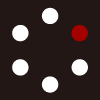
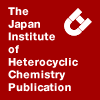
HETEROCYCLES
An International Journal for Reviews and Communications in Heterocyclic ChemistryWeb Edition ISSN: 1881-0942
Published online by The Japan Institute of Heterocyclic Chemistry
e-Journal
Full Text HTML
Received, 26th April, 2011, Accepted, 23rd May, 2011, Published online, 3rd June, 2011.
DOI: 10.3987/COM-11-S(P)14
■ Formal Synthesis of (-)-Clavepictine A and (+)-Clavepictine B from a Sulfinimine (N-Sulfinylimine)-Derived Chiral Building Block
Franklin A. Davis* and He Xu
Department of Chemistry, Temple University, 1901 N. 13th Street, Philadelphia, PA 19122, U.S.A.
Abstract
The sulfinimine-derived chiral building block trans-2,6-disubstituted 1,2,5,6-tetrahydropiperidine (+)-5 was employed in a concise formal asymmetric synthesis of the cytotoxic marine alkaloids clavepictines (-)-A and (+)-B (1a and 1b). This synthesis is highlighted by a highly diastereoselective hydroboration-oxidation reaction to install the C-3 hydroxyl group.Clavepictines A and B (1a and 1b) are quinolizidine alkaloids isolated from the marine Bermudan tunicate Clavelina picta.1,2 These alkaloids have an unusual cis-ring fused quinolizidine skeleton where the methyl and acetoxy (hydroxyl) groups occupy axial positions.2 Antifungal, antimicrobial, and antitumor activity have been attributed to these compounds.1 Couty and co-workers, in a structure-reactivity relationship (SAR) study concluded that their cytotoxicity is primarily determined by the side chain at C-10.3
Three asymmetric total syntheses of clavepictines A and B have been described.4-6 All of these syntheses utilized an intramolecular Michael-type cyclization of a functionalized piperidine to prepare the quinolizidine ring. In each case reduction of an intermediate acyl iminium ion species, generated from an N-acyl enamine was used to form the core trans-2,6-disubstituted piperidine ring. Momose and co-workers, in the first total synthesis of (+)-1a and (+)-1b, prepared the enamine from a lactone diol,4 while the Ma group used an enamine sulfone.5 Ha and Cha prepared the N-acyl enamine via a Comins’ cross-coupling of an enamide triflate.6 This group also generated the trans-2,6-disubstituted piperidine ring using Beak’s α-lithiation-substitution chemistry.
Recently we introduced a new method for the asymmetric synthesis of trans-2,6-disubstituted 1,2,5,6-tetrahydropiperidines such as (+)-5. These unsaturated heterocycles are valuable building blocks for the asymmetric synthesis of polysubstituted piperidines because of the many methods available for carbon-carbon double bond functionalization (Scheme 1).7,8 Our procedure involves a one-pot five-step cascade reaction of a sulfinimine-derived N-sulfinyl-δ-amino-β-ketophosphonate (+)-2 to a 3-phosphoryl dihydropyridone (+)-3 via an intermediate enaminone.7,9,10 Addition of methyl cuprate to (+)-3 gave the trans-piperidine (+)-4 which was transformed into 1,2,5,6-tetrahydropiperidine (+)-5. Compound (+)-5 was employed in the first total synthesis of (-)-myrtine (6) the unnatural isomer of the alkaloid (+)-myrtine. We describe here a formal synthesis of clavepictines A and B (1a and 1b) from building block (+)-5.
It was decided first to form the quinolizidine ring and introduce the C-3 hydroxy group later. The benzyl protecting group in (+)-5 was removed by treatment with sodium in liquid NH3 at -78 oC affording tetrahydropyridine alcohol (2S,6S)-(+)-7 in 90% yield (Scheme 2). Alcohol (+)-7 was oxidized with 1.5 equiv of Dess-Martin periodinane reagent to give an intermediate aldehyde 8, which was not purified, but was used directly in a Horner-Wadsworth-Emmons reaction with 1.1 equiv of diethyl phenylsulfonylmethylphosphonate11 and 1.1 equivalence of NaH, affording the piperidine vinyl sulfone (2S,6S)-(+)-9 in 66% yield over the two steps (Scheme 2). Attempts to remove the N-Boc group in (+)-9 with TMS-I were unsuccessful leading to unidenatified products. However, with excess TFA in CH2Cl2 (+)-9 gave a salt 10 that was treated with 10 equiv of Cs2CO3 in MeOH to give quinolizidine (+)-11 in 74% yield along with its minor isomer (Scheme 2). The intramolecular Michael cyclization gave the desired stereochemistry at C-10 as determined by X-ray crystallography as shown in Figure 2 and by its conversion to a compound of known absolute configuration (see below).
To install the C-3 hydroxyl group epoxidation of the double bond in (+)-11 was next considered. However, the basic nitrogen atom in (+)-11 and the anticipated problems in the regioselective ring opening of the epoxide led us to abandon this approach. In a study of the hydroboration of unsaturated piperidines Lyle and coworkers have reported that the direction of borane addition is strongly influenced by the positive charge on nitrogen; i.e. transition state TS-A should be favored over transition state TS-B (Scheme 3).12 Indeed hydroboration-oxidation of (+)-11 gave the desired alcohol (-)-12 in 50% isolated yield as a single isomer. Conversion of the alcohol into the MOM ether with methyl chloromethyl ether afforded (-)-13 in 82% yield (Scheme 3). Quinolizidine (-)-13 is identical in all respect to that prepared in Momose’ synthesis of the clavepictines.4 The synthesis of quinolizidine (-)-13 represents a formal asymmetric synthesis of clavepictines (-)-1a and (+)-1b and confirms the stereochemical assignments for (+)-11 and (-)-12.
In summary, a new formal synthesis of the cytotoxic marine alkaloids Clavepictines (-)-A and (+)-B (1a and 1b) is described from sulfinimine-derived building block trans-2,6-disubstituted 1,2,5,6-tetrahydropiperidins (+)-5. The synthesis is highlighted by a highly diastereoselective hydroboration-oxidation reaction to install the C-3 hydroxyl group.
ACKNOWLEDGEMENT
This work was supported by grants from the National Institutes of General Medical Sciences (GM57870 and GM51982).
EXPERIMENTAL
General procedure. All reagents were used as received unless otherwise noted. Tetrahydrofuran (THF), diethyl ether (Et2O), dichloromethane (DCM), and toluene were purified by filtration on a solvent purification system. Unless otherwise mentioned, all reagents were carried under argon atmosphere. Column chromatography was performed on silica gel, 230-400 mesh. TLC plates were visualized with UV, in an iodine chamber, or with phosphomolybdic acid, unless otherwise noted. 1H NMR and 13C NMR spectra were recorded on 500 and 400 MHz NMR spectrometers. (2S,6S)-(+)-tert-Butyl 6-(benzyloxy)butyl)-2-methyl-5,6-dihydropyridine-1-carboxylate (5) was prepared as previously described.7
(2S,6S)-(+)-tert-Butyl 6-(4-hydroxybutyl)-2-methyl-5,6-dihydropyridine-1 (2H)-carboxylate (7). In a 50-mL oven-dried three-neck round bottom flask equipped with a magnetic stirring bar, rubber septum, a cold finger, an ammonia gas inlet, and an argon inlet was placed Na metal (0.06 g, 2.56 mmol). The flask was cooled to −78 oC as the liquid ammonia (10 mL) was collected using the cold finger and reacted with Na to give a dark blue color solution. To the flask was slowly added t-BuOH (0.1 g in 1 mL THF) followed by (+)-5 (0.115 g, 0.32 mmol) in THF (6 mL). The solution was stirred at −78 oC for 10 min. The dry ice bath was removed and the solution was warmed to rt to evaporate the liquid ammonia before it was quenched with sat. aqueous NH4Cl (5 mL). The aqueous layer was extracted with EtOAc (2 × 10 mL). The combined organic phases were washed with H2O (2 × 5 mL), brine (5 mL), dried (MgSO4), and concentrated. Flash chromatography (EtOAc:hexanes:MeOH, 10:90:10) afforded 0.086 g (90%) of a colorless oil; [α]20D +50.8 (c 0.85, CHCl3); IR (neat) 3410, 2213, 1700, 1232, 1169 cm-1; 1H NMR (CDCl3) δ 5.73 (m, 2 H), 4.14 (m, 1 H), 3.98 (m, 1 H), 3.62 (d, J = 6.4 Hz, 2 H), 2.28 (m, 1 H), 2.09 (m, 1 H), 1.61-1.22 (m, 6 H), 1.47 (s, 9 H), 1.27 (d, J = 6.4 Hz, 3 H); 13C NMR (CDCl3) δ 155.9, 132.0, 122.6, 79.7, 63.2, 51.9, 48.6, 33.4, 32.9, 28.9, 27.2, 23.6, 21.9. HRMS calcd for C15H28NO3 (M + H) 270.2069. Found 270.2068.
(2S,6S)-(+)-tert-Butyl 2-methyl-6-(5-(phenylsulfonyl)pent-4-enyl)-5,6-dihydropyridine-1-carboxylate(9). In a 25-mL, one-necked, round-bottom flask fitted with magnetic stirring bar, rubber septum, and argon inlet was placed (+)-7 (0.067 g, 0.249 mmol) in CH2Cl2 (8 mL). To the flask was added Dess-Martin periodinane (1.06 mL, 15% in CH2Cl2) and the solution was stirred for 2 h. The reaction mixture was quenched with aqueous Na2SO3 (5 mL) and stirred for 10 min until the solution was clear. The mixture was then extracted with Et2O (3 × 10 mL) and the combined organic phases were washed with sat. aqueous NaHCO3 (2 × 5 mL), brine (5 mL), dried (MgSO4), and concentrated to give a yellow residue of the aldehyde 8. The crude aldehyde was used in the next step without purification. In another 25-mL one-necked, round-bottom flask fitted with magnetic stirring bar, rubber septum, and argon inlet was placed NaH (0.01 g, 0.249 mmol, 60% in mineral oil), which was washed with petroleum ether (3 × 5 mL). To this flask was added (EtO)2POCH2SO2Ph11 (0.073 g, 0.249 mmol) in THF (10 mL) followed by the crude aldehyde. The mixture was stirred for 30 min and quenched with sat. aqueous NH4Cl solution (5 mL). The phases were separated, the aqueous phase was extracted with EtOAc (2 × 10 mL) and the combined organic phasse were washed with H2O (5 mL), brine (5 mL), dried (MgSO4), and concentrated. Flash chromatography (EtOAc:hexanes, 20:80) afforded 0.077 g (76%) of a colorless oil; [α]20D +45.7 (c 1.5, CHCl3); IR (neat) 2360, 1684, 1365, 1147 cm-1; 1H NMR (CDCl3) δ 7.86 (m, 1 H), 7.84 (m, 1 H), 7.59 (m, 1 H), 7.52 (m, 2 H), 6.96 (m, 1 H), 6.30 (m, 1 H), 5.71 (m, 2 H), 4.09 (m, 1 H), 3.98 (m, 1 H), 2.31 (m, 1 H), 2.23 (m, 2 H), 1.50-1.38 (m, 4 H), 1.44 (s, 9 H), 1.26 (d, J = 6.4 Hz, 3 H); 13C NMR (CDCl3) δ 155.8, 147.1, 141.1, 133.5, 132.1, 131.0, 129.6, 127.9, 122.4, 79.8, 33.4, 31.7, 28.9, 27.3, 25.5, 22.0. HRMS calcd for C22H31NO4NaS (M + Na) 428.1872. Found 428.1859.
(4S,6S,9aS)-(+)-4-Methyl-6-(phenylsulfonylmethyl)-4,6,7,8,9,9a-hexahydro-1H-quinolizine (11). In a 25-mL, one-necked, round-bottom flask fitted with magnetic stirring bar, rubber septum, and argon inlet was placed (+)-9 (0.31 g, 7.64 mmol) in CH2Cl2 (20 mL). To the flask was added TFA (2.84 mL, 38.2 mmol) and the solution was stirred for 50 min. The solvent was removed and the flask was placed under vacuum for 1 h to afford a yellow residue. The residue was dissolved in MeOH (30 mL), Cs2CO3 (2.5 g, 76.4 mmol) was added and the reaction mixture was stirred for 30 min. At this time, the mixture was transferred to a pre-mixed solvent of EtOAc (150 mL) and brine (30 mL) in a 500-mL separatory funnel and shaken vigorously for 1 min. The organic phase was washed with brine (2 × 20 mL), dried (MgSO4), and concentrated. Flash chromatography (EtOAc:hexanes, 50:50) afforded 0.17 g (74%) of a white solid; mp 69-72 oC; [α]20D + 51.2 (c 0.45, CHCl3); IR (neat) 2360, 1303, 1142 cm-1; 1H NMR (CDCl3) δ 7.93 (d, J = 7.6 Hz, 2 H), 7.61 (d, J = 7.2 Hz, 1 H), 7.53 (d, J = 7.2 Hz, 2 H), 5.49 (m, 1 H), 5.36 (m, 1 H), 3.58 (m, 2 H), 3.30 (q, J = 8.0 Hz, 2 H), 2.81 (m, 1 H), 1.86 (m, 2 H), 1.67 (m, 2 H), 1.54 (m, 2 H), 1.36 (m, 1 H), 1.13 (m, 1 H), 1.00 (d, J = 6.4 Hz, 3 H); 13C NMR (CDCl3) δ 140.8, 133.6, 130.4, 129.3, 128.5, 121.8, 59.4, 50.2, 49.0, 48.7, 29.8, 25.8, 23.8, 21.2, 20.8; HRMS calcd for C17H24NO2S (M + H) 306.1528, found 306.1516.
(3R,4S,6S,9aS)-(-)-4-Methyl-6-(phenylsulfonylmethyl)-octahydro-1H-quinolizin-3-ol (12). In a 25-mL, one-necked, round-bottom flask fitted with magnetic stirring bar, rubber septum, and argon inlet was placed (+)-11 (0.030 g, 0.0982 mmol) in THF (5 mL) at 0 oC. To the flask was added BH3·THF (0.393 mL, 0.393 mmol, 1 M in THF) and the solution warmed to rt and stirred for 2 h. At this time, the rubber septum was removed and replaced with a reflux condenser, and 6 N NaOH (0.5 mL) and 30% H2O2 (0.1 mL) were added to the solution. The mixture was heated at 50-55 oC for 2 h, cooled to rt, diluted with H2O (2 mL), and extracted with EtOAc (3 × 10 mL). The combined organic phases were washed with H2O (5 mL), brine (5 mL), dried (MgSO4), and concentrated. Flash chromatography (EtOAc:hexanes, 50:50) afforded 0.016 g (50%) of a colorless oil; [α]20D -12.5 (c 0.21, CHCl3); IR (neat) 3520, 1311, 1149 cm-1; 1H NMR (CDCl3) δ 7.92 (d, J = 7.6 Hz, 2 H), 7.63 (t, J = 7.2 Hz, 1 H), 7.54 (t, J = 7.2 Hz, 2 H), 3.83 (m, 1 H), 3.70 (m, 1 H), 3.27 (dd, J = 14.0, 5.2 Hz, 1 H), 3.02 (m, 1 H), 2.71 (m, 2 H), 2.10-0.76 (m, 13 H); 13C NMR (CDCl3) δ 140.8, 133.7, 129.4, 128.5, 73.1, 58.4, 56.0, 50.1, 49.4, 28.6, 27.9, 24.2, 23.0, 20.5, 15.4. HRMS calcd for C17H26NO3S (M + H) 324.1633. Found 324.1644.
(3R,4S,6S,9aS)-(-)-3-(Methoxymethoxy)-4-methyl-6-(phenylsulfonyl-methyl)-octahydro-1H-quinolizine(13). In a 25-mL, one-necked, round-bottom flask fitted with magnetic stirring bar, rubber septum, and argon inlet was placed (-)-12 (0.020 g, 0.0618 mmol) in THF (5 mL). To the flask was added chloromethyl methyl ether (0.019 mL, 0.247 mmol) and DIPEA (0.043 mL, 0.247 mmol). The solution was stirred for 8 h and the solvent was concentrated to give a residue. Flash chromatography (EtOAc:hexanes, 50:50) afforded 0.017 g (82%) of a colorless oil; [α]20D -11.0 (c 0.30, CHCl3), [lit.4 [α]26D -10.95 (c 0.81, CHCl3)]; IR (neat) 3058, 1446, 1303, 1148 cm-1; 1H NMR (CDCl3) δ 7.92 (d, J = 7.6 Hz, 2 H), 7.61 (t, J = 7.6 Hz, 1 H), 7.54 (t, J = 7.6 Hz, 2 H), 4.70 (d, J = 6.8 Hz, 1 H), 4.58(d, J = 6.8 Hz, 1 H), 3.83 (m, 1 H), 3.64 (dd, J = 14.0, 6.4 Hz, 1 H), 3.36 (s, 3 H), 3.28 (dd, J = 14.4, 5.2 Hz, 1 H), 2.79 (m, 2 H), 2.62 (m, 1 H), 1.84-1.66 (m, 4 H), 1.55 (m, 1 H), 1.37-1.19 (m, 3 H), 1.05 (d, J = 5.6 Hz, 3 H), 1.02-0.93 (m, 2 H); 13C NMR (CDCl3) δ 140.6, 131.1, 128.8, 128.1, 95.6, 79.4, 58.2, 55.5, 53.1, 49.2, 48.9, 27.7, 25.8, 22.3, 21.0, 20.4, 15.4. HRMS calcd for C19H30NO4S (M + H) 368.1896. Found 368.1881. The spectra were consistent with literature values.4
References
1. M. F. Raub, J. H. Cardellina, M. I. Choudhary, C.-Z. Ni, J. Clardy, and M. C. Alley, J. Am. Chem. Soc., 1991, 113, 3178. CrossRef
2. F. Kong and D. J. Faulkner, Tetrahedron Lett., 1991, 32, 3667. CrossRef
3. C. Agami, F. Couty, G. Evano, F. Darro, and R. Kiss, Eur. J. Org. Chem., 2003, 2062. CrossRef
4. N. Toyooka, Y. Yotsui, Y. Yoshida, and T. Momose, J. Org. Chem., 1996, 61, 4882. CrossRef
5. S. Yu, X. Pu, T. Cheng, R. Wang, and D. Ma, Org. Lett., 2006, 8, 3179. CrossRef
6. (a) J. D. Ha, D. Lee, and J. K. Cha, J. Org. Chem., 1997, 62, 4550; CrossRef (b) J. D. Ha and J. K. Cha, J. Am. Chem. Soc., 1999, 121, 10012. CrossRef
7. F. A. Davis, H. Xu, and J. Zhang, J. Org. Chem., 2007, 72, 2046. CrossRef
8. For reviews see: (a) P. M. Weintraub, J. S. Sabol, J. M. Kane, and D. R. Borcherding, Tetrahedron, 2003, 59, 2953; CrossRef (b) S. Laschat and T. Dickner, Synthesis, 2000, 1781; CrossRef (c) M. S. M. Pearson, M. Mathe-Allainmat, V. Fargeas, and J. Lebreton, Eur. J. Org. Chem., 2005, 2159; CrossRef (d) F.-X. Felpin and J. Lebreton, Current Organic Synthesis, 2004, 1, 83; CrossRef (e) M. G. P. Buffat, Tetrahedron, 2004, 60, 1701. CrossRef
9. For leading references to the chemistry of N-sulfinylimines see: F. A. Davis, J. Org. Chem., 2006, 71, 8993. CrossRef
10. For reviews on N-sulfinylimines see: (a) D. Morton and R. A. Stockman, Tetrahedron, 2006, 62, 8869; CrossRef (b) C. H. Senanayake, D. Krishnamurthy, Z.-H. Lu, Z. Han, and I. Gallou, Aldrichimica Acta, 2005, 38, 93; (c) P. Zhou, B.-C. Chen, and F. A. Davis, Tetrahedron, 2004, 60, 8003. CrossRef
11. T. A. Blumenkopf, Synth. Commun., 1986, 16, 139. CrossRef
12. R. E. Lyle, R. R. Carle, C. R. Ellefson, and C. K. Spicer, J. Org. Chem., 1970, 35, 802. CrossRef