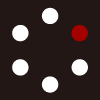
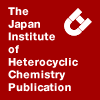
HETEROCYCLES
An International Journal for Reviews and Communications in Heterocyclic ChemistryWeb Edition ISSN: 1881-0942
Published online by The Japan Institute of Heterocyclic Chemistry
e-Journal
Full Text HTML
Received, 30th June, 2011, Accepted, 10th August, 2011, Published online, 18th August, 2011.
DOI: 10.3987/COM-11-S(P)65
■ Development of an Intramolecular Gassman’s [2+2] Cycloaddition
John B. Feltenberger, Changhong Ko, Jun Deng, Sunil K. Ghosh, and Richard P. Hsung*
Department of Chemistry, Division of Pharmaceutical Sciences, University of Wisconsin, 777 Highland Aenue, Madison, WI 53705-2222, U.S.A.
Abstract
The development of an intramolecular variant of Gassman’s cationic [2 + 2] cycloaddition is described herein. Mechanistic aspects of the stepwise nature of this cycloaddition process between vinyl acetals and unactivated olefins have been studied. We have also explored the scope of this reaction with regard to various oxygen-, nitrogen-, and carbon-tethered acetals to provide access to a diverse array of bicyclic scaffolds. Additionally, we have identified vinyl hemiaminals as having favorable reactivity for cationic [2 + 2] cycloaddition.INTRODUCTION
In the past half century, a diverse array of elegant work from the synthetic community has established the [2 + 2] cycloaddition, alongside with the Diels-Alder cycloaddition, as one of the most powerful cycloaddition manifolds in organic synthesis.1 With the Woodward-Hoffman rules as the fundamental guiding principle,2 photochemical [2 + 2] cycloadditions3-8 have naturally taken center stage and played a significant role in propelling this cycloaddition to its current prominence in synthesis.
Most notably, as shown in Scheme 1, [2 + 2] cycloadditions provide (1) an excellent entry to cyclobutanes [1→2], although regiochemical control remains a challenge intermolecularly, (2) a facile assembly of structural complexity via intramolecular cycloadditions with excellent regiochemical control [3→4], and (3) an innovative strategy inspired by natural products TaxolTM and ingenol for accessing medium-sized rings via a 2-carbon ring expansion that takes advantage of the ring strain of cyclobutanes [5→6].4
The concerted thermal [2 + 2] cycloaddition of alkenes is disallowed by orbital symmetry considerations as dictated by the Woodward-Hoffmann rules.2 However, several elegant advances have been made in the area of non-photochemical [2 + 2] cycloadditions,9 with most being stepwise transformations mediated by metals or Lewis acids.10,11 In many cases, these thermally driven [2 + 2] cycloadditions employ strained or pyramidalized olefins that can serve as activated 2π-components9 (collectively, 7a, Scheme 2, left box). Alternatively, the [2 + 2] cycloadditions of alkynes 7b,12 ketenes 7c, or allenes 7d13 may progress thermally via a formal six-electron [2π + 2π + 2π] process due to the presence of orthogonally positioned π-orbitals, thereby bypassing the frontier orbital restrictions (right box).14
In order for the thermal [2 + 2] cycloaddition of unstrained alkenes to take place, it must proceed through a stepwise, non-concerted mechanism. This has primarily been achieved using electronically matched donor and acceptor alkenes, described in Scheme 3 below. Several elegant approaches using silyl enol ethers with activated α,β-unsatured carbonyl components have been described, generally with high yields and diastereoselectivies.15 In 2007, Corey and Canales reported a highly enantioselective [2 + 2] cycloaddition of silyl enol ethers 8 with trifluoroethyl acrylate ester 9 using a chiral Lewis acid catalyst, to afford cyclobutanes 10 in up to 98% ee.16 However, in rare cases the acid promoter can generate a highly electrophilic species which may undergo [2 + 2] cycloaddition of unactivated olefins. Ishihara and co-workers reported the use of a chiral organoammonium salt to promote the enantioselective [2 + 2] cycloaddition of unactivated alkenes 11 with α-acyloxyacroleins 12, affording cyclobutanes 13 with up to 95% ee.17
In this context, what has remained unexplored is Gassman's cationic [2 + 2] cycloaddition18,19 through the activation of vinyl acetals 14 [Scheme 4]. This is intriguing because employing vinyl oxocarbenium ions 17 [complexed] or 18 [non-complexed] as highly reactive 2π-components via activation of vinyl acetals for inter- and intramolecular [4 + 2] cycloadditions [see 19 and 20, respectively] have been extensively investigated.20 In addition, although not shown here, utilizing vinyl oxocarbenium ions as a three-carbon component in oxyallyl [4 + 3] cycloadditions,21,22 and generating oxocarbenium ions from cyclic acetals for Mukaiyama-type aldol or Prins-type cyclizations23,24 are well documented. However, an intramolecular variant of Gassman's cationic [2 + 2] cycloaddition was not known25 until our efforts.
We perceived an invaluable opportunity to develop a useful thermally driven intramolecular [2 + 2] cycloaddition because (a) the cationic [2 + 2] cycloaddition pathway has a biosynthetic origin [Scheme 5];26 (b) it provides an excellent protocol for accessing biologically relevant cyclobutane containing natural products;27 and (c) thermally driven reactions generally possess advantages over their photochemical counterparts in terms of reaction scales, operations, functional group tolerances, and competing pathways.
RESULTS AND DISCUSSION
1. Preliminary Investigations of the Intramolecular Gassman’s [2 + 2] Cycloaddition.
Our exploration into the feasibility of an intramolecular Gassman’s [2 + 2] cycloaddition commenced with the facile construction of tethered vinyl acetal 22 [Scheme 6]. Alkylation of cis-2-butene-1,4-diol with 5-bromo-2-methyl-2-pentene, followed by PCC oxidation afforded enal trans-21 in 37% yield over two steps. Utilizing a mild protocol developed by Noyori,28 subjection of trans-21 with 1,2-bis(trimethylsiloxy)ethane and catalytic TMSOTf produced the desired vinyl acetal 22 in good yield.
With tethered vinyl acetal 22 in hand, we were poised to investigate our system with BF3·OEt2, which was successfully employed by Gassman in his intermolecular reactions.19 Upon treatment of acetal 22 to a stoichiometric amount of BF3·OEt2 in CH2Cl2 at 0 °C (Table 1, entry 1), we observed rapid consumption of the starting material, succeeding in the formation of cyclobutane 23 in 40% yield in 10 min, along with the presence of hydrolysis side-products. This key result validated the feasibility of an intramolecular Gassman’s [2 + 2] cycloaddition for the construction of bicyclic cyclobutane scaffolds. Other solvents screened were ineffective for the cycloaddition reaction, with full or partial recovery of acetal 22 (entries 2 and 3). It was determined that addition of 4 Å MS was critical for reducing the competing hydrolysis of the acetal moiety of both 22 and 23, thereby increasing the yield of desired cyclobutane 23 to 66% (entry 4). We noted that increasing the concentration from 0.005 M to 0.01 M (entry 5) proved to be detrimental to the yield of this reaction, and no attempts were made at higher concentrations. Further attempts to optimize the reaction conditions by changing the temperature and loading of BF3·OEt2 failed to improve yields (entries 6-9). These discouraging results led us to investigate other acids to facilitate the [2 + 2] cycloaddition.
As summarized below in Table 2, we have identified several other Lewis and Brønsted acids that are effective for the cationic [2 + 2] cycloaddition reaction. Of the Lewis acids tested, SnCl4 (entries 3-6) appears to perform comparably with BF3·OEt2, and we have found SnCl4 to be a more general acid with other substrates investigated (vide infra). Of note, there emerged a distinct temperature dependence, with no reaction observed at -78 °C over 2 h (entry 4), while at -20 °C the reaction completed in 10 min (entries 3 and 5). Of the Brønsted acids investigated, we found that Tf2NH29,30 afforded cyclobutane 23 in suitable yield (entry 9), while TFA was only moderately useful (entry 13). TfOH, another acid that was successfully employed by Gassman,18 was poor for our intramolecular cycloaddition (entry 10), with appreciable amounts of mono-cyclized product 24 observed (vide infra).
Upon finding several suitable conditions for the [2 + 2] cycloaddition of vinyl acetal 22, it was necessary to determine the relative stereochemistry of cycloadduct 23. It was found in all cases for the [2 + 2] cycloaddition that the cyclobutane product was isolated as a single diastereomer. We pursued confirmation of the stereochemistry via X-ray analysis after conversion of 23 into para-bromobenzoyl ester 25 as shown in Scheme 7. This transformation was facilitated first by hydrolysis of cyclobutane acetal 23 into its corresponding aldehyde, followed by NaBH4 reduction to the free alcohol and finally acylation to afford 25 in 70% overall yield. This structural confirmation shows the cis-fused ring junction of the cyclobutane, as well as the formation of the second carbon-carbon bond with preference for the acetal positioned on the convex face of the bicycle.
2. Mechanistic Studies.
During the course of our acid screen for the intramolecular cationic [2 + 2] cycloaddition, we observed in some cases the existence of mono-cyclized products (Table 2, entries 1 and 10). To account for these side products, we propose the following mechanistic pathway shown in Scheme 8. Upon activation of the vinyl acetal 22 with acid, the tethered olefin reacts with vinyl oxocarbenium 26 to form the first carbon-carbon bond, an enol ether, and a stabilized tertiary cation shown as 27. Trapping of the carbocation 27 with the enol ether represents the desired bond forming process to afford cyclobutane 23 (vide supra). An alternative pathway exists, represented by E1-elimination (arrows in 27) to afford alkenes 29 and 24 in a cationic ene or vinylogous-Prins manner (29 has not been observed – if formed, it could rapidly isomerize to 24 under the acidic reaction conditions). Also plausible is the formation of alkene 24 via proton abstraction through an external proton scavenger or sponge.
To better understand the stepwise nature of the cationic [2 + 2] cycloaddition reaction, we constructed cis- and trans-acetals 30 and cis- and trans-vinyl acetals 32 and subjected each set to identical reaction conditions (Scheme 9). Treatment of both cis- and trans-30 with SnCl4 led to cyclobutane 31 as a single diastereomer in good to high yields. Likewise, cis- and trans-32 led to cyclobutane 33, again isolated as a single diastereomer (relative stereochemistry was determined by nOe analysis). These results are consistent with a stepwise mechanism, whereupon activation of the vinyl acetal with an acid (shown as vinyl oxocarbenium A), the initial double bond geometry of either acetal 30 or olefin 32 is readily scrambled and lost through carbocation intermediate B, and the resulting cyclobutane is formed with a high degree of conformational control.
Recognition that an alternative pathway may account for the convergence of products, we initiated an isomerization study of both the cis- and trans-vinyl acetals 30. Namely, we were looking for interconversion of cis-30 into trans-30 or vice versa, prior to the cycloaddition event, which could then account for the isolation of single cycloaddition product 31. As shown in Table 3, upon treatment of pure trans-30 with 1.0 equivalent of SnCl4, the reaction was quenched with 3.0 equivalents of pyridine after 5 seconds (entry 1), and it was found that there was very little conversion to product with no isomerization observed. Increasing the reaction time past 5 seconds, we observed completeness of reaction at 1 minute, and even 20 seconds (entries 2 and 3), affording complete conversion to cyclobutane product 31 without a trace of isomerization to cis-30 detected.31 The cis isomer reacts more slowly, with only about 30% conversion to product after 20 seconds (entry 5), with no trans-30 observed.
Our findings shown in Table 3 were supported by the follow up study with cis- and trans- cinnamate derived acetals 32 exhibiting a similar result. Subjection of cis-32 to 1.0 equiv SnCl4 led to conversion to cyclobutane 33 in 10 seconds, with no starting material or trans-32 observed (Table 4, entry 1). Quenching the same reaction after 5 seconds, we found incomplete reaction, with recovery of cis-32 in addition to cycloadduct 33 (entry 3). In each case studied, we saw no evidence of interconversion into the opposite isomer prior to cycloaddition, providing evidence for the scrambling of olefin geometry after the first carbon-carbon bond has been formed.
3. Expanding the Scope of Intramolecular [2 + 2] Cycloadditions.
To showcase the utility of an intramolecular Gassman [2 + 2] cycloaddition for the construction of cyclobutane frameworks, we decided to pursue a more diverse set of vinyl acetals, varying in tether length and composition. We are particularly interested in nitrogen- and carbon-tethered systems, for their potential importance in natural product synthesis.
A. Oxygen-Tethered Vinyl Acetals
We decided to first examine the effect of the ring-size of the acetal. Toward this goal, the vinyl acetal containing 1,3-dioxane 34 was prepared from vinyl aldehyde 21 using Noyori’s acetalization conditions. Vinyl acetal 34 was subjected to the optimized conditions employing SnCl4 as a Lewis acid, giving cycloadduct 35 in 50% yield (Scheme 10). Therefore, the ring-size of the acetal has little impact on the outcome of the cycloaddition as the yield from 34 was comparable to that of 22 bearing a 1,3-dioxolane. Additionally, we explored placing oxygen at the homoallylic position with respect to the vinyl acetal moiety. Treatment of 36 with our standard Lewis acidic conditions afforded cycloadduct 37 in 45% yield, exhibiting similar reactivity to vinyl acetal 22.
B. Nitrogen-Tethered Vinyl Acetals
We chose to pursue sulfonamide- and carbamate-tethered vinyl acetals due to the relative ease of installation as well as removal of these protecting groups. As shown in Scheme 11, sulfonamide-tethered vinyl acetal 41 can be readily accessed starting with Mitsunobu reaction of sulfonamide 38 and THP-protected cis-2-butene-1,2-diol 39, followed by hydrolysis of THP using p-TsOH in MeOH and then PCC oxidation to afford vinyl aldehyde 40 in 29% yield over 3 steps. Upon treatment of aldehyde 40 to Noyori’s acetalization protocol, vinyl acetal 41 was afforded in 48% yield.
We were quickly met with a challenging [2 + 2] cycloaddition of vinyl acetal 41, as shown in Scheme 12. After some screening, optimal conditions were found utilizing 1.0 equivalent of SnCl4 to produce desired cyclobutane 42 in a modest 25% yield. It is noteworthy that mono-cyclized product 43 was isolated in 13% yield, which could arise from intermolecular trapping of the carbocation intermediate with chloride released from the Lewis acid. We also investigated carbamate-tethered acetals 44 and 46, which afforded desired cycloadducts 45 and 47, respectively, in slightly higher yields. To date, we have been unable to identify a more optimal set of conditions to enact the desired cycloaddition for this substrate.
C. Carbon-Tethered Vinyl Acetals
To render this cationic [2 + 2] cycloaddition useful toward natural product synthesis,27,32 we examined vinyl acetals 51 and 55 with an all-carbon tether (Scheme 13). Vinyl acetal 51 was prepared starting from diethyl malonate 48, which was converted to THP-protected alcohol 49 by mono-prenylation followed by allylation with known allyl iodide.33 Deprotection of the THP group by acidic hydrolysis conditions followed by PCC oxidation with double bond isomerization afforded vinyl aldehyde 50 in good yield. Acetalization using Noyori’s conditions gave the cycloaddition precursor 51. To prepare the vinyl acetal 55, aldehyde 53 was prepared in three steps from 52. Aldehyde 53 was converted to enal 54 by treatment with formylmethylenetriphenylphosphorane. Noyori’s acetalization of vinyl aldehyde 54 afforded vinyl acetal 55 in good yield.
Anticipating that the gem-diester tethered vinyl acetal 51 may undergo [2 + 2] cycloaddition with the assistance of a favorable Thorpe-Ingold effect,34 we subjected it to our standard conditions (Scheme 14). While we did find cycloadduct 56 in modest yield, we also isolated a proportional amount of mono-cycle 57. As with the nitrogen tethered examples, we have been unable to identify a set of conditions as to optimize this reaction further.
Initial attempts at intramolecular [2 + 2] cycloaddition with vinyl acetal 55 using our standard conditions with acids BF3·OEt2 or SnCl4 showed that these acids were ineffective at promoting this cycloaddition on a substrate possessing an all-carbon tether. We turned our attention to an iron (III) chloride catalyst adsorbed on silica gel described by Chavan and co-workers as an effective Lewis acid catalyst for ionic Diels-Alder reactions.35 It is noted here that iron (III) chloride is a known as a Lewis acid as well as an oxidant. In a related study, an iron (III) perchlorate salt adsorbed on alumina support was used as a single-electron oxidant to promote the [2 + 2] cycloaddition of styrene derivitives via cation radical pathway.11a
Further screening revealed 0.5 equiv of FeCl3 adsorbed on silica gel (5% w/w)35 to be the optimal conditions to give the desired cyclobutane 58 in 64% yield (Scheme 15). We note here that this Lewis acid was the first we had found to be optimally active at sub-stoichiometric loadings. Seeking to better understand the nature of the active catalytic species, reactions performed with SiO2 or 0.1 equiv of FeCl3 alone failed to afford the desired product, which suggests that the FeCl3 adsorbed on silica gel has an attenuated reactivity profile. Furthermore, treatment of trans-30 with catalytic loadings of FeCl3-SiO2 (5% w/w) afforded cyclobutane 31 in yields comparable to those seen with SnCl4.
4. Pursuit of a Diastereoselective Cationic [2 + 2] Cycloaddition.
Having demonstrated the feasibility of an intramolecular Gassman [2 + 2] cycloaddition, we were interested in establishing a diastereoselective variant of this reaction involving C2-symmetric chiral cyclic acetals as shown in 59 [Scheme 16]. An important element in rendering this approach feasible is that vinyl oxocarbenium ions such as 60 have been proposed to still be complexed [see the arrow in 60] to the oxygen atom that is coordinated to a given Lewis acid. This complexation23,24 can provide both the rigidity and facial bias necessary for the olefin to approach the vinyl oxocarbenium ion in 60 selectively.
In addition, by using the concepts developed from the aldol chemistry involving chiral acetals derived from C2-symmetric 1,3-dioxanes,24 the Lewis acid would prefer to coordinate to the oxygen atom adjacent to the RAX substituent in vinyl oxocarbenium ions 61 to avoid the gauche interaction with REQ. In addition, the approach of the incoming nucleophile [olefin in this case] should stereoelectronically prefer to be anti-periplanar to the leaving C-O bond. Based on this analysis, cycloadditions through vinyl oxocarbenium ions 61 can be highly stereoselective.
To explore a potentially diastereoselective [2 + 2] cycloaddition, we have assembled a handful of chiral C2-symmetric vinyl acetals [Scheme 17] which were subjected to our cationic cycloaddition protocol. Investigating chiral 1,3-dioxolane acetal 62 derived from from (S,S)-2,3-butanediol treated with 1.0 equiv SnCl4 afforded only a modest yield of cyclobutane 63 with a dr of 2:1(determined by 13C NMR), without assignment of the absolute stereochemistry of the major diastereomer.36 Increasing the tether length as shown in acetal 66 improves the yield to 40%, with the dr remaining at 2:1. Changing the acetal moiety to chiral 1,3-dioxane 68 again affords only modest dr of 2:1. While these stereoselectivities are disappointing, they are in actuality not entirely surprising. The transference of stereochemical information from the chiral acetal to the β-position of the vinyl oxocarbenium for the first C-C bond formation represents an example of remote 1,5-stereoinduction, which is also observed in half-cycle adducts 64 and 65.
5. Activation of Hemiaminal for Gassman’s [2 + 2] Cycloaddition.
We decided to explore other modes of activation for Gassman’s [2 + 2] such as employing vinyl hemiaminals 70 for the cycloaddition. Meyers and co-workers have disclosed the thermal [2 + 2] cycloaddition of vinyl hemiaminals; however, this reaction does not proceed via Gassman-type activation, and affords the opposite regiochemistry of such.37 What remains undeveloped is use of these vinyl hemiaminals in a Gassman-type cationic process. While this mode of activation could lead to both enol ethers 71 and enamines 72 after the 1st bond formation depending on whether the oxygen or nitrogen atom is activated by the Lewis acid, enamines 72 should be much more nucleophilic than enol ethers in general, thereby enhancing the 2nd bond formation and diminishing the competing E1-elimination pathway that was observed with acetals (vide supra). The stereochemical outcome in 73 could be intriguing, although the information at the hemiaminal center is lost during the reaction. What may play a significant role is the electronics of the R substituent in facilitating this 2nd bond formation in 72.
We first studied hemiaminal 74, which was synthesized in good yield from a trans-acetalization between N-toluenesulfonyl ethanolamine and acrolein diethyl acetal.38 As shown in Table 5, the reaction of 74 with 3.0 equiv tetramethylethylene 75 and TiCl4 (entry 1) afforded 76 exclusively in 29% yield, which can occur via sequential 1,2-methyl and hydrogen shifts after the first C-C bond formation. Using SnCl4, which was an effective acid in our intramolecular [2 + 2] cycloaddition of acetals also afforded this undesired rearrangement product in 61% yield (entry 2). Gratifyingly, BF3·OEt2 afforded cyclobutane 77 in good yield, with some side product 76 still observable. Other acids screened also afforded 77 (entries 4-6), but upon treatment of hemiaminal 74 with 1.0 equiv triflimide (entry 7), we obtained nearly quantitative amounts of desired cyclobutane 77. This result represents the highest yielding Gassman-type [2 + 2] cycloaddition reported to date.
Having found the optimal acid for the reaction of hemiaminal 74 with tetramethylethylene, we decided to explore the ability to run this reaction catalytically using triflimide. It was found that the reaction proceeds efficiently and in high yield, even with triflimide loadings of 1 mol% (Table 6, entry 5). Interestingly, the reaction yield is nearly the same whether using 50 mol% down to 1 mol% of triflimide used (entries 2-5). Also of note, the dr of the reaction increased modestly from 3:1 to 5:1 when decreasing the amount of acid used.
Having demonstrated the achievability of using hemiaminals for Gassman’s [2 + 2] cycloaddition in an intermolecular fashion, recently, we have begun investigation of an intramolecular hemiaminal [2 + 2] cycloaddition. We constructed the cinnamate-tethered hemiaminal 80 analogous to acetal trans-32 from a transacetalization of diethyl acetal 79.32,33 Treatment of hemiaminal 80 with 1.0 equiv of triflimide afforded desired cyclobutane 81, albeit the isolated yield is poor. We are currently optimizing this reaction further, and exploring other tethered hemiaminal substrates for this intramolecular cationic [2 + 2] cycloaddition.
CONCLUSION
We reported here our efforts toward establishing an intramolecular Gassman’s [2 + 2] cycloaddition of alkene-tethered vinyl acetals. Our mechanistic studies have validated a stepwise cationic mechanism for this cycloaddition. A wide range of functionalities within the tether have been shown to be tolerated, which increases the usefullness of this reaction for the synthesis of natural products. While chiral acetals have been investigated, they afford only modest diastereoselectivities. We have also shown that an intermolecular Gassman’s [2 + 2] cycloaddition with hemiaminals is feasible, affording cyclobutanes in high yields, and are currently investigating an intramolecular variant of this process. We are currently pursuing an enantioselective version of Gassman’s intramolecular cationic [2 + 2] cycloaddition with chiral Brønsted and Lewis acids.
EXPERIMENTAL
All reactions were performed in flame-dried glassware under a nitrogen atmosphere. Solvents were distilled prior to use. Reagents were used as purchased (Aldrich, Acros), except where noted. Chromatographic separations were performed using Bodman 60 Å SiO2. 1H and 13C NMR spectra were obtained on Varian VI-300, VI-400, and VI-500 spectrometers using CDCl3 (except where noted) with TMS or residual CHCl3 in the solvent as standard. Melting points were determined using a Laboratory Devices MEL-TEMP and are uncorrected/calibrated. Infrared spectra were obtained using NaCl plates on a Bruker Equinox 55/S FT–IR Spectrophotometer, and relative intensities are expressed qualitatively as s (strong), m (medium), and w (weak). TLC analysis was performed using Aldrich 254 nm polyester-backed plates (60 Å, 250 µm) and visualized using UV and a suitable chemical stain. Low-resolution mass spectra were obtained using an Agilent-1100-HPLC/MSD and can be either APCI or ESI, or an IonSpec HiRes-MALDI FT-Mass Spectrometer. High-resolution mass spectral analyses were performed at University of Wisconsin Mass Spectrometry Laboratories. All spectral data obtained for new compounds are reported. X-Ray analyses were performed at the X-Ray facility in University of Minnesota.
Synthesis of Aldehyde 21.
Allylic Alcohol S1. To a solution of NaH (1.58 g, 39.6 mmol) in THF (80 mL) and DMF (20 mL) was added 2-butene-1,4-diol (4.65 g, 52.9 mmol) at 0 oC. The mixture was stirred for 10 min at 0 oC before 5-bromo-2-methyl-2-pentene (3.70 mL, 26.4 mmol) was added. The mixture was warmed to rt and stirred for 24 h, and the progress of the reaction was monitored by TLC. Upon disappearance of the starting material, the reaction was quenched with sat aq NH4Cl and extracted with EtOAc (3 × 100 mL). The combined organic layers were washed with water (2 × 100 mL) and sat aq NaCl (100 mL), dried over Na2SO4, and concentrated in vacuo. Further purification was performed by silica gel flash column chromatography [gradient eluent: 6:1 to 2:1 hexane/EtOAc] to afford allylic alcohol S1 (2.20 g, 50%) as colorless oil. S1: Rf = 0.30 [33% EtOAc in hexanes]; 1H NMR (400 MHz, CDCl3) d 1.63 (d, J = 1.2 Hz, 3H), 1.70 (d, J = 1.6 Hz, 3H), 2.03 (brs, 1H), 2.29 (dt, J = 7.2 and 7.2 Hz, 2H), 3.43 (t, J = 7.2 Hz, 2H), 4.06 (brd, J = 6.4 Hz, 2H), 4.20 (brd, J = 6.0 Hz, 2H), 5.12 (ttt, J = 1.2, 1.6, and 7.2 Hz, 1H), 5.65-5.75 (m, 1H), 5.79-5.85 (m, 1H).
General Procedure 1: For the PCC Oxidation to Vinyl Aldehyde.
Aldehyde 21. To a suspension of PCC (1.23 g, 5.60 mmol) in anhyd CH2Cl2 (20 mL) was added the above allylic alcohol S1 (636.0 mg, 3.70 mmol) at 0 oC. The solution was warmed to rt and stirred for 4 h, and the progress of the reaction was monitored using TLC. Upon completion, the reaction mixture was diluted with diethyl ether and filtered through a pad of CeliteTM. The filtrate was concentrated in vacuo and purified by silica gel flash column chromatography [gradient eluent: 10:1 to 4:1 hexane/EtOAc] to afford vinyl aldehyde 21 (570.0 mg, 74%) as yellow oil.
21: Rf = 0.28 [20% EtOAc in hexanes]; 1H NMR (500 MHz, CDCl3) δ 1.62 (s, 3H), 1.69 (s, 3H), 2.30 (q, J = 7.0 Hz, 2H), 3.46 (t, J = 7.0 Hz, 2H), 4.24 (dd, J = 6.0 and 7.5 Hz, 2H), 5.10-5.13 (m, 1H), 6.36 (dddd, J = 2.0, 2.0, 4.0, and 15.5 Hz, 1H), 6.83 (dt, J = 4.0 and 16.0 Hz, 1H), 9.57 (d, J = 8.0 Hz, 1H); 13C NMR (125 MHz, CDCl3) δ 17.7, 25.7, 28.6, 69.3, 71.1, 119.9, 131.6, 133.9, 153.4, 193.2; IR (neat) cm-1 1116s, 1692s, 2861m, 2971m; mass spectrum (APCI): m/e (% relative intensity) 169 (M+H)+ (100).
General Procedure 2: For the Preparation of Vinyl Acetal.
Vinyl Acetal 22. To a solution of vinyl aldehyde 21 (220.0 mg, 1.30 mmol) and 1,2-bis(trimethylsilyloxy)ethane (405.0 mg, 1.90 mmol) in anhyd CH2Cl2 (1 mL) was added TMSOTf (2.90 mg, 0.013 mmol) at -78 oC. The reaction mixture was stirred for 4 h under nitrogen atmosphere, and the progress of the reaction was monitored using TLC. Upon completion, the reaction was quenched with pyridine, poured into a sat aq NaHCO3 (5 mL), and extracted with diethyl ether (3 × 10 mL). The combined organic layers were washed with sat aq NaCl (10 mL), dried over Na2SO4, and concentrated in vacuo. Further purification was performed by silica gel flash column chromatography [gradient eluent: 6:1 to 2:1 hexane/EtOAc] to afford vinyl acetal 22 (231.0 mg, 84%) as colorless oil.
22: Rf = 0.29 [20% EtOAc in hexanes]; 1H NMR (500 MHz, CDCl3) δ 1.62 (s, 3H), 1.70 (s, 3H), 2.28 (q, J = 7.5 Hz, 2H), 3.41 (t, J = 7.5 Hz, 2H), 3.89-3.92 (m, 2H), 3.99-4.03 (m, 4H), 5.09-5.12 (m, 1H), 5.28 (d, J = 6.0 Hz, 2H), 5.69-5.74 (m, 1H), 5.99 (ddd, J = 5.0, 5.0, and 15.0 Hz, 1H), 13C NMR (125 MHz, CDCl3) δ 17.7, 25.6, 28.5, 28.6, 64.9, 69.9, 70.2, 103.2, 120.2, 127.9, 133.0, 133.5; IR (neat): cm-1 2967s, 2882s, 2858s; mass spectrum (ESI): m/e (% relative intensity) 235.1 (M+Na)+ (100); HRMS-ESI m/e calcd for C12H20NaO3 [M+Na]+ 235.1310, found 235.1303.
General Procedure 3: For Intramolecular [2 + 2] Cycloaddition.
Cyclobutane 23. To a solution of vinyl acetal 22 (23.0 mg, 0.11 mmol) and 4Å molecular sieves (100 mg) in anhyd CH2Cl2 (20 mL) was added 1.0 M SnCl4 (0.10 mL, 0.11 mmol) at -20 oC. The reaction mixture was stirred for 10 min under nitrogen atmosphere at the same temperature, and the progress of the reaction was monitored using TLC. Upon completion, the reaction was quenched via addition of pyridine until the yellow color of reaction mixture turned colorless. The resulting reaction mixture was poured into sat aq NaHCO3 (10 mL), and extracted with CH2Cl2 (3 × 10 mL). The combined organic layers were washed with sat aq NaCl (10 mL), dried over Na2SO4, and concentrated in vacuo. Further purification was performed by silica gel flash column chromatography [gradient eluent: 6:1 to 2:1 hexane/EtOAc] to afford 17 (14.0 mg, 60%) as colorless oil.
23: Rf = 0.20 [20% EtOAc in hexanes]; 1H NMR (500 MHz, CDCl3) δ 1.03 (s, 3H), 1.16 (s, 3H), 1.57-1.60 (m, 1H), 1.90-1.95 (m, 2H), 2.11-2.16 (m, 1H), 2.23-2.27 (m, 1H), 3.04-3.09 (m, 1H), 3.53 (dd, J = 4.0, and 12.0 Hz, 1H), 3.76-3.93 (m, 6H), 4.84 (d, J = 7.5 Hz, 1H); 13C NMR (125 MHz, CDCl3) δ 23.7, 24.0, 24.3, 29.4, 36.4, 37.6, 45.3, 64.5, 64.6, 64.9, 68.2, 105.5; IR (neat): cm-1 1146m, 1698w, 2839m, 2952s; mass spectrum (APCI): m/e (% relative intensity) 213 (M+H)+ (100), 151 (10); HRMS-ESI m/e calcd for C12H20NaO3 235.1310, found 235.1310.
24: Rf = 0.22 [20% EtOAc in hexanes]; 1H NMR (400 MHz, CDCl3) δ 1.65 (s, 3H), 1.69 (s, 3H), 1.90 (ddd, J = 6.4, 6.8, and 14.4 Hz, 1H), 1.98 (ddd, J = 4.0, 8.4, and 14.4 Hz, 1H), 2.20-2.28 (m, 1H), 2.36 (ddd, J = 1.2, 2.0, and 14.4 Hz, 1H), 2.77-2.80 (m, 1H), 3.27 (ddd, J = 3.2, 10.8, and 12.4 Hz, 1H), 3.41 (dd, J = 3.2 and 11.2 Hz, 1H), 3.79-4.00 (m, 6H), 4.77 (dd, J = 4.0, and 6.8 Hz, 1H); 13C NMR (100 MHz, CDCl3) δ 19.9, 20.1, 26.9, 35.2, 35.4, 64.9, 65.0, 69.3, 72.6, 104.0, 124.2, 128.7; IR (neat): cm-1 948s, 1128s, 1140s 2882m, 2957m; mass spectrum (APCI): m/e (% relative intensity) 213 (M+H)+ (100), 183 (50), 151 (35).
Synthesis of Ester 25.
Aldehyde S2. To a solution of cyclobutane 23 (25.0 mg, 0.12 mmol) in THF (5 mL) was added 10% aq HCl (1.5 mL) at 0 oC. The reaction mixture was warmed to rt and stirred for 2 h. The progress of the reaction was monitored using TLC. Upon completion, the reaction was quenched with aq NaHCO3 (5 mL), and extracted with diethyl ether (3 × 10 mL). The combined organic layers were washed with sat aq NaCl (10 mL), dried over Na2SO4, and concentrated in vacuo. Further purification was performed by silica gel flash column chromatography [gradient eluent: 6:1 to 2:1 hexane/EtOAc] to afford aldehyde S2 (231.0 mg, 84%) as colorless oil.
S2: Rf = 0.23 [20% EtOAc in hexanes]; 1H NMR (500 MHz, CDCl3) δ 1.16 (s, 3H), 1.22 (s, 3H), 1.63-1.68 (m, 1H), 1.92-1.97 (m, 2H), 2.61-2.65 (m, 1H), 3.07-3.12 (m, 2H), 3.59 (dd, J = 4.5 and 12.5 Hz, 1H), 3.73 (d, J = 12.5 Hz, 1H), 3.85-3.89 (m, 1H), 9.58 (d, J = 5.0 Hz, 1H); 13C NMR (125 MHz, CDCl3) δ 23.9, 24.1, 24.5, 27.4, 37.5, 40.8, 54.5, 64.8, 68.1, 203.3; mass spectrum (ESI): m/e (% relative intensity) 191.1 (M+Na)+(100); HRMS-ESI m/e calcd for C10H16NaO2 191.1048, found 191.1051.
Alcohol S3. To a solution of aldehyde S2 (10.0 mg, 0.060 mmol) in EtOH (3 mL) was added NaBH4 (2.20 mg, 0.060 mmol) at 0 oC. The reaction mixture was stirred for 1 h at the same temperature, and the progress of the reaction was monitored using TLC. Upon completion, the reaction was quenched by 1N aq HCl. The resulting reaction mixture was extracted with CH2Cl2 (3 × 5 mL). The combined organic layers were washed with sat aq NaCl (10 mL), dried over Na2SO4, and concentrated in vacuo. Further purification was performed by silica gel flash column chromatography [gradient eluent: 2:1 to 1:1 hexane/EtOAc] to afford alcohol S3 (9.80 mg, 97%) as colorless oil.
S3: Rf = 0.25 [50% EtOAc in hexanes]; 1H NMR (500 MHz, CDCl3) δ 1.05 (s, 3H), 1.10 (s, 3H), 1.57-1.61 (m, 1H), 1.88-1.97 (m, 3H), 2.33-2.38 (m, 1H), 3.07-3.10 (m, 1H), 3.53-3.58 (m, 2H), 3.64-3.68 (m, 1H), 3.80 (d, J = 12.5 Hz, 1H), 3.84-3.87 (m, 1H); 13C NMR (125 MHz, CDCl3) δ 23.1, 24.1, 24.5, 31.3, 36.7, 37.3, 45.1, 63.1, 65.1, 68.3; IR (neat): cm-1 3408s, 2954s, 2922s, 2850m; mass spectrum (ESI): m/e (% relative intensity) 193.1 (M+Na)+ (100); HRMS-ESI m/e calcd for C10H18NaO2 193.1204, found 193.1200.
Ester 25. To a solution of alcohol S3 (16.0 mg, 0.093 mmol), Et3N (0.12 mL, 0.093 mmol), and DMAP (3.00 mg, 0.025 mmol) in anhyd CH2Cl2 (5 mL) were added 4-bromobenzoyl chloride (2.90 mg, 0.013 mmol) at 0 oC. The reaction mixture was warmed to rt and stirred for 12 h. The progress of the reaction was monitored using TLC. Upon completion, the reaction was quenched with H2O and extracted with CH2Cl2 (3 × 10 mL). The combined organic layers were washed with sat aq NaCl (10 mL), dried over Na2SO4, and concentrated in vacuo. Further purification was performed by silica gel flash column chromatography [gradient eluent: 6:1 to 2:1 hexane/EtOAc] to afford 25 (34.0 mg, 95%) as a white solid.
25: Rf = 0.80 [50% EtOAc in hexanes]; 1H NMR (500 MHz, CDCl3) δ 1.00 (s, 3H), 1.08 (s, 3H), 1.52-1.58 (m, 1H), 1.86-2.01 (m, 3H), 2.56 (ddd, J = 7.0, 7.0, amd 14.5 Hz, 1H), 3.00-3.05 (m, 1H), 3.50 (dd, J = 4.0 and 12.0 Hz, 1H), 3.75 (d, J =12.0 Hz, 1H), 3.80-3.82 (m, 1H), 4.21-4.22 (m, 2H), 7.49-7.51 (m, 2H), 7.78-7.80 (m, 2H); 13C NMR (125 MHz, CDCl3) δ 23.3, 23.9, 24.2, 31.5, 36.8, 37.5, 41.5, 64.9, 65.2, 68.0, 128.0, 129.2, 131.0, 131.1, 131.7, 131.8, 165.8; IR (neat): cm-1 2960m, 2926m, 2850m, 1718s; mass spectrum (ESI): m/e (% relative intensity) 375.2 (M+Na)+ (100); HRMS-ESI m/e calcd for C17H21BrNaO3 375.0572, found 375.0570.
Synthesis of Vinyl Acetals Trans-30 and Cis-30.
Allylic Alcohol S4. To a solution of NaH (1.30 g, 32.6 mmol) in THF (100 mL) was added 2-butene-1,4-diol (2.68 mL, 32.6 mmol) at 0 oC. The mixture was stirred for 10 min at 0 oC before 4-bromo-2-methyl-2-butene (2.00 mL, 16.3 mmol) was added. The mixture was warmed to rt and stirred for 24 h, and the progress of the reaction was monitored by TLC. Upon disappearance of the starting material, the reaction was quenched with sat aq NH4Cl and extracted with EtOAc (3 × 100 mL). The combined organic layers were washed with sat aq NaCl (100 mL), dried over Na2SO4, and concentrated in vacuo. Further purification was performed by silica gel flash column chromatography [gradient eluent: 2:1 to 1:1 hexane/EtOAc] to afford allylic alcohol S4 (3.90 g, 84%) as colorless oil. S4: Rf = 0.30 [33% EtOAc in hexanes]; 1H NMR (400 MHz, CDCl3) δ 1.68 (d, J = 1.2 Hz, 3H), 1.75 (d, J = 1.6 Hz, 3H), 2.15 (brs, 1H), 4.00 (d, J = 6.8 Hz, 2H), 4.04 (ddt, J = 0.8, 1.6, and 6.0 Hz, 2H), 4.20 (dd, J = 5.6 and 6.0 Hz, 2H), 5.35 (ttt, J = 1.2, 1.6, and 6.8 Hz, 1H), 5.73 (ddddd, J = 1.2, 1.6, 5.2, 6.0, and 11.6 Hz, 1H), 5.81 (ddddd, J = 1.2, 1.6, 5.2, 6.0, and 11.6 Hz, 1H).
Vinyl Aldehyde Trans-S5. – Prepared according to general procedure 1 to afford vinyl aldehyde trans-S5 (2.50 g, 69%) as colorless oil from allylic alcohol S4.
Trans-S5: Rf = 0.42 [25% EtOAc in hexanes]; 1H NMR (400 MHz, CDCl3) δ 1.69 (d, J = 1.2 Hz, 3H), 1.77 (d, J = 1.6 Hz, 3H), 4.04 (d, J = 7.2 Hz, 2H), 4.24 (dd, J = 2.0 and 4.4 Hz, 2H), 5.36 (ttt, J = 1.2, 1.6, and 7.2 Hz, 1H), 6.35 (ddt, J = 2.0, 8.0, and 16.0 Hz, 1H), 6.86 (dt, J = 4.4 and 16.0 Hz, 1H), 9.58 (d, J = 8.0 Hz, 1H); 13C NMR (100 MHz, CDCl3) δ 18.3, 26.0, 67.6, 68.6, 120.5, 132.0, 138.2, 153.8, 193.5; IR (neat) cm-1 2980w, 2880w, 1723s, 1293s, 906s; mass spectrum (APCI): m/e (% relative intensity) 155 (M+H)+ (100).
Vinyl Aldehyde Cis-S5. To a solution of Dess-Martin periodinane (3.00 g, 7.0 mmol) in CH2Cl2 (50 mL) was allyl alcohol S4 (1.00 g, 6.40 mmol) in CH2Cl2 (50 mL) at rt. The mixture was stirred for 2 h and the progress of the reaction was monitored using TLC. Upon disappearance of the starting material, the reaction was quenched with sat aq NaHCO3 and sat aq Na2S2O3, and extracted with CH2Cl2 (3 × 50 mL). The combined organic layers were washed with sat aq NaCl (50 mL), dried over Na2SO4, and concentrated in vacuo. Further purification was performed by silica gel flash column chromatography [gradient eluent: 10:1 to 4:1 hexane/EtOAc] to afford cis-S5 (746.0 mg, 76%) as colorless oil.
Cis-S5: Rf = 0.32 [33% EtOAc in hexanes]; 1H NMR (400 MHz, CDCl3) δ 1.69 (d, J = 0.8 Hz, 3H), 1.69 (d, J = 1.2 Hz, 3H), 4.04 (d, J = 7.2 Hz, 2H), 4.48 (dd, J = 1.6 and 5.2 Hz, 2H), 5.36 (ttt, J = 0.8, 1.2, and 7.2 Hz, 1H), 6.05 (dddd, J = 1.6, 2.0, 6.8, and 11.6 Hz, 1H), 6.44 (ddd, J = 5.2, 6.0, and 11.6 Hz, 1H), 10.08 (d, J = 6.8 Hz, 1H); 13C NMR (100 MHz, CDCl3) δ 18.3, 26.0, 66.8, 67.6, 120.5, 129.9, 138.4, 148.3, 191.7; IR (neat) cm-1 2985w, 2875w, 1710s, 1290s, 925s; mass spectrum (APCI): m/e (% relative intensity) 155 (M+H)+ (100)
Vinyl Acetal Trans-30. – Prepared according to general procedure 2 to afford vinyl acetal trans-30 (480.0 mg, 84%) as colorless oil.
Trans-30: Rf = 0.30 [20% EtOAc in hexanes]; 1H NMR (500 MHz, CDCl3) δ 1.66 (s, 3H), 1.74 (s, 3H), 3.88-3.93 (m, 2H), 3.95-4.01 (m, 6H), 5.28 (d, J = 10.5 Hz, 1H), 5.32-5.35 (m, 1H), 5.68-5.72 (m, 1H), 5.98 (dt, J = 5.5 and 15.5 Hz, 1H); 13C NMR (125 MHz, CDCl3) δ 18.0, 25.7, 64.9, 66.7, 69.2, 103.2, 120.8, 128.2, 132.9, 137.2; IR (neat): cm-1 2970m, 2878s, 1685w, 1448w; mass spectrum (ESI): m/e 221.2 (M+Na)+ (100); HRMS-ESI m/e calcd for C11H18NaO3 221.1154, found 221.1155.
Vinyl Acetal Cis-30. – Prepared according to general procedure 2 to afford vinyl acetal cis-30 in 67% yield as a colorless oil.
Cis-30: Rf = 0.29 [20% EtOAc in hexanes]; 1H NMR (400 MHz, CDCl3) δ 1.68 (s, 3H), 1.75 (s, 3H), 3.87-3.91 (m, 2H), 3.97 (d, J = 7.2 Hz, 1H), 3.99-4.03 (m, 2H), 4.15 (dd, J = 1.6 and 6.4 Hz, 1H), 5.35 (ttt, J = 1.2, 1.6, and 7.2 Hz, 1H), 5.54 (dd, J = 0.8 and 6.8 Hz, 1H), 5.59 (dddd, J = 1.6, 1.6, 6.8, and 11.2 Hz, 1H), 5.89 (dddd, J = 0.8, 5.6, 6.0, and 11.2 Hz, 1H); 13C NMR (100 MHz, CDCl3) δ 18.2, 26.0, 65.1, 65.8, 66.9, 99.3, 121.0, 128.3, 133.9, 137.6; IR (neat): cm-1 3011w, 2882w, 1074s, 957s; mass spectrum (APCI): m/e (% relative intensity) 199 (M+H)+ (85), 169 (30), 137 (100), 113 (15).
Cyclobutane 31. – Prepared according to general procedure 3 to afford cyclobutane 31 (33.0 mg, 76%) as a colorless oil from trans-30. Also prepared according to general procedure 3 in 50% yield from cis-30.
31: Rf = 0.27 [20% EtOAc in hexanes]; 1H NMR (400 MHz, CDCl3) δ 1.00 (s, 3H), 1.18 (s, 3H), 1.76 (dd, J = 7.2 and 7.6 Hz, 1H), 2.33 (dd, J = 6.8 and 7.2 Hz, 1H), 2.78 (ddd, J = 4.4, 7.2, and 7.6 Hz, 1H), 3.33 (dd, J = 4.4 and 9.2 Hz, 1H), 3.38 (dd, J = 6.8 and 10.4 Hz, 1H), 3.77 (d, J = 9.2 Hz, 1H), 3.80-3.86 (m, 2H), 3.88-3.95 (m, 2H), 4.05 (d, J = 10.4 Hz, 1H), 4.95 (dd, J = 7.2 Hz, 1H); 13C NMR (125 MHz, CDCl3) δ 24.4, 25.6, 34.3, 35.9, 47.2, 49.6, 64.7, 65.0, 69.2, 72.5, 102.2; IR (neat): cm-1 2958m, 2866m, 1138m, 1073s, 990s; mass spectrum (APCI): m/e (% relative intensity) 199 (M+H)+ (100), 137 (20), 101 (35).
Synthesis of Vinyl Acetal Trans-32 and Cis-32.
Allylic Alcohol Trans-S6. To a solution of NaH (0.47 g, 19.6 mmol) in THF (25 mL) was added 2-butene-1,4-diol (1.73 g, 19.6 mmol) at 0 oC. The mixture was stirred for 1 h at 0 oC before adding a solution of trans-cinnamyl bromide (1.93 mL, 9.80 mmol) in THF (5 mL) dropwise. The mixture was warmed to rt and stirred for 24 h, and the progress of the reaction was monitored using TLC. Upon disappearance of the starting material, the reaction was quenched with sat aq NH4Cl and extracted with EtOAc (3 × 20 mL). The combined organic layers were washed with water (2 × 20 mL) and sat aq NaCl (20 mL), dried over Na2SO4, and concentrated under reduced pressure. Further purification was performed by silica gel flash column chromatography [2:1 hexane/EtOAc] to afford allylic alcohol trans-S6 (1.36 g, 68%) as colorless oil. Trans-S6: Rf = 0.28 [33% EtOAc in hexanes]; 1H NMR (400 MHz, CDCl3) δ 1.78 (t, J = 6.0 Hz, 1H), 4.13 (dd, J = 2.0 and 6.0 Hz, 2H), 4.18 (dd, J = 1.2 and 6.0 Hz, 2H), 4.24 (ddd, J = 1.6, 6.0, and 6.0 Hz, 2H), 5.76 (dtt, J = 1.6, 6.0, and 11.2 Hz, 1H), 5.85 (dtt, J = 1.6, 6.0, and 11.2 Hz, 1H), 6.29 (dt, J = 6.0 and 16.0 Hz, 1H), 6.62 (dd, J = 1.6 and 16.0 Hz, 1H), 7.22-7.45 (m, 5H).
Allylic alcohol cis-S6 was prepared from cis-cinnamyl bromide in the same manner in 43% yield.
Cis-S6: Rf = 0.30 [33% EtOAc in hexanes]; 1H NMR (400 MHz, CDCl3) δ 1.67 (brs, 1H), 4.07 (dd, J = 1.2 and 6.0 Hz, 2H), 4.18 (dd, J = 1.2 and 6.4 Hz, 2H), 4.28 (dd, J = 2.0 and 6.4 Hz, 2H), 5.71 (dtt, J = 1.2, 6.0, and 11.2 Hz, 1H), 5.81 (dtt, J = 1.2, 6.4, and 11.2 Hz, 1H), 5.85 (dt, J = 6.4 and 11.6 Hz), 6.63 (dt, J = 2.0 and 11.6 Hz, 1H), 7.18-7.38 (m, 5H).
Vinyl Aldehyde trans-78. – Prepared according to general procedure 1 to afford vinyl aldehyde trans-78 (1.71 g, 78%) as a yellow oil.
Trans-78: Rf = 0.11 [10% EtOAc in hexanes]; 1H NMR (400 MHz, CDCl3) δ 4.24 (dd, J = 1.6 and 6.0 Hz, 2H), 4.32 (dd, J = 2.0 and 4.0 Hz, 2H), 6.29 (dt, J = 6.0 and 16.0 Hz, 1H), 6.4 (ddt, J = 2.0, 8.0, and 16.0 Hz, 1H), 6.64 (dt, J = 1.6 and 16 Hz, 1H), 6.87 (dt, J = 4.0 and 16.0 Hz, 1H) 7.24-7.42 (m, 5H), 9.61 (d, J = 8.0 Hz, 1H); 13C NMR (100 MHz, CDCl3) δ 68.7, 71.8, 125.3, 126.8, 128.2, 128.8, 132.1, 133.4, 136.6, 153.2, 193.4; IR (neat) cm-1 3027w, 2840w, 2729w, 1686s, 1495m, 1449m, 1359m, 1112s, 966s; mass spectrum (APCI): m/e (% relative intensity) 203 (M+H)+ (77), 117 (68), 101 (100).
Vinyl Aldehyde Cis-78. – Prepared according to general procedure 1 to afford vinyl aldehyde cis-78 in 56% yield as a colorless oil.
Cis-78: Rf = 0.63 [33% EtOAc in hexanes]; 1H NMR (400 MHz, CDCl3) δ 4.26 (dd, J = 2.0 and 4.4 Hz, 2H), 4.33 (dd, J = 2.0 and 6.4 Hz, 2H), 5.85 (dt, J = 6.4 and 11.6 Hz, 1H), 6.35 (ddt, J = 2.0, 8.0, and 15.6 Hz, 1H), 6.66 (dt, J = 2.0 and 11.6 Hz, 1H), 6.82 (dt, J = 4.4 and 15.6 Hz, 1H), 7.18-7.38 (m, 5H), 9.57 (d, J = 8.0 Hz, 1H); 13C NMR (100 MHz, CDCl3) δ 67.9, 69.0, 127.6, 128.3, 128.5, 129.0, 132.1, 132.6, 136.6, 153.2, 193.5; IR (neat) cm-1 3025w, 2825w, 1686s, 1494w, 1447w, 1341w; mass spectrum (APCI): m/e (% relative intensity) 203 (M+H)+ (75), 173 (8), 117 (100).
Vinyl Acetal Trans-32. – Prepared according to general procedure 2 to afford vinyl acetal trans-32 (365.0 mg, 99%) as a white solid.
Trans-32: Rf= 0.20 [15% EtOAc in hexanes]; mp 56-59 °C; 1H-NMR (400MHz, CDCl3) δ 3.88-4.04 (m, 4H), 4.08 (dd, J = 1.6 and 5.2 Hz, 2H), 4.16 (dd, J = 1.6 and 6.0 Hz, 2H), 5.30 (dd, J = 0.8 and 6.0 Hz, 1H), 5.78 (ddt, J = 1.6, 6.0, and 15.6 Hz, 1H), 6.04 (ddt, J = 0.8, 5.2, and 15.6 Hz, 1H), 6.28 (dt, J = 6.0 and 16.0 Hz, 1H), 6.62 (dt, J = 1.6 and 16.0 Hz, 1H), 7.21-7.40 (m, 5H); 13C NMR (100 MHz, CDCl3) δ 65.2, 69.6, 71.1, 103.4, 126.1, 126.7, 127.9, 128.6, 128.7, 132.7, 132.9, 136.9; IR (film) cm-1 3026w, 2883w, 2850w, 1495w, 1459w, 1391m, 1359m; mass spectrum (APCI): m/e (% relative intensity) 247 (M+H) + (100), 186 (14), 117 (33).
Vinyl Acetal Cis-32. – Prepared according to general procedure 2 to afford vinyl acetal cis-32 in 85% yield as a colorless oil.
Cis-32: Rf = 0.19 [15% EtOAc in hexane]; 1H NMR (400 MHz, CDCl3) δ 3.86-4.02 (m, 4H), 4.04 (dd, J = 1.6 and 5.6 Hz, 2H), 4.26 (dd, J = 1.6 and 6.4 Hz, 2H), 5.27 (dd, J = 0.8 and 6.0 Hz, 1H), 5.73 (ddt, J = 1.6, 6.0, and 15.6 Hz, 1H), 5.85 (dt, J = 6.4 and 12.0 Hz, 1H), 6.01 (ddt, J = 0.8, 5.2, and 15.6 Hz, 1H), 6.61 (dt, J = 1.6 and 12.0 Hz, 1H), 7.18-7.37 (m, 5H); 13C NMR (100 MHz, CDCl3) δ 65.2, 67.4, 69.8, 103.4, 127.4, 128.5, 128.6, 129.0, 129.1, 132.0, 132.8, 136.8; IR (neat) cm-1 2882w, 2846w, 1600w, 1494w, 1400w; mass spectrum (APCI): m/e (% relative intensity) 247 (M+H)+ (100), 185 (25), 129 (18), 117 (55).
Cyclobutane 33. – Prepared according to general procedure 3 to afford cyclobutane 33 (40.0 mg, 47%) as a colorless oil from trans-32. Also prepared according to general procedure 3 in 45% yield from cis-32.
33: Rf= 0.46 [33% EtOAc in hexanes]; 1H-NMR (400MHz, CDCl3) δ 2.57 (ddt, J = 1.2, 5.6, and 10.0 Hz, 1H), 2.97 (ddd, J = 2.0, 4.8, and 8.0 Hz, 1H), 3.29 (ddd, J = 1.2, 5.6, and 8.4 Hz, 1H), 3.49 (ddd, J = 1.2, 6.0, and 10.0 Hz, 1H), 3.55-3.77 (m, 6H), 3.93 (d, J = 9.2 Hz, 1H), 4.02 (d, J = 9.6 Hz), 4.68 (d, J = 5.6 Hz, 1H), 7.10-7.40 (m, 5H); 13C NMR (100 MHz, CDCl3) δ 37.6, 42.3, 43.4, 44.8, 65.0, 65.0, 73.9, 73.9, 104.4, 126.3, 128.2, 128.6, 140.4; IR (neat) cm-1 3027w, 2957w, 2848w, 1603w, 1495w, 1452w; mass spectrum (APCI): m/e (% relative intensity) 247 (M+H)+ (100), 217 (12), 185 (16), 117 (10); HRMS-ESI m/e calcd for C15H19O3 247.1329, found 247.1339.
Vinyl Acetal 34. – Prepared according to general procedure 2 to afford vinyl acetal 34 (340 mg, 79%) as a colorless oil.
34: Rf = 0.30 [20% EtOAc in hexanes]; 1H NMR (400 MHz, CDCl3) δ 1.36 (dtt, J = 2.4, 4.8, and 10.4 Hz, 1H), 1.62 (d, J = 1.2 Hz, 3H), 1.69 (d, J =1.6 Hz, 3H), 2.12 (ddddd, J = 4.8, 4.8, 10.4, 10.4, and 11.6 Hz, 1H), 2.27 (dt, J = 7.2 and 7.2 Hz, 2H), 3.40 (t, J = 7.2 Hz, 2H), 3.84 (ddd, J = 2.4, 10.2, and 11.6 Hz, 2H), 4.00 (ddd, J = 0.8, 1.6, and 5.2 Hz, 2H), 4.15 (dddd, J = 1.6, 1.6, 4.8, and 12.0 Hz, 2H), 5.00 (dd, J = 0.8 and 4.4 Hz, 1H), 5.12 (ttt, J = 1.2, 1.6, and 7.2 Hz, 1H), 5.74 (ddt, J = 1.6, 4.4 and 16.0 Hz, 1H), 5.98 (ddt, J = 0.8, 5.2, and 16.0 Hz, 1H); 13C NMR (100MHz, CDCl3) δ 18.0, 25.9, 28.9, 67.1, 70.4, 100.4, 120.6, 128.9, 131.4, 133.7; IR (neat) cm-1 3052w, 2932w, 1377m, 1140s, 966s; mass spectrum (APCI): m/e (% relative intensity) 227 (M+H)+ (100), 183 (12), 151 (70).
Cyclobutane 35. – Prepared according to general procedure 3 to afford cyclobutane 35 (31.0 mg, 50%) as a colorless oil from 34.
35: Rf = 0.23 [20% EtOAc in hexanes]; 1H NMR (400 MHz, CDCl3) δ 1.01 (s, 3H), 1.12 (s, 3H), 1.28-1.32 (m, 1H), 1.50-1.58 (m, 1H), 1.82-1.96 (m, 2H), 2.00-2.12 (m, 2H), 2.30 (dd, J = 8.0 and 10.4 Hz, 1H), 3.05 (ddd, J = 2.0, 11.2, and 12.0 Hz, 1H), 3.50 (dd, J =4.4 and 12.0 Hz, 1H), 3.65-3.73 (m, 2H), 3.80-3.87 (m, 2H), 4.03-4.08 (m, 2H), 4.46 (d, J = 7.6 Hz, 1H); 13C NMR (125 MHz, CDCl3) δ 23.8, 24.2, 24.6, 26.2, 30.2, 36.6, 37.5, 46.1, 65.1, 66.6, 66.8, 68.6, 104.1; IR (neat): cm-1 2953m, 2923m, 1357m, 1144s, 1104s, 1020s, 973s; mass spectrum (APCI): m/e (% relative intensity) 227 (M+H)+ (100), 183(14), 151 (74).
Vinyl Acetal 36. – Prepared according to general procedure 2 to afford vinyl acetal 36 (250 mg, 66%) as a colorless oil.
36: Rf = 0.53 [33% EtOAc in hexanes]; 1H NMR (400 MHz, CDCl3) δ 1.55 (d, J = 0.8 Hz, 3H), 1.62 (d, J = 1.2 Hz, 3H), 2.26 (ddt, J = 1.6, 6.8, and 7.2 Hz, 2H), 3.36 (t, J = 7.2 Hz, 2H), 3.76-3.81 (m, 2H), 3.83 (d, J = 7.2 Hz, 2H), 3.86-3.91 (m, 2H), 5.07 (d, J = 6.4 Hz, 1H), 5.24 (tqq, J = 0.8, 1.2, and 7.2 Hz, 1H), 5.43 (dddd, J = 1.2, 1.6, 6.0, and 15.6 Hz, 1H), 5.84 (dddd, J = 0.4, 6.4, 7.2, and 15.6 Hz, 1H); 13C NMR (100MHz, CDCl3) δ 18.1, 25.9, 32.7, 65.0, 67.4, 69.1, 104.1, 121.3, 128.1, 134.0, 137.0.
Cyclobutane 37. – Prepared according to general procedure 3 to afford cyclobutane 37 (25.0 mg, 45%) as a colorless oil from 36.
37: Rf = 0.47 [33% EtOAc in hexanes]; 1H NMR (400 MHz, CDCl3) δ 1.05 (s, 3H), 1.15 (s, 3H), 1.54 (ddt, J = 2.0, 4.0, and 14.0 Hz, 1H), 1.80 (ddt, J = 6.8, 11.6, and 14.0 Hz, 1H), 1.94 (ddd, J = 8.4, 8.4, and 8.4 Hz, 1H), 2.19 (dd, J = 7.2 and 9.6 Hz, 1H), 2.45 (dddd, J = 2.4, 7.2, 7.2 and 8.8 Hz, 1H), 3.55 (ddd, J = 4.4, 10.2 and 10.2 Hz, 1H), 3.69 (dd, J = 9.2 and 12.0 Hz, 1H), 3.76-3.78 (m, 1H), 3.80-3.83 (m, 2H), 3.92-3.95 (m, 2H), 3.94-3.97 (m, 1H), 4.83 (d, J = 7.2 Hz, 1H); 13C NMR (100MHz, CDCl3) δ 24.4, 24.5, 25.0, 26.4, 36.5, 38.5, 47.2, 64.7, 64.8, 64.9, 67.1, 105.8; mass spectrum (APCI): m/e (% relative intensity) 213 (M+H)+ (100); HRMS-ESI m/e calcd for C14H23NaO3 235.1305, found 235.1302.
Synthesis of Vinyl Aldehyde 40.
DIAD (1.52 mL, 7.31 mmol), was added dropwise in the dark to a solution of alcohol 39 (0.97 g, 5.62 mmol), sulfonamide 38 (1.75 g, 7.31 mmol), and Ph3P in anhyd. THF (50 mL) at rt. After stirring for 24 hours, H2O was added (100 mL) and the aqueous phase was extracted with hexane (3 x 50 mL). The combined organic extracts were washed with brine, dried (MgSO4), and filtered. The filtrate was evaporated in vacuo and the resulting residue was purified by flash liquid chromatography over silica gel (EtOAc:Hexanes 1:4) to afford THP-protected alcohol S7 (1 g, 2.54 mmol, 45% yield) as a colorless oil.
S7: Rf= 0.64 [33% EtOAc in hexanes]; 1H-NMR (400MHz, CDCl3) δ 1.48-1.61 (m, 7H), 1.66 (s, 3H), 1.70 (dt, J = 3.2, 9.6 Hz, 1 H), 1.75-1.86 (m, 1H), 2.42 (s, 3H), 3.49 (m, 1H), 3.78 (d, J = 6.4 Hz, 4H), 3.82 (ddd, J = 3.2, 8.4, and 11.6 Hz, 1H), 3.91 (ddd, J = 0.8, 6.0 and 12.8 Hz, 1H), 4.16 (ddd, J = 0.8, 5.6 and 13.2 Hz, 1H), 4.58 (dd, J = 2.8 and 4.0 Hz, 1H), 4.98 (tqq, J = 1.2, 1.2 and 7.2 Hz, 1H), 5.55 (dtdd, J = 0.8, 0.8, 6.0 and 15.6 Hz, 1H), 5.66 (dtdd, J = 0.8, 0.8, 5.6 and 15.6 Hz, 1H), 7.28 (d, J = 8.0 Hz, 2H), 7.69 (d, J = 8.0 Hz, 2H).
To a solution of S7 (0.54 g, 1.37 mmol) in MeOH (15 mL) was added p-toluenesulfonic acid (0.01 g, 0.07 mmol) to stir overnight at rt. The MeOH was removed under reduced pressure and the crude residue was purified by flash chromatography ( EtOAc:Hexanes 1:2) to afford alcohol S8 (0.41 g, 1.31 mmol, 96% yield) as a colorless oil.
S8: Rf= 0.25 [33% EtOAc in hexanes]; 1H-NMR (400MHz, CDCl3) δ 1.60 (s, 3H), 1.67 (s, 3H), 2.43 (s, 3H), 3.78 (d, J = 6.8 Hz, 2H), 3.83 (d, J = 6.8 Hz, 2H), 4.16 (t, J = 6.0 Hz, 2H), 5.01 (tqq, J = 1.2, 1.2 and 6.8 Hz, 1H), 5.46 (dtt, J = 1.6, 6.8 and 11.2 Hz, 1H), 5.74 (dtt, J = 1.6, 6.4 and 11.2 Hz, 1H), 7.30 (d, J = 8.8 Hz, 2H), 7.70 (d, J = 8.8 Hz, 2H).
Vinyl Aldehyde 40. – Prepared according to general procedure 1 to afford vinyl aldehyde 40 (0.70 g, 66%) as a colorless oil from allylic alcohol S8.
40: Rf= 0.37 [33% EtOAc in hexanes]; 1H-NMR (400MHz, CDCl3) δ 1.56 (s, 3H), 1.65 (s, 3H), 2.44 (s, 3H), 3.79 (d, J = 7.2 Hz, 2H), 3.97 (dd, J = 1.6, 6.0 Hz, 2H), 4.98 (tqq, J = 1.2, 1.2 and 7.2 Hz, 1H), 6.14 (ddt, J = 1.6, 7.6 and 15.6 Hz, 1H), 6.69 (dt, J = 6.0 and 15.6 Hz, 1H), 7.32 (d, J = 8.0 Hz, 2H), 7.70 (d, J = 8.0 Hz, 2H), 9.51 (d, J = 7.6 Hz, 1H); 13C NMR (100 MHz, CDCl3) δ 14.4, 18.0, 21.7, 25.9, 46.0, 48.0, 118.3, 127.5, 130.3, 133.5, 136.7, 138.5, 143.9, 152.5, 193.1; mass spectrum (APCI): m/e (% relative intensity) 308.2 (M+H)+ (100), 222.0 (30), 240.0 (20).
Vinyl Acetal 41. – Prepared according to general procedure 2 to afford vinyl acetal 41 (304.0 mg, 48%) as a colorless oil from vinyl aldehyde 40.
41: Rf= 0.51 [33% EtOAc in hexanes]; 1H-NMR (400MHz, CDCl3) δ 1.58 (s, 3H), 1.65 (s, 3H), 2.42 (s, 3H), 3.77 (d, J = 7.2 Hz, 2H), 3.79 (d, J = 7.2 Hz, 2H), 3.84-3.99 (AA’BB’, 4H), 4.96 (tqq, J = 1.2, 1.2 and 7.2 Hz, 1H), 5.18 (d, J = 6.4 Hz, 1H), 5.55 (ddt, J = 1.2, 6.0 and 15.6 Hz, 1H), 5.75 (dt, J = 7.2 and 15.6 Hz, 1H), 7.28 (d, J = 8.0 Hz, 2H), 7.69 (d, J = 8.0 Hz, 2H).
Cyclobutane 42. – Prepared according to general procedure 3 to afford 42 (15.5 mg, 25% yield) and 43 (9 mg, 13% yield) as colorless oils from vinyl acetal 41.
42: Rf= 0.43 [33% EtOAc in hexanes]; 1H-NMR (400MHz, CDCl3) δ 1.06 (s, 3H), 1.14 (s, 3H), 1.98 (t, J = 7.2 Hz, 1H), 2.20 (t, J = 8.0 Hz, 1H), 2.43 (s, 3H), 2.48 (dd, J = 10.4 and 13.6 Hz, 1H), 2.50 (dd, J = 4.0 and 9.2 Hz, 1H), 2.67 (ddd, J = 0.8, 7.2 and 13.6 Hz, 1H), 3.41 (d, J = 9.2 Hz, 1H), 3.59 (d, J = 10.4 Hz, 1H), 3.77-3.95 (m, 4H), 4.93 (d, J = 7.2 Hz, 1H), 7.31 (d, J = 7.6 Hz, 2H), 7.70 (d, J = 7.6 Hz, 2H); 13C NMR (100 MHz, CDCl3) δ 21.8, 24.5, 25.6, 34.3, 34.8, 45.7, 49.3, 49.6, 53.3, 64.9, 65.1,104.9, 128.4, 129.8, 132.2, 143.8; mass spectrum (APCI): m/e (% relative intensity) 352.1 (M+H)+ (100).
43: Rf= 0.36 [33% EtOAc in hexanes]; 1H-NMR (400MHz, CDCl3) δ 1.49 (s, 3H), 1.53 (s, 3H), 1.74 (ddd, J = 4.4, 10.0 and 14.0 Hz, 1H), 1.90 (ddd, J = 4.0, 4.4 and 14.0 Hz, 1H), 2.17 (ddd, J = 5.6, 6.4 and 8.4 Hz, 1H), 2.36 (m, 1H), 2.44 (s, 3H), 3.02 (dd, J = 5.2 and 9.6 Hz, 1H), 3.08 (dd, J = 6.4 and 10.0 Hz, 1H), 3.27 (dd, J = 7.6 and 9.6Hz, 1H), 3.32 (dd, J = 8.8 and 10.0 Hz, 1H), 3.78-3.98 (AA’BB’, 4H), 4.81 (t, J = 4.4 Hz, 1H), 7.34 (d, J = 8.4 Hz, 2H), 7.70 (d, J = 8.4 Hz, 2H); mass spectrum (APCI): m/e (% relative intensity) 352.1 (M+H)+ (60), 388.1 (M+Cl)+ (100), 390.1 (30); HRMS-ESI m/e calcd for C18H27ClNO4S 388.1344, found 388.1363.
Vinyl Acetal 44. – Prepared according to general procedure 2 to afford vinyl acetal 44 (400.0 mg, 70%) as a colorless oil.
44: Rf= 0.42 [50% EtOAc in hexanes]; 1H-NMR (400MHz, CDCl3) δ 1.55 (s, 3H), 1.60 (s, 3H), 2.14-2.26 (m, 2H), 3.08-3.20 (m, 2H), 3.57 (s, 3H), 3.66-3.80 (m, 4H), 3.85-3.91 (m, 2H), 4.96-5.06 (m, 1H), 5.07 (d, J = 6.4 Hz, 1H), 5.37-5.46 (m, 1H), 5.77 (dt, J = 6.4 and 15.6 Hz, 1H); 13C NMR (100 MHz, CDCl3) δ 18.0, 25.9, 31.4, 45.0, 45.6, 46.3, 54.7, 65.2, 104.0, 120.7, 128.5, 134.1, 156.9.
Cyclobutane 45. – Prepared according to general procedure 3 to afford cyclobutane 45 (16.2 mg, 30% yield) as a colorless oil from 44.
45: Rf= 0.36 [50% EtOAc in hexanes]; 1H-NMR (400MHz, CDCl3) δ 1.03 (s, 3H), 1.16 (s, 3H), 1.55-1.70 (m, 1H), 1.70-1.75 (m, 1H), 1.76-1.89 (m, 1H), 1.90-2.02 (m, 1H), 2.06 (dd, J = 7.6 and 9.2 Hz, 1H), 2.44 (ddt, J = 5.2, 5.6 and 5.6 Hz), 3.28-3.40 (m, 4H), 3.69 (s, 3H), 2.77-3.96 (m, 4H), 4.84 (d, J = 7.2 Hz, 1H); mass spectrum (APCI): m/e (% relative intensity) 270 (M+H)+ (100); HRMS-ESI m/e calcd for C14H24NO4 270.1700, found 270.1699.
Vinyl Acetal 46. – Prepared according to general procedure 2 to afford vinyl acetal 46 (200.0 mg, 67%) as a colorless oil.
46: Rf= 0.18 [20% EtOAc in hexanes]; 1H-NMR (400MHz, CDCl3) δ 1.50 (s, 3H), 1.57 (s, 3H), 2.04-2.14 (m, 2H), 3.00-3.14 (m, 2H), 3.58 (s, 3H), 3.74-3.86 (m, 4H), 3.86-3.91 (m, 2H), 4.90-5.00 (m, 1H), 5.13 (d, J = 6.0 Hz, 1H), 5.42-5.54 (m, 1H), 5.70-5.83 (m, 1H); 13C NMR (100 MHz, CDCl3) δ 17.9, 25.9, 27.1, 27.5, 46.5, 47.4, 48.6, 52.8, 65.2, 103.3, 120.7, 128.3, 128.9, 132.3, 134.3, 156.9.
Cyclobutane 47. – Prepared according to general procedure 3 to afford cyclobutane 47 (19.3 mg, 40% yield) as a colorless oil from 46.
47: Rf= 0.20 [20% EtOAc in hexanes]; 1H-NMR (400MHz, CDCl3) δ 1.02 (s, 3H), 1.17 (s, 3H), 1.56-1.92 (m, 4H), 1.93-2.02 (m, 1H), 2.34-2.50 (m, 1H), 2.84-2.98 (m, 1H), 3.28-3.46 (m, 1H), 3.54-3.62 (m, 1H), 3.69 (s, 3H), 3.78-3.86 (m, 2H), 3.90-3.94 (m, 2H), 4.86 (d, J = 7.2 Hz, 1H); HRMS-ESI m/e calcd for C14H24NO4 270.1700, found 270.1703.
Synthesis of Vinyl Aldehyde 50.
THP-protected allyl alcohol 49 To a solution of NaH (733 mg, 18.3 mmol) in THF (40 mL) was added diethyl malonate (2.65 mL, 17.45 mmol) at 0 oC. The mixture was warmed to rt to stir for 1h. 4-bromo-2-methyl-2-butene (2.44 mL, 20.94 mmol) was added and the mixture stirred for 24 h, and the progress of the reaction was monitored by TLC. Upon disappearance of the starting material, the reaction was quenched with sat aq NH4Cl and extracted with EtOAc (3 × 100 mL). The combined organic layers were washed with sat aq NaCl (100 mL), dried over Na2SO4, and concentrated in vacuo. Further purification was performed by silica gel flash column chromatography [gradient eluent: 2:1 to 1:1 hexane/EtOAc] to afford diethyl malonate S9 (3.40 g, 86%) as colorless oil.
To a solution of NaH (1.08 g, 27.0 mmol) in THF (30 mL) was added diethyl malonate S9 (2.46 g, 10.8 mmol) at 0 oC. The mixture was warmed to rt to stir for 1h. Tetrahydro-2-[(4-iodo-2-butenyl)oxy]-2H- pyran (3.66 g, 13.0 mmol) was added and the mixture stirred for 24 h, and the progress of the reaction was monitored by TLC. Upon disappearance of the starting material, the reaction was quenched with sat aq NH4Cl and extracted with EtOAc (3 × 100 mL). The combined organic layers were washed with sat aq NaCl (100 mL), dried over Na2SO4, and concentrated in vacuo. Further purification was performed by silica gel flash column chromatography [gradient eluent: 2:1 to 1:1 hexane/EtOAc] to afford THP-protected allyl alcohol 49. (3.80 g, 92%) as colorless oil.
49: Rf = 0.31 [10% EtOAc in hexanes]; 1H NMR (400 MHz, CDCl3) δ 1.18-1.25 (m, 6H), 1.44-1.62 (m, 6H), 1.64-1.74 (m, 4H), 1.76-1.86 (m, 2H), 2.52-2.68 (m, 4H), 3.44-3.53 (m, 2H), 3.78-4.07 (m, 2H), 4.10-4.20 (m, 4H), 4.55-4.66 (m, 1H), 4.90-5.00 (m, 1H), 5.44-5.72 (m, 2H).
Vinyl Aldehyde 50. – Prepared according to general procedure 1 to afford vinyl aldehyde 50 (1.0 g, 59%) as colorless oil from allylic alcohol.
50: Rf = 0.53 [33% EtOAc in hexanes]; 1H NMR (400 MHz, CDCl3) δ 1.25 (t, J = 7.2 Hz, 3H), 1.61 (d, J = 0.8 Hz, 3H), 1.70 (d, J = 1.2 Hz, 3H), 2.63 (d, J = 7.6 Hz, 2H), 2.85 (dd, J = 1.2 and 8.0 Hz, 2H), 4.21 (t, J = 7.2 Hz, 4H), 4.96 (tqq, J = 0.8, 1.2 and 7.6 Hz, 1H), 6.11 (dddd, J = 1.2, 1.6, 8.0 and 15.6 Hz, 1H), 6.77 (ddd, J = 7.2, 8.0 and 15.6 Hz, 1H), 9.49 (d, J = 8.0 Hz, 1H).
Vinyl Acetal 51. – Prepared according to general procedure 2 to afford vinyl acetal 51 (160.0 mg, 44%) as a colorless oil.
51: Rf = 0.42 [33% EtOAc in hexanes]; 1H NMR (400 MHz, CDCl3) δ 1.23 (t J = 7.2 Hz, 6H), 1.60 (d, J = 0.4 Hz, 3H), 1.68 (d, J = 0.8 Hz, 3H), 2.58 (d, J = 7.6 Hz, 2H), 2.62 (dd, J = 1.2 and 7.6, 2H), 3.84-3.87 (m, 2H), 3.92-3.98 (m, 2H), 4.12-4.22 (m, 4H), 4.95 (tqq, J = 0.4, 0.8 and 7.6 Hz, 1H), 5.17 (d, J = 6.0 Hz, 1H), 5.52 (dddd, J = 1.2, 1.2, 6.0 and 15.2 Hz, 1H), 5.77 (ddd, J = 7.2, 7.6, and 15.2 Hz, 1H); 13C NMR (100MHz, CDCl3) δ 14.3, 18.2, 26.2, 31.3, 35.2, 57.7, 61.4, 65.0, 103.6, 117.6, 130.9, 131.0, 135.9, 171.1.
Cyclobutane 56. – Prepared according to general procedure 3 to afford 56 (20.5 mg, 30% yield) and 57 (19.0 mg, 28% yield) as colorless oils from 51.
56: Rf = 0.46 [33% EtOAc in hexanes]; 1H NMR (400 MHz, CDCl3) δ 1.00 (s, 3H), 1.15 (s, 3H), 1.23 (t, J = 7.2 Hz, 3H), 1.25 (t, J = 7.2 Hz, 3H), 1.81 (dd, J = 7.2 and 7.6 Hz, 1H), 2.03 (dd, J = 4.0 and 13.6 Hz, 1H), 2.24 (dd, J = 7.6 and 8.4 Hz, 1H), 2.34-2.38 (m, 2H), 2.55 (ddd, J = 0.8, 8.4 and 13.6 Hz, 1H), 2.59-2.65 (m, 1H), 3.80-3.83 (m, 2H), 3.90-3.92 (m, 2H), 4.19-4.55 (m, 4H), 4.86 (d, J = 7.2 Hz, 1H); mass spectrum (APCI): m/e (% relative intensity) 341 (M+H)+ (100); HRMS-ESI m/e calcd for C18H29O6 341.1959, found 341.1976.
57: Rf = 0.42 [33% EtOAc in hexanes]; 1H NMR (400 MHz, CDCl3) δ 1.25 (q, J = 7.2 Hz, 6H), 1.56 (s, 3H), 1.60 (s, 3H), 1.88 (ddd, J = 3.2, 5.6 and 14.0 Hz, 1H), 2.00-2.21 (m, 3H), 2.30-2.40 (m, 1H), 2.48-2.65 (m, 2H), 3.78-3.89 (m, 2H), 3.90-4.00 (m, 2H), 4.11-4.24 (m, 4H), 4.86 (dd, J = 4.0 and 5.6 Hz, 1H).
Silyl Ether S10. To a solution of Ph3PCH(CH3)2 (16.6 g, 38.4 mmol) in THF (150 mL) was added 1.6M n-BuLi (24.0 mL, 38.4 mmol) in THF (50 mL) dropwise at 0 oC, stirred for 30 min before adding aldehyde 52 (5.90 g, 25.6 mmol) at 0 oC. The resulting reaction mixture was warmed to rt, stirred for 2 h, and the progress of the reaction was monitored using TLC. Upon completion, the reaction was quenched with sat aq NH4Cl, extracted with diethyl ether (3 × 150 mL). The combined organic layers were washed with sat aq NaCl (150 mL), dried over Na2SO4, and concentrated in vacuo. Further purification was performed by silica gel flash column chromatography [gradient eluent: 40:1 to 20:1 hexane/EtOAc] to afford S10 (6.30 g, 96%) as colorless oil. S10: Rf = 0.40 [5% EtOAc in hexanes]; 1H NMR (400 MHz, CDCl3) δ 0.06 (s, 6H), 0.90 (s, 9H), 1.30-1.37 (m, 4H), 1.52 (tt, J = 6.4 and 6.8 Hz, 2H), 1.61 (d, J = 1.2 Hz, 2H), 1.69 (d, J = 1.6 Hz, 3H), 1.97 (dt, J = 6.4 and 6.8 Hz, 2H), 3.61(t, J = 6.4 Hz, 2H), 5.12 (ttt, J = 1.2, 1.6, and 6.8 Hz, 1H).
To a solution of S10 (6.07 g, 23.7 mmol) in THF (100 mL) was added 1.0 M TBAF (36.0 mL, 36.0 mmol) at -20 oC and warmed to rt. The resulting reaction mixture was stirred for 12 h, and the progress of the reaction was monitored using TLC. Upon completion, the reaction was quenched with water, extracted with diethyl ether (3 × 100 mL). The combined organic layers were washed with sat aq NaCl (100 mL), dried over Na2SO4, and concentrated in vacuo. Further purification was performed by silica gel flash column chromatography [gradient eluent: 10:1 to 4:1 hexane/EtOAc] to afford alcohol S11 (6.30 g, 96%) as colorless oil.
To a solution of oxalyl chloride (2.50 mL, 28.2 mmol) in anhyd CH2Cl2 (50 mL) was added DMSO (4.00 mL, 56.4 mmol) in anhyd CH2Cl2 (50 mL) at -78 oC and stirred for 30 min at the same temperature. The alcohol S11 (3.34 g, 23.5 mmol) in anhyd CH2Cl2 (50 mL) was added dropwise at -78 oC and stirred for 30 min. Et3N (16.2 mL, 118.0 mmol) was added dropwise. The resulting reaction mixture was warmed to rt and stirred for 1 h. The reaction was quenched with water and extracted with CH2Cl2 (3 × 100 mL). The combined organic layers were washed with sat aq NaCl (100 mL), dried over Na2SO4, and concentrated in vacuo. Further purification was performed by silica gel flash column chromatography [gradient eluent: 20:1 to 6:1 hexane/EtOAc] to afford aldehyde 53 (2.90 g, 88%) as yellow oil. 53: Rf = 0.40 [10% EtOAc in hexanes]; 1H NMR (400 MHz, CDCl3) δ 1.37 (tt, J = 7.2 and 7.6 Hz, 2H), 1.60 (d, J = 1.2 Hz, 2H), 1.60-1.67 (m, 2H), 1.69 (d, J = 1.6 Hz, 3H), 2.00 (dt, J = 7.2 and 7.6 Hz, 2H), 2.42 (dt, J = 2.0 and 7.2 Hz, 2H), 5.01 (ttt, J = 1.2, 1.6, and 6.8 Hz, 1H), 9.76 (d, J = 2.0 Hz, 1H).
Vinyl Aldehyde 54. To a solution of formylmethylenetriphenylphosphorane (3.90 g, 12.9 mmol) in CHCl3 (50 mL) was added aldehyde 53 (1.60 g, 11.7 mmol) in CHCl3 (30 mL) dropwise at 0 oC. The resulting reaction mixture was stirred for 2 h at 0 oC, then warmed to rt, and stirred for 12 h. The progress of the reaction was monitored using TLC. Upon completion, the solvent was removed in vacuo. Further purification was performed by silica gel flash column chromatography [gradient eluent: 20:1 to 6:1 hexane/EtOAc] to afford vinyl aldehyde 54 (1.46 g, 75%) as colorless oil.
54: Rf= 0.40 [10 % EtOAc in hexanes]; 1H NMR (400 MHz, CDCl3) δ 1.38 (tt, J = 7.2 and 7.6 Hz, 2H), 1.52 (tt, J = 7.2 and 8.0 Hz, 2H), 1.60 (d, J = 0.8 Hz, 3H), 1.69 (d, J = 1.2 Hz, 3H), 2.00 (dt, J = 7.2 and 7.6 Hz, 2H), 2.34 (ddt, J = 1.2, 7.2, and 8.0 Hz, 2H), 5.10 (ttt, J = 0.8, 1.2, and 7.2 Hz, 1H), 6.12 (ddt, J = 1.2, 7.6, and 15.6 Hz, 1H), 6.85 (dt, J = 7.2 and 15.6 Hz, 1H), 9.50 (d, J = 7.6 Hz, 1H); 13C NMR (100 MHz, CDCl3) δ 17.9, 25.9, 27.6, 29.5, 32.9, 124.3, 132.1, 133.2, 159.2, 194.4; IR (neat) cm-1 2929w, 2858w, 1689, 1377w, 1124m, 975m; mass spectrum (ESI): m/e (% relative intensity) 189.2 (M+Na)+ (100), 149.2 (10).
Vinyl Acetal 55. – Prepared according to general procedure 2 to afford vinyl acetal 55 (303.0 mg, 60%) as a colorless oil.
55: Rf = 0.39 [10% EtOAc in hexanes]; 1H NMR (400 MHz, CDCl3) δ 1.29-1.45 (m, 4H), 1.59 (d, J = 1.2 Hz, 3H), 1.68 (d, J = 1.6 Hz, 3H), 1.96 (dt, J = 7.2 and 7.2 Hz, 2H), 2.08 (ddt, J = 1.2, 6.8, and 7.2 Hz, 2H), 3.87-3.93 (m, 2H), 3.96-4.02 (m, 2H), 5.09 (ttt, J = 1.2, 1.6, and 7.2 Hz, 1H), 5.18 (d, J = 6.8 Hz, 1H), 5.48 (dddd J = 1.2, 1.6, 6.8, and 15.6 Hz, 1H), 5.93 (dt, J = 6.8 and 15.6 Hz, 1H); 13C NMR (100 MHz, CDCl3) δ 17.9, 25.9, 28.4, 29.5, 32.2, 65.1, 104.4, 124.7, 126.3, 131.6, 138.1; IR (neat) cm-1 2968m, 2856w, 1141s, 1059s, 961s; mass spectrum (ESI): m/e (% relative intensity) 233.2 (M+Na)+ (100), 211.2 (5).
Cyclobutane 58. – Prepared according to general procedure 3 (using 50 mol% of 5 wt% FeCl3 on silica gel) to afford cyclobutane 58 (6.40 mg, 64%) as a colorless oil from 55.
58: Rf= 0.42 [10% EtOAc in hexanes]; 1H NMR (400 MHz, CDCl3) δ 0.99 (s, 3H), 1.14 (s, 3H), 1.25-1.77 (m, 9H), 1.98 (dd, J = 7.2 and 10.8 Hz, 1H), 2.32 (dddd, J = 2.0, 8.0, 8.0, and 8.4 Hz, 1H), 3.75-3.85(m, 2H), 3.88-3.97 (m, 2H), 4.77 (d, J = 7.2 Hz, 1H); 13C NMR (100 MHz, CDCl3) δ 22.6, 23.3, 24.3, 24.4, 25.8, 29.7, 36.7, 40.6, 47.0, 64.7, 106.4; IR (neat) cm-1 2926s, 1219m, 1059s, 940s; mass spectrum (APCI): m/e (% relative intensity) 211 (M+H)+ (100), 149 (50), 115 (10).
Vinyl Acetal 62. – Prepared according to general procedure 2 (using (2S,3S)-Bis- [(trimethylsilyl)oxy]butane) and trans-S5 to afford vinyl acetal 62 (258.0 mg, 70%) as a colorless oil.
62: Rf= 0.41 [10% EtOAc in hexanes]; 1H NMR (400 MHz, CDCl3) δ 1.25 (d, J = 6.0 Hz, 3H), 1.30 (d, J = 6.0 Hz, 3H), 1.66 (d, J = 1.2 Hz, 3H), 1.74 (d, J = 1.2 Hz, 3H), 3.60-3.69 (m, 2H), 3.95 (dt, J = 1.2 and 6.8 Hz, 2H), 4.00 (dd, J = 1.6 and 5.6 Hz, 2H), 5.34 (tsept, J = 1.2 and 6.8 Hz, 1H), 5.40 (dd, J = 0.4 and 6.0 Hz, 1H), 5.75 (ddt, J = 1.6, 6.0, and 15.6 Hz, 1H), 5.99 (dtd, J = 0.4, 5.6, and 15.6 Hz); 13C NMR (100 MHz, CDCl3) δ 17.1, 17.2, 26.0, 66.9, 69.5, 78.4, 80.0, 102.4, 121.1, 129.4, 133.0, 137.4; mass spectrum (APCI): m/e (% relative intensity) 227 (M+H)+ (100).
Cyclobutane 63. – Prepared according to general procedure 3 to afford 63 (14.8 mg, 30% yield), 64 (9.9 mg, 20% yield), and 65 (20.2 mg, 40% yield) as colorless oils from 62.
63: Rf= 0.36 [10% EtOAc in hexanes]; 1H NMR (400 MHz, CDCl3) δ 0.98 (s, 3H), 1.17 (s, 3H), 1.20-1.36 (m, 6H), 1.72-1.78 (m, 1H), 2.31 (t, J = 7.2 Hz, 1H), 2.78 (dq, J = 6.4 and 7.6 Hz, 1H), 3.32-3.40 (m, 2H), 3.51-3.62 (m, 2H), 3.77 (dd, J = 4.0 and 8.8 Hz, 1H), 4.04 (d, J = 10.0 Hz, 1H), 5.12 (d, J = 7.2 Hz, 1H); HRMS-ESI m/e calcd for C13H22O3Na 249.1461, found 249.1474.
64: Rf= 0.29 [10% EtOAc in hexanes]; 1H NMR (400 MHz, CDCl3) δ 1.22 (d, J = 5.6 Hz, 3H), 1.28 (d, J = 5.6 Hz, 3H), 1.56 (s, 3H), 1.60 (s, 3H), 1.78 (dd, J = 4.8 and 10.4 Hz, 1H), 1.93 (ddd, J = 2.4, 4.4 and 6.8 Hz, 1H), 2.20 (ddd, J = 2.4, 5.2 and 7.6 Hz, 1H), 2.30-2.40 (m, 1H), 3.54-3.64 (m, 3H), 3.78-3.86 (m, 1H), 3.86-3.91 (t, J = 9.6 Hz, 1H), 3.97 (dt, J = 5.2 and 8.8 Hz, 1H), 5.04 (t, J = 4.4 Hz, 1H); HRMS-ESI m/e calcd for C13H22O3Na 249.1461, found 249.1472.
65: Rf= 0.22 [10% EtOAc in hexanes]; 1H NMR (400 MHz, CDCl3) δ 1.16 (d, J = 6.0 Hz, 3H), 1.17 (d, J = 6.0 Hz, 3H), 1.55 (s, 3H), 1.61 (s, 3H), 2.70-2.80 (m, 1H), 3.40-3.46 (m, 1H), 3.54-3.63 (m, 1H), 3.63-3.72 (m, 1H), 3.84-3.91 (m, 1H), 3.91-4.02 (m, 2H), 4.88 (dd, J = 9.6 and 12.4 Hz), 6.19 (d, J = 12.4 Hz); HRMS-ESI m/e calcd for C13H22O3Na 249.1461, found 249.1475.
Vinyl Acetal 66. – Prepared according to general procedure 2 (using (2S,3S)-Bis- [(trimethylsilyl)oxy]butane) and 21 to afford vinyl acetal 66 (150.0 mg, 50%) as a colorless oil.
66: Rf= 0.25 [20% EtOAc in hexanes]; 1H NMR (500 MHz, CDCl3) δ 1.23 (d, J = 4.5 Hz, 3H), 1.28 (d, J = 4.5 Hz, 3H), 1.60 (s, 3H), 1.68 (s, 3H), 2.25 (q, J = 7.5 Hz, 2H), 3.83 (t, J = 7.0 Hz, 2H), 3.60-3.67 (m, 2H), 4.00 (d, J = 5.5 Hz, 2H), 5.10 (dt, J = 1.5 and 7.0 Hz, 1H), 5.38 (d, J = 5.5 Hz, 1H), 5.72 (ddd, J = 1.5, 6.5 and 15.5 Hz, 1H), 5.96 (dt, J = 5.5 and 15.5 Hz, 1H); 13C NMR (125 MHz, CDCl3) δ 16.9, 17.0, 17.8, 25.7, 28.7, 70.1, 70.3, 78.2, 79.8, 102.2, 120.4, 129.0, 132.8, 133.5, ; IR (neat) cm-1 2971m, 2929w, 2857m, 1456w, 1380m, 1360w, 1323w, 1121m, 1081m, 1027w; HRMS-ESI m/e calcd for C14H24NaO3 263.1623, found 263.1620.
Cyclobutane 67. – Prepared according to general procedure 3 to afford cyclobutane 67 (24.3 mg, 40% yield) as a colorless oil from 66.
67: Rf= 0.20 [20% EtOAc in hexanes]; 1H NMR (500 MHz, CDCl3) δ 1.03 (s, 3H), 1.15 (s, 3H), 1.20 (d, J = 6.0 Hz, 3H), 1.23 (d, J = 6.0 Hz, 3H), 1.52-1.60 (m, 1H), 1.86-1.97 (m, 2H), 2.09-2.16 (m, 1H), 2.21-2.28 (m, 1H), 3.05 (t, J = 5.5 Hz, 1H), 3.48-3.60 (m, 4H), 3.86 (t, J = 8.0 Hz, 2H), 5.00 (d, J = 7.0 Hz, 1H); 13C NMR (125 MHz, CDCl3) δ 17.1, 17.2, 23.7, 24.1, 24.4, 65.0, 68.4, 77.8, 79.3, 104.4; IR (neat) cm-1 2956m, 2931w, 2871w, 2838w, 1457w, 1384w, 1317w, 1148w, 1106w, 1088w; HRMS-ESI m/e calcd for C14H24NaO3 263.1623, found 263.1614.
Vinyl Acetal 68. – Prepared according to general procedure 2 (using (2S,4S)-bis- (trimethylsilyloxy)pentane) and 21 to afford vinyl acetal 68 (230.0 mg, 78%) as a colorless oil.
68: Rf= 0.47 [20% EtOAc in hexanes]; 1H NMR (400 MHz, CDCl3) δ 1.20-1.25 (m, 3H), 1.32-1.38 (m, 1H), 1.37-1.41 (m, 3H), 1.61 (s, 3H), 1.69 (s, 3H), 1.87 (ddd, J = 6.0, 12.0 and 13.2 Hz, 1H), 2.26 (q, J = 7.2 Hz, 2H), 3.39 (dt, J = 3.6 and 7.2 Hz, 2H), 3.96-4.07 (m, 3H), 4.34 (pent, J = 6.8 Hz, 1H), 5.07-5.16 (m, 1H), 5.31 (d, J = 4.4 Hz, 1H), 5.73 (ddt, J = 1.6, 4.8 and 15.6 Hz, 1H), 5.99 (ddt, J = 0.8, 5.6 and 15.6 Hz); 13C NMR (100 MHz, CDCl3) δ 17.4, 18.0, 22.1, 25.9, 29.0, 36.9, 67.8, 68.4, 70.5, 70.6, 93.0, 130.6, 129.6, 131.3, 133.7.
Cyclobutane 69. – Prepared according to general procedure 3 to afford cyclobutane 69 (21 mg, 45% yield) as a colorless oil from 68.
69: Rf= 0.66 [33% EtOAc in hexanes]; 1H NMR (400 MHz, CDCl3) δ major – 1.00 (s, 3H), 1.11 (s, 3H), 1.12 (d, J = 6.0 Hz, 3H), 1.26-1.28 (m, 1H), 1.30 (d, J = 6.8 Hz, 3H), 1.50-1.59 (m, 1H), 1.75-1.96 (m, 3H), 1.97-2.08 (m, 1H), 2.25 (d, J = 8.0 Hz), 3.00-3.11 (m, 1H), 3.48 (d, J = 4.4 Hz, 1H), 3.80-3.90 (m, 3H), 4.18-4.29 (m, 1H), 4.77 (d, J = 5.6 Hz, 1H); 13C NMR (100 MHz, CDCl3) δ major – 17.6, 22.2, 23.6, 24.3, 24.6, 30.6, 36.4, 37.2, 37.8, 46.0, 65.2, 67.3, 67.8, 68.7, 96.0; mass spectrum (APCI): m/e (% relative intensity) 255 (M+H)+ (100); HRMS-ESI m/e calcd for C15H27O3 255.1955, found 255.1955.
Preparation and Gassman's Intermolecular [2 + 2] Cycloaddition of Vinyl Hemiaminal 74.
Vinyl Hemiaminal 74. To a solution of N-tosyl ethanolamine (1.55 g, 7.22 mmol) in dry 1,2-dichloroethane (50 mL) were added 4Å molecular sieves (500 mg), acrolein diethyl acetal (3.30 mL, 21.7 mmol), and pyridinium p-toluenesulfonate (544 mg, 2.17 mmol). The mixture was refluxed overnight, cooled to room temperature and, after EtOAc (50 mL) addition, filtered and the filtrate washed with a 5% aqueous NaHCO3 solution (100 mL) and brine (100 mL) and dried over Na2SO4. The mixture was filtered and the solvent removed in vacuo. Further purification was performed by flash chromatography [4:1 hexane:EtOAc] to afford vinyl hemiaminal 74 (1.71 g, 6.75 mmol, 93% yield) as a white solid.
74: Rf= 0.54 [33% EtOAc in hexanes]; 1H NMR (400 MHz, CDCl3) δ 2.44 (s, 3H), 3.38-3.45 (m, 1H), 3.46-3.54 (m, 2H), 3.80-3.90 (m, 1H), 5.32 (dt, J = 1.2 and 10.4 Hz, 1H), 5.50 (dt, J = 1.2 and 17.2 Hz, 1H), 5.57 (dt, 1.2 and 4.4 Hz, 1H), 5.83 (ddd, J = 4.4, 10.4 and 17.2 Hz, 1H), 7.32 (d, J = 8.8 Hz, 2H), 7.76 (d, J = 8.8 Hz, 2H).
Procedure with SnCl4.
To a solution of vinyl hemiaminal 74 (51.0 mg, 0.20 mmol) and 4Å molecular sieves (100 mg) in anhyd CH2Cl2 (2.0 mL) was added 1.0 M SnCl4 in CH2Cl2 (0.20 mL, 0.20 mmol) at -20 oC. The reaction mixture was stirred for 30 min under nitrogen atmosphere at the same temperature, and the progress of the reaction was monitored using TLC. Upon completion, the reaction was quenched via addition of pyridine. The resulting reaction mixture was filtered through a cotton plug and concentrated in vacuo. Further purification was performed by silica gel flash column chromatography [gradient eluent: 20:1 to 10:1 hexane/EtOAc] to afford 76 (41.2 mg, 61%) as colorless oil.
76 (mixture of diastereomers): Rf= 0.66 [33% EtOAc in hexanes]; 1H NMR (400 MHz, CDCl3) δ 0.85 (s, 9H), 0.86 (s, 9H), 0.93 (d, J = 2.0 Hz, 3H), 0.95 (d, J = 2.0 Hz, 3H), 1.95 (qdd, J = 0.8, 2.0 and 6.8 Hz, 1H), 1.97 (qdd, J = 0.8, 2.0 and 6.8 Hz, 1H), 2.43 (s, 6H), 3.38-3.56 (m, 6H), 3.82-3.90 (m, 2H), 5.36 (ddd, J = 0.8, 2.4 and 4.8 Hz, 1H), 5.40 (ddd, J = 0.8, 2.4 and 4.8 Hz, 1H), 5.55 (dd, J = 0.8 and 2.4 Hz, 1H), 5.56 (dd, J = 0.8 and 2.4 Hz, 1H), 5.84 (ddd, J = 1.2, 2.0 and 8.8 Hz, 1H), 5.88 (ddd, J = 1.2, 2.0 and 8.8 Hz, 1H), 7.32 (d, J = 8.0 Hz, 4H), 7.76 (d, J = 8.0 Hz, 4H); 13C NMR (100 MHz, CDCl3) δ 15.2, 15.3, 21.8, 27.7, 33.1, 33.2, 44.4, 46.5, 46.6, 46.7, 65.2, 65.3, 90.8, 90.9, 126.3, 126.3, 128.0, 138.9, 139.1, 144.3; mass spectrum (APCI): m/e (% relative intensity) 338.2 (M+H)+ (100), 254.1 (10).
Procedure with Tf2NH.
To a solution of vinyl hemiaminal 77 (52.0 mg, 0.205 mmol) and 4Å molecular sieve (100 mg) in anhyd CH2Cl2 (2.05 mL) was added a 0.5 M Tf2NH (0.41 mL, 0.205 mmol) solution in CH2Cl2 at -78 oC. The reaction mixture was stirred for 15 min under nitrogen atmosphere at the same temperature, and the progress of the reaction was monitored using TLC. Upon completion, the reaction was quenched via addition of pyridine. The resulting reaction mixture was filtered through a pad of CeliteTM and concentrated in vacuo. Further purification was performed by silica gel flash column chromatography [gradient eluent: 20:1 to 10:1 hexane/EtOAc] to afford 77 in a 3:1 ratio of diastereomers (68.2 mg, 98.5 %) as colorless oil.
77 (major diastereomer): Rf= 0.65 [33% EtOAc in hexanes]; 1H NMR (400 MHz, CDCl3) δ 0.89 (s, 3H), 0.90 (s, 3H), 1.03 (s, 3H), 1.08 (s, 3H), 1.62 (dd, J = 8.4 and 11.2 Hz, 1H), 1.80 (dd, J = 10.8 and 10.8 Hz, 1H), 2.08 (dd, J = 8.0, 10.0 and 10.0 Hz, 1H), 3.26 (ddd, J = 7.2, 7.2 and 7.2 Hz, 1H), 3.38 (ddd, J = 7.2, 7.2 and 11.6 Hz, 1H), 3.55 (ddd, J = 5.2, 7.2 and 11.6 Hz, 1H), 3.78 (ddd, J = 5.2, 7.2 and 7.6 Hz, 1H), 5.28 (d, J = 9.6 Hz, 1H), 7.32 (d, J = 8.0 Hz, 2H), 7.73 (d, J = 8.0 Hz, 2H); 13C NMR (100 MHz, CDCl3) δ 19.8, 21.8, 23.9, 24.5, 25.2, 33.8, 35.8, 40.4, 43.4, 46.3, 64.8, 93.4, 128.0, 130.1, 135.2, 144.2; mass spectrum (APCI): m/e (% relative intensity) 338.2 (M+H)+ (45), 284.1 (35), 254.1 (100).
Preparation and Gassman's Intramolecular [2 + 2] Cycloaddition of Vinyl Hemiaminal 80.
Vinyl Hemiaminal 80. To a solution of vinyl aldehyde trans-78 (0.202 g, 1.0 mmol) and (EtO)3CH (0.37 g, 2.5 mmol) in absolute ethanol (5.0 mL) was added NBS (3.54 mg, 0.02 mmol), and the resulting solution was stirred at room temperature. After completion of the reaction, a cold aqueous solution of NaOH (10%, 10 mL) was added and the mixture was extracted with Et2O (3 x 10 mL) and dried over anhydrous Na2SO4, evaporation of the solvent under reduced pressure gave the diethyl acetal 79, the product pure enough for the next step.
To a solution of above acetal and N-tosyl ethanolamine (0.215 g, 1.0 mmol) in dry toluene (20 mL) was added 4Å molecular sieves (1.0 g). The mixture was refluxed for 4h, cooled to room temperature and then EtOAc (50 mL) was added, and the solution was filtered. The filtrate was concentrated in vacuo and purified by flash chromatography [5:1 hexane:EtOAc] to afford vinyl hemiaminal 80 (0.28 g, 70% yield) as a liquid.
80: Rf = 0.25 [25% EtOAc in hexanes];1H-NMR (400MHz, CDCl3) δ 2.43 (s, 3H), 3.41-3.52 (m, 3H), 3.84-3.87 (m, 1H), 4.07-4.09 (m, 2H), 4.15 (dd, J = 1.2 and 6.0 Hz, 1H), 5.61 (dd, J = 0.8 and 4.8 Hz, 1H), 5.73 (ddt, J = 1.6, 3.2, and 15.6 Hz, 1H), 6.00 (ddt, J = 1.2, 5.2, and 15.2 Hz, 1H), 6.25 (dt, J = 6.0 and 16.0 Hz, 1H), 6.60 (d, J =16.0 Hz, 1H), 7.22-7.40 (m, 7H) , 7.73 (dt, J = 2.0 and 4.8 Hz, 2H); 13C NMR (100 MHz, CDCl3) δ 21.8, 46.4, 65.4, 69.5, 71.2, 90.0, 126.1, 126.7, 127.9, 128.0, 128.8, 130.1, 131.3, 132.8, 134.7, 136.9, 144.4.
To a solution of vinyl hemiaminal 80 (79.8 mg, 0.20 mmol) and 4Å molecular sieves (100 mg) in anhyd CH2Cl2 (2.0 mL) was added a 0.5 M Tf2NH (0.40 mL, 0.20 mmol) solution in CH2Cl2 at -78 oC. The reaction mixture was stirred for 15 min under nitrogen atmosphere at the same temperature, and the progress of the reaction was monitored using TLC. Upon completion, the reaction was quenched via addition of pyridine. The resulting reaction mixture was filtered through a pad of CeliteTM and concentrated in vacuo. Further purification was performed by silica gel flash column chromatography [gradient eluent: 5:1 to 2:1 hexane/EtOAc] to afford cyclobutane 71 (7.6 mg, 10 %) as a colorless oil.
81: Rf = 0.51 [33% EtOAc in hexanes]; 1H-NMR (400MHz, CDCl3) δ 2.37 (s, 3H), 2.50-2.52 (m, 1H), 3.08-3.13 (m, 3H), 3.30-3.40 (m, 3H), 3.58-3.69 (m, 3H), 3.92 (d, J = 9.6 Hz, 1H), 4.01 (d, J = 9.2 Hz, 1H), 5.11 (d, J = 9.6 Hz, 1H), 7.20-7.40 (m, 7H), 7.51-7.53 (m, 2H); 13C NMR (100 MHz, CDCl3) δ 21.7, 39.2, 41.6, 43.7, 44.8, 46.4, 64.6, 74.0, 74.3, 91.5, 126.7, 127.8, 127.9, 128.5, 128.8, 130.1, 134.3, 140.9, 144.4. HRMS-ESI m/e calcd for C22H26NO4S 400.1577, found 400.1599.
ACKNOWLEDGEMENTS
We thank ACS-PRF-AC for funding. We also thank The National Science Foundation [NSF - CHE1012198] for support of this work. We thank Dr. Vic Young and Ben Kucera at the University of Minnesota for providing X-ray structural analysis.
References
1. For some general reviews on cycloadditions, see: (a) See Chapters on cycloaddition reactions in Comprehensive Organic Synthesis, ed. by B. M. Trost and I. Fleming, Pergamon Press, 1991, Vol. 4 and Vol. 5; (b) W. Carruthers, Cycloaddition Reactions in Organic Synthesis Pergamon Press: Oxford and New York, 1990; (c) A. Padwa and A. Schoffstall, in Advances in Cycloaddition, ed. by D. P. Curran, JAI Press: Greenwich, CT, 1990, Vol. 2; (d) E. J. Corey, Angew. Chem. Int. Ed., 2002, 41, 1650. CrossRef
2. R. B. Woodward and R. Hoffmann, Angew. Chem., Int. Ed. Engl., 1969, 8, 781. CrossRef
3. M. T. Crimmins "Photochemical Cycloadditions," in Comprehensive Organic Synthesis, Vol. 5, ed. by B. M. Trost, I. Fleming, and G. Pattenden, Pergamon Press: New York, 1991, p. 123. CrossRef
4. J. D. Winkler, C. M. Bowen, and F. Liotta, Chem. Rev., 1995, 95, 2003. CrossRef
5. For an excellent review, see: T. Bach, Synthesis, 1998, 683, and references 1-15 cited within. CrossRef
6. (a) E. Lee-Ruff and G. Mladenova, Chem. Rev., 2003, 103, 1449; CrossRef (b) M. Lukina, Russ. Chem. Rev., 1963, 32, 635. CrossRef
7. H. N. C. Wong, K. L. Lau, and K. F. Tam, Top. Curr. Chem., 1986, 133, 83.
8. For recent intramolecular [2 + 2] cycloadditions using visible light and a ruthenium catalyst, see: (a) M. A. Ischay, M. E. Anzovino, J. Du, and T. P. Yoon, J. Am. Chem. Soc., 2008, 130, 12886; CrossRef (b) J. Du and T. P. Yoon, J. Am. Chem. Soc., 2009, 131, 14604; CrossRef (c) M. A. Ischay, Z. Lu, and T. P. Yoon, J. Am. Chem. Soc., 2010, 132, 8572. CrossRef
9. For a review on thermal [2 + 2] cycloaddition reactions see: J. E. Baldwin "Thermal Cyclobutane Ring Formation," in Comprehensive Organic Synthesis, Vol. 5, ed. by B. M. Trost, I. Fleming, and G. Pattenden, Pergamon Press: New York, 1991, p. 63. CrossRef
10. For reviews on metal mediated cycloadditions and cyclizations, see: (a) I. Nakamura and Y. Yamamoto, Chem. Rev., 2004, 104, 2127; CrossRef (b) J. A. Varela and C. Saá, Chem. Rev., 2003, 103, 3787; CrossRef (c) M. Rubin, A. W. Sromek, and V. Gervorgyan, Synlett, 2003, 2265; CrossRef (d) C. Aubert, O. Buisine, and M. Malacria, Chem. Rev., 2002, 102, 813; CrossRef (e) S. Saito and Y. Yamamoto, Chem. Rev., 2000, 100, 2901; CrossRef (f) H.-W. Frühauf, Chem. Rev., 1997, 97, 523; (g) I. Ojima, M. Tzamarioudaki, Z. Li, and R. J. Donovan, Chem. Rev., 1996, 96, 635; CrossRef (h) M. Lautens, W. Klute, and W. Tam, Chem. Rev., 1996, 96, 49. CrossRef
11. For recent examples of metal mediated [2 + 2] cycloadditions, see: (a) H. Ohara, T. Itoh, M. Nakamura, and E. Nakamura, Chem. Lett., 2001, 624; CrossRef (b) T.-G. Baik, A. L. Luis, L.-C. Wang, and M. J. Krische, J. Am. Chem. Soc., 2001, 123, 6716; CrossRef (c) M. W. Bouwkamp, A. C. Bowman, E. Lobkovsky, and P. J. Chirik, J. Am. Chem. Soc., 2006, 128, 13340. CrossRef
12. For recent examples, see: (a) R. F. Sweel, M. P. Schramm, and S. A. Kozimin, J. Am. Chem. Soc., 2004, 126, 7442; CrossRef (b) F. Marion, J. Coulomb, C. Courillon, L. Fensterbank, and M. Malacria, Org. Lett., 2004, 6, 1509; CrossRef (c) N. Riddell, K. Villeneuve, and W. Tam, Org. Lett., 2005, 7, 3681; CrossRef (d) S. Couty, C. Meyer, and J. Cossy, Angew. Chem. Int. Ed., 2006, 45, 6726. CrossRef
13. For recent reviews on allene cycloaddition chemistry, see: (a) S. Ma, Chem. Rev., 2005, 105, 2829; CrossRef (b) For a compendium on the chemistry of allenes, see: N. Krause and A. S. K. Hashmi, Modern Allene Chemistry; Wiley-VCH Verlag GmbH & Co. KGaA: Weinheim, 2004, Vol. 1 and 2; For some examples, see: (c) Y. Horino, M. Kimura, Y. Wakamiya, T. Okajima, and Y. Tamaru, Angew. Chem. Int. Ed., 1999, 38, 121; CrossRef (d) M. Kimura, Y. Horino, Y. Wakamiya, T. Okajima, and Y. Tamaru, J. Am. Chem. Soc., 1997, 119, 10869. CrossRef
14. D. J. Pasto, J. Am. Chem. Soc., 1979, 101, 37. CrossRef
15. (a) K. Inanaga, K. Takasu, and M. Ihara, J. Am. Chem. Soc., 2005, 127, 3668; CrossRef (b) M. B. Boxer and H. Yamamoto, Org. Lett., 2005, 7, 3127; CrossRef (c) K. Takasu, M. Ueno, K. Inanaga, and M. Ihara, J. Org. Chem., 2004, 69, 517; CrossRef (d) K. Narasaka, Y. Hayashi, H. Shimadzu, and S. Nihata, J. Am. Chem. Soc., 1992, 114, 8869; CrossRef (e) T. A. Engler, M. A. Letavic, and J. P. Reddy, J. Am. Chem. Soc., 1991, 113, 5068; CrossRef (f) S. Ahmad, Tetrahedron Lett., 1991, 32, 6997; CrossRef (g) Y. Ichikawa, A. Narita, A. Shiozawa, Y. Hayashi, and K. Narasaka, J. Chem. Soc., Chem. Commun., 1989, 1919; (h) Y. Hayashi and K. Narasaka, Chem. Lett., 1989, 793. CrossRef
16. E. Canales and E. J. Corey, J. Am. Chem. Soc., 2007, 129, 12686. CrossRef
17. K. Ishihara and K. Nakano, J. Am. Chem. Soc., 2007, 129, 8930. CrossRef
18. P. G. Gassman, S. P. Chavan, and L. B. Fertel, Tetrahedron Lett., 1990, 31, 6489. CrossRef
19. P. G. Gassman and A. C. Lottes, Tetrahedron Lett., 1992, 33, 157. CrossRef
20. (a) M. Harmata and P. Rashatasakhon, Tetrahedron, 2003, 59, 2371; CrossRef (b) M. Harmata, Tetrahedron, 1997, 53, 6235. CrossRef
21. For reviews on oxyallyl cation [4 + 3] cycloadditions, see: (a) M. Harmata, Acc. Chem. Res., 2001, 7, 595; CrossRef (b) H. M. L. Davies, in Advances in Cycloaddition, ed. by M. Harmata, JAI Press: 1998, Vol. 5, pp. 119-164; (c) F. G. West “Photocyclization and Photocycloaddition Reactions of 4- and 2-Pyrones.” In Advances in Cycloaddition; ed. by M. Lautens; JAI: Grennwich, 1997, Vol. 4, pp. 1-40; (d) J. H. Rigby and F. C. Pigge “[4 + 3] Cycloaddition Reactions.” Organic React. 1997, 51, pp. 351-478; (e) A. Hosomi and Y. Tominaga, In “[4 + 3] Cycloaddition.” Comprehensive Organic Synthesis, ed. by B. M. Trost, I. Fleming, and G. Pergamon, Oxford, 1991, Vol. 5, Chapter 5.1, pp. 593-615; (f) For an excellent review solely on heteroatom-stabilized oxyallyl cations, see: M. Harmata, Recent Res. Devel. in Organic Chem., 1997, 1, pp. 523-535; (g) H. M. R. Hoffmann, Angew. Chem. Int. Ed. Engl., 1973, 12, 819; CrossRef (h) H. M. R. Hoffmann and D. R. Joy, J. Chem. Soc. B, 1968, 1182; CrossRef (i) H. M. R. Hoffmann, Angew Chem. Int. Ed. Engl., 1984, 23, 1. CrossRef
22. For some recent examples, see; (a) T. Sakai, Y. Ishibashi, and M. Ohno, Tetrahedron Lett., 1982, 23, 1693; CrossRef (b) B. Föhlisch, D. Krimmer, E. Gehrlach, and D. Kashammer, Chem. Ber., 1988, 121, 1585; (c) D. H. Murray and K. Albizati, Tetrahedron Lett., 1990, 31, 4109; CrossRef (d) M. Harmata, S. Elomari, and C. J. Barnes, J. Am. Chem. Soc., 1996, 118, 2860, and references cited within. CrossRef
23. For a review, see; T. Mukaiyama and M. Murakami, Synthesis, 1987, 1043. CrossRef
24. For a review, see: A. Alexakis and P. Mangeney, Tetrahedron Asymmetry, 1990, 1, 477. CrossRef
25. For our previous communication on an intramolecular Gassman’s cationic [2 + 2] cycloaddition, see: C. Ko, J. B. Feltenberger, S. K. Ghosh, and R. P. Hsung, Org. Lett., 2008, 10, 1971. CrossRef
26. For a leading reference, see: H. V. Thulasiram, H. K. Erickson, and C. D. Poulter, Science, 2007, 316, 73. CrossRef
27. For citations on isolations recent natural products, see: (a) Pestalotiopsin A: M. Pulici, F. Sugawara, H. Koshino, J. Uzawa, S. Yoshida, E. Lobkovsky, and J. Clardy, J. Org. Chem., 1996, 61, 2122; (b) 6-hydroxypunctaporonins: S. T. Deyrup, D. C. Swenson, J. B. Gloer, and D. T. Wicklow, J. Nat. Prod., 2006, 69, 608; CrossRef (c) Artarborol: C. Fattorusso, E. Stendardo, G. Appendino, E. Fattorusso, P. Luciano, A. Romano, and O. Taglialatela-Scafati, Org. Lett., 2007, 9, 2377; CrossRef (d) Providencin: J. Marrero, A. D. Rodríguez, P. Baran, and R. G. Raptis, Org. Lett., 2003, 5, 2551; CrossRef (e) Biyouyanagin A: N. Tanaka, M. Okasaka, Y. Ishimaru, Y. Takaishi, M. Sato, M. Okamoto, T. Oshikawa, S. U. Ahmed, L. M. Consentino, and K.-H. Lee, Org. Lett., 2005, 7, 2997. CrossRef
28. T. Tsunoda, M. Suzuki, and R. Noyori, Tetrahedron Lett., 1980, 21, 1357. CrossRef
29. For studies on the acidity of HNTf2, see: (a) C. Thomazeau, H. Olivier-Bourbigou, L. Magna, S. Luts, and B. Gilbert, J. Am. Chem. Soc., 2003, 125, 5264, and references cited therein; CrossRef (b) J. Foropoulus and D. D. DesMarteau, Inorg. Chem., 1984, 23, 3720. CrossRef
30. C. Ko and R. P. Hsung, Organic Biomol. Chem., 2007, 7, 431. CrossRef
31. The isomerization study was performed later in our investigations on intramolecular Gassman’s [2 + 2] cycloadditions. While the times in this study for reaction completion were generally under 1 minute, our other reactions were monitored for completion by typical TLC analysis.
32. (a) J. Marrero, A. D. Rodríguez, P. Baran, R. G. Raptis, J. A. Sánchez, E. Ortega-Barria, and T. L. Capson, Org. Lett., 2004, 6, 1661; CrossRef (b) B. Doroh and G. A. Sulikowski, Org. Lett., 2006, 8, 903. CrossRef
33. R. A. Holton and J. R. Zoeller, J. Am. Chem. Soc., 1985, 107, 2124. CrossRef
34. (a) R. M. Beesley, C. K. Ingold, and J. F. Thorpe, J. Chem. Soc., 1915, 107, 1080; (b) C. K. Ingold, J. Chem. Soc., 1921, 119, 305; (c) C. K. Ingold, S. Sako, and J. F. Thorpe, J. Chem. Soc., 1922, 120, 1117.
35. For a preparation of FeCl3-SiO2, see: (a) S. P. Chavan and A. K. Sharma, Synlett, 2001, 667. CrossRef
36. We also investigated chiral 1,3-dioxolane acetal S12 derived from (S,S)-hydrobenzoin which gave a similar product distribution to 62, with poorer diastereoselectivity and yield of the desired cyclobutane S13.
37. (a) G. P. Brengel, C. Rithner, and A. I. Meyers, J. Org. Chem., 1994, 59, 5144; CrossRef (b) A. I. Meyers, M. A. Tschantz, and G. P. Brengel, J. Org. Chem., 1995, 60, 4359; CrossRef (c) M. D. Groaning, G. P. Brengel, and A. I. Meyers, J. Org. Chem., 1998, 63, 5517; CrossRef (d) M. D. Groaning and A. I. Meyers, Tetrahedron Lett., 1999, 40, 8071; CrossRef (e) M. D. Groaning, G. P. Brengel, and A. I. Meyers, Tetrahedron, 2001, 57, 2635. CrossRef
38. A. Bernardi, S. Cardani, T. Pilati, G. Poli, C. Scolastico, and R. Villa, J. Org. Chem., 1988, 53, 1600. CrossRef
39. B. Karimi, H. Seradj, and G. Ebrahimian, Synlett, 1999, 1456. CrossRef