. Sewitz, P. S. Shirude, R. Rodriguez, R. Shahid, and S. Balasubramanian, J. Am. Chem. Soc., 2007, 129, 12926. CrossRef
7. G-quadruplex structure of bcl-2 promoter region, see: (a) J. Dai, T. S. Dexheimer, D. Chen, M. Carver, A. Ambrus, R. A. Jones, and D. Yang, J. Am. Chem. Soc., 2006, 128, 1096; CrossRef (b) For bcl-2 transcription, see: X.-D. Wang, T.-M. Ou, Y.-J. Lu, Z. Li, Z. Xu, C. Xi, J.-H. Tan, S.-L. Huang, L.-K. An, D. Li, L.-Q. Gu, and Z.-S. Huang, J. Med. Chem., 2010, 53, 4390. CrossRef
8. G-quadruplex structure of c-myc promoter region, see: (a) T. Simonsson, P. Pecinkal, and M. Kubista, Nucleic Acids Res., 1998, 26, 1167; CrossRef For c-myc transcription, see: (b) A. Siddiqui-Jain, C. L. Grand, D. J. Bearss, and L. H. Hurley, Proc. Natl. Acad. Sci. USA, 2002, 99, 11593. CrossRef
9. Isolation of telomestatin, see: (a) K. Shin-ya, K. Wierzba, K. Matsuo, T. Ohtani, Y. Yamada, K. Furihata, Y. Hayakawa, and H. Seto, J. Am. Chem. Soc., 2001, 123, 1262; CrossRef Total synthesis of telomestatin, see: (b) T. Doi, M. Yoshida, K. Shin-ya, and T. Takahashi, Org. Lett., 2006, 8, 4165; CrossRef (c) T. Doi, K. Shibata, M. Yoshida, M. Takagi, M. Tera, K. Nagasawa, K. Shin-ya, and T. Takahashi, Org. Biomol. Chem., 2011, 9, 387; CrossRef (d) J. Linder, T. P. Garner, H. E. L. Williams, M. S. Searle, and C. J. Moody, J. Am. Chem. Soc., 2011, 133, 1044. CrossRef
10. M.-Y. Kim, H. Vankayalapati, K. Shin-ya, K. Wierzba, and L. H. Hurley, J. Am. Chem. Soc., 2002, 124, 2098. CrossRef
11. Selected reports of telomestatin as G-quadruplex ligands, see: (a) F. Rosu, V. Gabelica, K. Shin-ya, and E. D. Pauw, Chem. Commun., 2003, 2702; CrossRef (b) E. M. Rezler, J. Seenisamy, S. Bashyam, M.-Y. Kim, E. White, W. D. Wilson, and L. H. Hurley, J. Am. Chem. Soc., 2005, 127, 9439; CrossRef (c) P. Shirude, E. Gillies, S. Ladame, F. Godde, K. Shin-Ya, I. Huc, and S. Balasubramanian, J. Am. Chem. Soc., 2007, 129, 11890. CrossRef
12. (a) M. Tera, Y. Sohtome, H. Ishizuka, T. Doi, M. Takagi, K. Shin-ya, and K. Nagasawa, Heterocycles, 2006, 69, 505; CrossRef (b) M. Tera, H. Ishizuka, M. Takagi, M. Suganuma, K. Shin-ya, and K. Nagasawa, Angew. Chem. Int. Ed., 2008, 47, 5557; CrossRef (c) T. Shalaby, A. O. von Bueren, M.-L. Hürlimann, G. Fiaschetti, D. Castelletti, M. Tera, K. Nagasawa, A. Arcaro, I. Jelesarov, K. Shin-ya, and M. Grotzer, Mol. Cancer. Ther., 2010, 9, 167; CrossRef (d) S. Majima, M. Tera, K. Iida, K. Shin-ya, and K. Nagasawa, Heterocycles, 2011, 82, 1345. CrossRef
13. Tetra-substituted naphthalene diimides have reported to show exceptional affinity for telomeric G-quadruplex DNA.14.
14. (a) F. Cuenca, O. Greciano, M. Gunaratnam, S. Haider, D. Munnur, R. Nanjunda, W. D. Wilson, and S. Neidle, Bioorg. Med. Chem. Lett., 2008, 18, 1668; CrossRef (b) G. N. Parkinson, F. Cuenca, and S. Neidle, J. Mol. Biol., 2008, 381, 1145. CrossRef
15. Á. Pintér and G. Haberhauer, Eur. J. Org. Chem., 2008, 2375. CrossRef
16. M. Kunishima, C. Kawachi, F. Iwasaki, K. Terao, and S. Tani, Tetrahedron Lett., 1999, 40, 5327. CrossRef
17. (a) G. Burrell, J. M. Evans, G. E. Jones, and G. Stemp, Tetrahedron Lett., 1990, 31, 3649; CrossRef (b) A. J. Phillips, Y. Uto, P. Wipf, M. J. Reno, and D. R. Williams, Org. Lett., 2000, 2, 1165. CrossRef
18. D. R. Williams, D. J. Lowder, Y. G. Gu, and D. A. Brooks, Tetrahedron Lett., 1997, 38, 33. CrossRef
19. Spectral data for 15: [α]25D -26.4 (c 1.0, CHCl3); 1H NMR (400 MHz, CD3Cl) δ 8.27 (s, 1H), 7.29-7.21 (m, 5H), 5.98-5.87 (m, 1H), 5.52-5.50 (m, 1H), 5.30 (d, J = 5.0 Hz, 1H), 5.20 (d, J = 5.0, Hz 1H), 4.95-4.94 (m, 1H), 4.58 (d, J = 4.8 Hz, 2H), 4.54 (s, 2H), 4.58-4.50 (m, 1H), 3.92 (s, 3H), 3.82 (t, J = 6.5 Hz, 2H), 3.53 (t, J = 6.5 Hz, 2H), 3.09 (br, 2H), 2.65 (s, 3H), 2.15-1.81 (m, 2H). 1.52-1.25 (m, 4H), 1.41 (s, 9H), 13C NMR (400 MHz, CDCl3) δ 162.8, 161.5, 156.4, 156.0, 155.7, 155.5, 155.1, 151.7, 150.8, 143.4, 137.9, 134.2, 132.5, 128.2, 127.5, 126.2, 124.6, 117.8, 79.1, 72.7, 67.0, 65.9, 52.1, 49.1, 40.0, 33.8, 29.5, 28.3, 26.6, 22.4, 11.7, HRMS (ESI, M+Na) calcd for C35H43N5O10Na 716.2908, found 716.2904. Spectral data for 22: [α]25D -33.9 (c 1.0, CHCl3); 1H NMR (400 MHz,CD3Cl) δ 8.28 (s, 1H), 7.29-7.21 (m, 5H), 5.98-5.87 (m, 1H), 5.52-5.50 (m, 1H), 5.30 (d, J = 5.0 Hz, 1H), 5.20 (d, J = 5.0 Hz 1H), 4.97-4.96 (m, 1H), 4.58 (d, J = 4.8 Hz, 2H), 4.53 (s, 2H), 4.58-4.50 (m, 1H), 3.93 (s, 3H), 3.83 (t, J = 6.5 Hz, 2H), 3.44 (t, J = 6.5 Hz, 2H), 3.05 (br, 2H), 2.77 (s, 3H), 2.16-1.81 (m, 2H). 1.54-1.29 (m, 4H)1.41 (s, 9H), 13C NMR (400 MHz, CDCl3) δ 163.0, 161.3, 156.4, 155.8, 155.5, 154.2, 151.4, 150.8, 143.2, 137.7, 133.9, 132.3, 128.0, 127.3, 127.2, 125.2, 125.0, 117.5, 78.7, 72.4, 66.8, 65.7, 52.0, 49.0, 40.0, 33.6, 29.2, 28.2, 26.4, 22.2, 11.7, HRMS (ESI, M+Na) calcd for C35H43N5O10Na 716.2908, found 716.2909. Spectral data for 25: [α]25D 0.8 (c 1.0, CHCl3); 1H NMR (500 MHz, CD3Cl) δ 8.26 (s, 2H), 7.48 (d, J = 9.2 Hz, 1H), 7.27-7.22 (m, 10H), 5.95-5.88 (m, 1H), 5.53-5.52 (m, 1H), 5.47-5.41 (m, 1H), 5.32 (d, J = 5.0 Hz, 1H), 5.21 (d, J = 5.0 Hz, 1H), 4.96-4.95 (m, 1H), 4.59 (d, J = 4.8 Hz, 2H), 4.54 (s, 4H), 4.59-4.53 (m, 2H), 3.93 (s, 3H), 3.90-3.86 (m, 4H), 3.54 -3.48 (m, 4H), 3.11 (br, 4H), 2.67 (s, 3H), 2.68 (s, 3H), 2.17-1.87 (m, 4H), 1.54-1.29 (m, 8H), 1.41 (s, 18H),13C NMR (500 MHz, CDCl3) δ 162.1, 161.5, 159.9, 156.5, 155.9, 155.7, 155.4, 151.7, 151.4, 151.0, 143.4, 141.2, 138.0, 137.8, 136.5, 134.2, 132.5, 128.3, 127.7, 127.5, 126.2, 124.7, 124.5, 117.9, 79.1, 72.9, 67.1, 65.9, 52.1, 49.2, 46.6, 40.2, 40.0, 38.0, 33.8, 29.7, 29.5, 28.4, 26.8, 22.4, 11.8, HRMS (ESI, M+Na) calcd for C65H78N10O17Na 1293.5444, found 1293.5431. Spectral data for 31: [α]25D -7.0 (c 1.0, CHCl3); 1H NMR (500 MHz,CD3Cl) δ 8.28 (s, 1H), 8.25 (s, 1H), 7.49 (d, J = 9.2 Hz, 1H), 7.30-7.21 (m, 10H), 5.98-5.87 (m, 1H), 5.50-5.49 (m, 1H), 5.47-5.42 (m, 1H), 5.31 (d, J = 5.0 Hz, 1H), 5.21 (d, J = 5.0 Hz, 1H), 4.97-4.96 (m, 1H), 4.58 (d, J = 4.8 Hz, 2H), 4.53 (s, 4H), 4.58-4.50 (m, 2H), 3.93 (s, 3H), 3.84 (t, J = 6.5 Hz, 4H), 3.46 (t, J = 6.5 Hz, 4H), 3.07 (br, 4H), 2.77 (s, 3H), 2.76 (s, 3H), 2.16-1.81 (m, 4H). 1.54-1.29 (m, 8H)1.41 (s, 18H), 13C NMR (500 MHz, CDCl3) δ 163.1, 162.5, 161.3, 159.8, 156.5, 155.9, 155.8, 155.5, 154.5, 154.3, 151.5, 150.6, 143.2, 140.9, 137.7, 136.3, 132.4, 128.1, 127.4, 127.3, 125.3, 125.2, 125.0, 117.6, 78.8, 72.5, 66.8, 65.7, 52.0, 49.0, 46.5, 40.0, 33.6, 33,4 29.2, 28.2, 26.4, 22.6, 22.2, 11.7, HRMS (ESI, M+Na) calcd for C65H78N10O17Na 1293.5444, found 1293.5446. Spectral data for 3: [α]25D 12.9 (c 0.3, CH3OH); 1H NMR (400 MHz, DMSO-d6) δ 8.89 (s, 2H), 8.26 (d, J = 7.2 Hz, 2H), 7.72 (br, 4H), 5.42-5.30 (m, 2H), 4.97 (t, J = 5.0 Hz, 2H), 3.88-3.67 (m, 4H), 3.28-3.21 (m, 4H), 2.75 (br, 10H), 2.11-1.85 (m, 4H), 1.70-0.70 (m, 8H); 13C NMR (400 MHz, DMSO-d6) δ 162.3, 158.9, 155.6, 154.4, 152.9, 151.0, 142.3, 135.8, 124.7, 124.4, 69.8, 58.7, 46.9, 33.6, 29.5, 26.6, 21.1, 11.5, HRMS (ESI, M+Na) calcd for C36H42N10O10Na 797.2983, found 797.2982. Spectral data for 4: [α]25D 48.3 (c 0.1, CH3OH); 1H NMR (300 MHz, DMSO-d6) δ 8.89 (m, 8H), 8.23 (d, J = 7.2 Hz, 2H), 7.71 (br, 4H), 5.43-5.32 (m, 2H), 5.00 (t, J = 5.5 Hz, 2H), 3.88-3.76 (m, 4H), 2.72 (br, 10H), 2.14-1.82 (m, 4H), 1.66-0.77 (m, 8H); 13C NMR (400 MHz, DMSO-d6) δ 162.1, 159.0, 155.8, 154.8, 152.9, 151.7, 142.4, 135.9, 125.5, 123.9, 69.9, 58.8, 47.1, 33.5, 29.6, 26.8, 20.1, 11.6, HRMS (ESI, M+H) calcd for C36H43N10O10 775.3164, found 775.3147.
20. (a) A. D. Cian, L. Guittat, M. Kaiser, B. Sacca, S. Amrane, A. Bourdoncle, P. Alberti, M. P. Teulade-Fichou, L. Lacroix, and J. L. Mergny, Methods, 2007, 42, 183; CrossRef (b) J. L. Mergny and J.-C. Maurizot, ChemBioChem, 2001, 2, 124. CrossRef
21. In FRET melting assays, four representative single stranded G-quadruplex forming oligonucleotides (GFOs), i.e., telo21 (telomere), kit22 (c-kit), bcl27 (bcl-2) and myc22 (c-myc) were used in the presence of potassium cation (60 mM cacodylate buffer, pH 7.0 containing 60 mM KCl). GFOs oligonucleotides used in this paper are as follows.
22. No significant interactions between the double-stranded DNA and 6OTDs are observed.