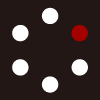
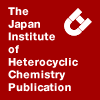
HETEROCYCLES
An International Journal for Reviews and Communications in Heterocyclic ChemistryWeb Edition ISSN: 1881-0942
Published online by The Japan Institute of Heterocyclic Chemistry
e-Journal
Full Text HTML
Received, 23rd July, 2011, Accepted, 22nd August, 2011, Published online, 31st August, 2011.
DOI: 10.3987/COM-11-S(P)85
■ Enantioenriched Formation of Synthons of 1,5-Hexadiene Bis-epoxides for Construction of Nonracemic cis- and trans-2,5-Disubstituted Tetrahydrofurans
David R. Williams* and Ramkrishna De
Department of Chemistry, Indiana University, 800 East Kirkwood Avenue, Bloomington, IN 47405-7102, U.S.A.
Abstract
A pathway for the synthesis of enantioenriched bis-epoxides derived from 2,6-heptadien-1-ol is described. Use of the Katsuki titanium salalen catalyst affords high asymmetric induction for mild epoxidation of the monosubstituted alkene as the key step. Regioselective nucleophilic additions to these chiral bis-epoxides lead to a stereocontrolled preparation of nonracemic cis- and trans-2,5-disubstituted tetrahydrofurans.In the course of our studies for natural product total synthesis, we have previously developed a number of strategies for the stereocontrolled formation of substituted tetrahydrofurans (THF’s), which are frequently embedded within the molecular architectures of microbial and marine metabolites.1 Unabated interest in the stereoselective synthesis of THF’s continues,2 and these activities have, in part, been encouraged by studies of biomimetic cascade reactions from polyepoxide precursors to give complex THF’s and related oxacyclic systems.3 Our studies leading to the total synthesis of (+)-breynolide documented an early example of this concept by formation of the bis-epoxide 1 for sequential ring-opening reactions to produce the advanced intermediate tetrahydrothiophene-ketal 2 using sodium hydrogen sulfide.4 However, issues of enantiofacial and diastereofacial control in the formation of polyepoxide precursors remains a particularly challenging problem. The reagents and the conditions required for the preparation of nonracemic polyepoxides present a number of difficulties owing to the inherent reactivity of these oxirane systems with Lewis acids and nucleophilic Lewis bases. Previous studies have focused on strategies for the enantiocontrolled preparation of 1,5-hexadiene bis-epoxides as synthons toward the construction of nonracemic THF’s.5 For example, the Jacobsen hydrolytic kinetic resolution of 1,5-hexadiene bis-epoxide 3 has provided (2R,5R)-4 in 21% yield with >95% ee.6,7 The corresponding (2S,5S)-ent-4 is available from the chiral pool via a seven-step route using D-mannitol.8
In these cases, direct methods resulting in asymmetric induction via reagent-controlled epoxidations have generally been problematic for monosubstituted aliphatic alkenes.9 This aspect is a significant barrier for successful applications leading to nonracemic cis- and trans-2,5-disubstituted THF’s because a generalized synthetic solution relies upon the selective nucleophilic opening of a terminal oxirane. The intermediate, alkoxide initiates the cascading internal backside displacement leading to tetrahydrofuran formation as illustrated in 5.6 Herein, we describe an effective means for the preparation of enantioenriched bis-epoxides of 2,6-heptadien-1-ol and subsequent reactions leading to the selective formation of 2,5-disubstituted THF’s.
For our purpose, we required the use of (Z)-2,6-heptadien-1-ol (8), which was readily prepared via the low temperature Wittig reaction of aldehyde 6 and phosphonium salt 7 followed by DDQ deprotection (Scheme 1). Sharpless asymmetric epoxidation of 8 with D-(–)-diethyl tartrate (DET) provides the expected epoxyalcohol as the major product of a 91:9 ratio of enantiomers. The ratio of enantiomers was determined by a modified Mosher ester analysis.10 Protection of the primary alcohol as the corresponding tert-butyldiphenylsilyl (TBDPS) or tert-butyldimethylsilyl (TBS) ethers 9a and 9b proceeded in high yields, as well as conversion into the p-methoxybenzyl (PMB) ether 9c (NaH; PMBCl; TBAI; THF/DMF; (4:1 by volume), 81% yield), and this step provided materials with favorable solubility for subsequent manipulations. Asymmetric oxidation of the terminal alkene of each of the derivatives 9a, b, c, was accomplished using 2 mol% of the Katsuki titanium salalen catalyst,11 (S,S)-KAT-10, in methylene chloride by the dropwise addition of 30% aq. H2O2 (1.5 eq) at room temperature. The reaction proceeds over 24 h with continuous stirring, and results in a stereoselective epoxidation with production of the nonracemic bis-epoxide 11 as the principle component of a highly enriched mixture of epoxide isomers (65% yield), without the formation of other byproducts.
Starting alkene is routinely recovered by flash chromatography (20–25% yield) and is recycled with additional catalyst 10 and oxidant to provide an 80% overall conversion to 11 after two cycles. The Katsuki catalyst is slowly degraded during the oxidation, and at 24 h, sluggish reactions are not revived by the introduction of small quantities of 10 and aqueous hydrogen peroxide. The level of asymmetric induction achieved by the Katsuki catalyst was evaluated via the 13C NMR data of the bis-epoxide products and displayed a 90:10 ratio of diastereomers (based on a measure of signal peak intensities).12 Additionally, we have used the Jacobsen hydrolytic kinetic resolution (HKR)13 employing (R,R)-Co(II)(salen)OAc with 11a to enrich the diastereomeric purity (dr 94:6).12
We note that the order of the epoxidation steps is significant. In this regard, our studies have also examined opportunities for the asymmetric epoxidation of optically active (R,Z)-5-(oxiran-2-yl)pent-2-en-1-ol which was prepared from D-malic acid (Aldrich). Directed oxidations via the Sharpless asymmetric epoxidation or VO(acac)2 and tBuOOH led to rapid decomposition owing to the Lewis acidity of these reagents. The Jacobsen epoxidation14 of ether derivatives of this substrate using the (R,R)-salen-Mn(III) catalyst led to the desired bis-epoxides in low yield with poor diastereoselectivity.
This strategy offers a modular approach for the synthesis of THF units which is amenable to the preparation of all stereochemical isomers within a series of nonracemic compounds by simple variations of: (a) the E/Z-geometry of the starting allylic alcohol; (b) the choice of D/L-tartrate; and (c) the use of the (R,R)- or (S,S)-Katsuki catalyst. As a proof of principle, we have also prepared the
diastereomeric bis-epoxide 12 from 8 by using L-DET for the Sharpless asymmetric epoxidation, and the use of the (S,S)-Katsuki catalyst, ent-10, with alkenes 9a and 9c afforded bis-epoxides 13a and 13c. These epoxides were obtained with similar stereoselectivity and yield as described in Scheme 1. A number of results featuring the formation of chiral, nonracemic THF’s by the anticipated 5-exo-tet cyclization initiated by site-selective nucleophilic attack are shown in Table 1. In each instance, a small quantity of THF diastereomer (5–8%) was separated from the major product by flash silica gel chromatography.15 The examples of Table 1 are chosen to illustrate the extension of the concept to a variety of common nucleophiles, including alkynyl, alkenyl and alkyl carbanions which lead to the preparation of THF’s 14–23. Yields of these products have not been optimized, and in some cases, small quantities of epoxyalcohol have been identified as side products stemming from incomplete cyclizations. However, in the examples of copper(I)-catalyzed reactions (entries 1, 2, 8 and 9), we have noted the formation of byproducts arising from nucleophilic opening at C3 of the epoxy TBDPS ether in addition to the desired attack at the terminal oxirane. Deprotection of the products 14–23 provides for the formation of 1,2-diols which may undergo oxidative cleavage to yield the corresponding tetrahydrofuran carboxaldehydes as substrates for subsequent nucleophilic additions. Thus, our chiral bis-epoxides serve as nonracemic synthons of 1,5-hexadiene bis-epoxides which afford regiocontrolled introduction of the nucleophilic attack leading to THF formation.
In summary, the Katsuki oxidation facilitates the asymmetric epoxidation of monosubstituted alkene in the presence of nonracemic titanium salalen catalyst. This key transformation allows for the preparation of sensitive, enantioenriched bis-epoxides derived from 2,6-heptadien-1-ol following the Sharpless asymmetric epoxidation of the allylic alcohol moiety. We have shown that bis-epoxides 11, 12 and 13 are useful in the synthesis of nonracemic cis- and trans-2,5-disubstituted tetrahydrofurans via initial site-selective nucleophilic attack at the less hindered oxirane. In this fashion, our bis-epoxides serve as chiral synthons that provide a flexible and general strategy toward the formation of substituted THF’s for efficient incorporation into more complex molecular arrays.
ACKNOWLEDGEMENTS
We gratefully acknowledge support from Indiana University (Faculty Research Support Program) and we thank the National Institutes of Health (GM042897) for partial support of this study.
References
1. For examples of various methods and strategies: D. R. Williams and J. G. Phillips, Tetrahedron, 1986, 42, 3013; CrossRef D. R. Williams and F. H. White, J. Org. Chem., 1987, 52, 5067; CrossRef D. R. Williams, Y. Harigaya, J. L. Moore, and A. D’sa, J. Am. Chem. Soc., 1984, 106, 2641; CrossRef D. R. Williams and K. G. Meyer, Org. Lett., 1999, 1, 1303; CrossRef D. R. Williams, J. Grote, and Y. Harigaya, Tetrahedron Lett., 1984, 25, 5231. CrossRef
2. J. P. Wolfe and M. B. Hay, Tetrahedron, 2007, 63, 261. CrossRef
3. For a general review and some specific examples: I. Vilotijevic and T. F. Jamison, Angew. Chem. Int. Ed., 2009, 48, 5250; CrossRef Z. Xiong, R. Busch, and E. J. Corey, Org. Lett., 2010, 12, 1512; CrossRef T. R. Hoye and J. C. Suhadolnik, Tetrahedron, 1986, 42, 2855; CrossRef Y. Morimoto, T. Okita, and H. Kambara, Angew. Chem. Int. Ed., 2009, 48, 2538. CrossRef
4. D. R. Williams, Paul A. Jass, H.-L. A. Tse, and R. D. Gaston, J. Am. Chem. Soc., 1990, 112, 4552. CrossRef
5. S. Rigolet, I. McCort, and Y. Le Merrer, Tetrahedron Lett., 2002, 43, 8129; CrossRef M. E. Jung and O. Kretschik, J. Org. Chem., 1998, 63, 2975; CrossRef F. E. McDonald, F. Bravo, X. Wang, X. Wei, M. Toganoh, J. R. Rodriguez, B. Do, W. A. Neiwert, and K. I. Hardcastle, J. Org. Chem., 2002, 67, 2515; CrossRef J. A. Marshall and H. R. Chobanian, Org. Lett., 2003, 5, 1931. CrossRef
6. G. J. Florence and R. Cadou, Tetrahedron Lett., 2008, 49, 6784. CrossRef
7. K. C. O’Brien, E. A. Colby, and T. F. Jamison, Tetrahedron, 2005, 61, 6243. CrossRef
8. N. Machinaga and C. Kibayashi, Synthesis, 1992, 989; CrossRef M. E. Maier and S. Reuter, Liebigs Ann./Receuil, 1997, 2043.
9. For examples: V. B. Valadkar, G. L. Tembe, R. N. Ram, and H. S. Rama, Catal. Lett., 2003, 90, 91; CrossRef A. Berkessel and M. Frauenkran, J. Chem. Soc. Perkin Trans. 1, 1997, 1, 2265; CrossRef H. Tian, X. She, J. Xu, and Y. Shi, Org. Lett., 2001, 3, 1929. CrossRef
10. J. A. Dale and H. S. Mosher, J. Am. Chem. Soc., 1973, 95, 512. CrossRef
11. For leading references: Y. Sawada, K. Matsumoto, and T. Katsuki, Angew. Chem. Int. Ed., 2007, 46, 4559; CrossRef D. Xiong, M. Wu, S. Wang, F. Li, C. Xia, and W. Sun, Tetrahedron: Asymmetry, 2010, 21, 374; CrossRef A. Berkessel, M. Brandenburg, and M. Schäfer, Adv. Synth. Catal., 2008, 350, 1287. CrossRef
12. Our reaction pathway independently introduces two elements of chirality and produces two pairs of enantiomeric diastereoisomers A, B, C and D, shown below. A ratio of isomers (A:B:C:D ratio 80:8:11:1) is estimated based upon the enantiofacial selectivity of the Sharpless epoxidation (91:9 er) and a diastereofacial preference of the Katsuki oxidation (88:12). This calculation predicts a diastereomer ratio (A+D:B+C) of dr 81:19. Our experimental observations (dr 90:10) may reflect the degree of uncertainty introduced in the measurement of peak heights in the decoupled 13C NMR spectrum or unintended enrichment as a result of flash chromatography of the bis-epoxide. On the other hand, the NMR determination of the A:B ratio following the application of HKR to the bis-epoxides of 11a is in close agreement with prediction (dr 91:9). Based on this assessment, the optical purity of 11a (A and D are enantiomers) is 98% ee.
13. For a leading reference: S. E. Schaus, B. D. Brandes, J. F. Larrow, M. Tokunaga, K. B. Hansen, A. E. Gould, M. E. Furrow, and E. N. Jacobsen, J. Am. Chem. Soc., 2002, 124, 1307. CrossRef
14. W. Zhang, J. L. Loebach, S. R. Wilson, and E. N. Jacobsen, J. Am. Chem. Soc., 1990, 112, 2801. CrossRef
15. The modified Mosher ester analysis was used to evaluate the optical purity of our products 14–23 and these NMR experiments showed no evidence for the presence of enantiomeric material. Based on the limitations of detection, we assume an er ≥ 96:4. The amount of the minor diastereomer THF is in line with our expectations (see reference 12) based on similar yields as in Table 1.