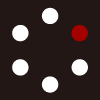
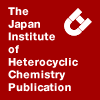
HETEROCYCLES
An International Journal for Reviews and Communications in Heterocyclic ChemistryWeb Edition ISSN: 1881-0942
Published online by The Japan Institute of Heterocyclic Chemistry
e-Journal
Full Text HTML
Received, 30th June, 2011, Accepted, 8th August, 2011, Published online, 10th August, 2011.
DOI: 10.3987/COM-11-S(P)71
■ Asymmetric Synthesis of Isoquinuclidine by Diels-Alder Reaction of 1,2-Dihydropyridine and Chiral Dienophile Utilizing a Chiral Lewis Acid
Masafumi Hirama, Chonticha Suttibut, N. D. M. Romauli Hutabarat, Chigusa Seki,* Noritaka Sakuta, Takefumi Tsuchiya, Yoshihito Kohari, Hiroto Nakano, Koji Uwai, Nobuhiro Takano, Mitsukuni Yasui, Yuko Okuyama, Kenichi Osone, Mitsuhiro Takeshita, and Haruo Matsuyama*
Department of Applied Chemistry, Graduate School of Engineering, Muroran Institute of Technology, 27-1 Mizumoto, Muroran, Hokkaido 050-8585, Japan
Abstract
The asymmetric Diels-Alder reaction of 1-phenoxycarbonyl-1,2-dihydropyridine 1 with 3-acryloyl (4S)-4-benzyl-1,3-oxazolidin-2-one (4S)-2 {or (4R)-2} in the presence of Ti-TADDOLate 4 as a chiral Lewis acid afforded the chiral isoquinuclidine derivative endo-(4’S)-3 in high yield (99%) with high diastereoselectivity (up to 92% d.e.). The reaction exhibits a strong match-mismatch effect. The stereochemistry of endo-(4’S)-3 was established to be (1R, 4S, 7R) and a reaction mechanism is proposed.The isoquinuclidines (2-azabicyclo[2.2.2]octane ring systems) are found in natural products such as iboga-type indole alkaloids which have varied and interesting biological properties (Figure 1).1 (+)-Catharanthine is of interest because of its eminent role as a biogenetic as well as a synthetic precursor of the antitumor alkaloids, vinblastine and vincristine.1 (–)-Ibogaine is the medicine for alcohol dependence which has bearing on the ibogamine skeleton.2 Furthermore, isoquinuclidines are valuable intermediates in the synthesis of other alkaloids,3 and in medicinal chemistry such as oseltamivir.4,5 The most promising method for the synthesis of isoquinuclidine derivatives is the Diels-Alder (D-A) reaction between 1,2-dihydropyridines and dienophiles.
In the asymmetric synthesis of isoquinuclidines, the diastereoselective cycloadditions using 1,2-dihydropyridines or dienophiles having a chiral auxiliary have been reported.6 The D-A reaction of 1,2-dihydropyridines and N-acryloyl (1S)-2,10-camphorsultam in the presence of Lewis acid, such as titanium tetrachloride, zirconium tetrachloride, and hafnium tetrachloride, afforded the endo-cycloaddition product (chiral isoquinuclidine derivatives) in good yield with excellent diastereoselectivity (up to 98% d.e.).6f Recently, its catalytic enantioselective synthesis was also reported.7 In order to synthesize the chiral isoquinuclidines, we adopted the asymmetric D-A reaction catalyzed by a chiral Lewis acid. We report the study on the synthesis of the chiral isoquinuclidines by D-A reaction of 1,2-dihydropyridine 1 with chiral dienophile 2 having a chiral auxiliary in the presence of Lewis acid. We investigated the two ways of the asymmetric D-A reactions. One is the reaction of 1-phenoxycarbonyl-1,2-dihydropyridine 18 and 3-acryloyl- (4S)-4-benzyl-1,3-oxazolidin-2-one (4S)-2 {or 3-acryloyl-(4R)-4-benzyl-1,3-oxazolidin-2-one (4R)-2}9 using Lewis acid and the other is the reaction of 1 and (4S)-2 {or (4R)-2} using Ti-TADDOLate 4 as a chiral Lewis acid. Though it is reported that 1,2-dihydropyridine is unstable for Lewis acid,6d our reaction system afforded chiral isoquinuclidines in good yields with high diastereoselectivity (up to 92% d.e.). The absolute stereochemistry of the cycloaddition product endo-(4’S)-3 was determined to be (7R) by conversion of endo-(4’S)-3 to the known benzyl ester (7R)-5.
We first examined the D-A reaction of 1-phenoxycarbonyl-1,2-dihydropyridine 1 and 3-acryloyl- (4S)-4-benzyl-1,3-oxazolidin-2-one (4S)-2 using Lewis acids such as dichlorotitanium diisopropoxide, zirconium tetrachloride, hafnium tetrachloride, and scandium trifluoromethane- sulfonate (Scheme 1). These D-A reactions were carried out in dichloromethane at 0 oC for 24 hours in the presence of molecular sieves 4A and the results are summarized in Table 1. The D-A reaction of 2 equiv. of 1,2-dihydropyridine 1 and 1 equiv. of (4S)-2 using 2 equiv. of Ti(i-PrO)2Cl2 was carried out at 0 oC for 24 hours to afford the cycloaddition product endo-(4’S)-3 in 99% yield with 63% d.e. and the product was only endo isomer (Table 1, Entry 1). In the D-A reaction of 2 equiv. of 1 and (4S)-2 using 1 equiv. of ZrCl4, only endo-(4’S)-3 was obtained in 42% yield with 48% d.e. (Entry 2). Similarly, D-A reaction of 2 equiv. of 1 and (4S)-2 using 1 equiv. of HfCl4, only endo-(4’S)-3 was afforded in 73% yield with 59% d.e. (Entry 4). The D-A reacton of 2 equiv. of 1 and 1 equiv. of (4S)-2 using 1 equiv. of Sc(OTf)3 gave only endo-(4’S)-3 in 99% yield with 52% d.e. (Entry 6). However, in the D-A reaction of 3 equiv. of 1 and (4S)-2 using 1 equiv. of ZrCl4, the yield of endo-(4’S)-3 increased to 72% yield with 57% d.e. (Entry 3). Similarly, in the D-A reaction of 3 equiv. of 1 and (4S)-2 using 1 equiv. of HfCl4, the yield of endo-(4’S)-3 increased to 94% yield with 43% d.e. (Entry 5). As a result, the chemical yield of endo-(4’S)-3 in the D-A reaction of 1 and (4S)-2 was good to high, however, the diastereoselectivity of endo-(4’S)-3 was moderate (43% d.e. to 63% d.e.).
In order to investigate the additional effect of chiral Lewis acid, the chiral 1,4-diol (TADDOL)11 {TADDOL: (2R,3R)-2,3-O-isopropylidene-1,1,4,4-tetraphenyl-1,2,3,4-butanetetrol, (2R,3R)-2,3-
O-(1-phenylethylidene)-1,1,4,4-tetraphenyl-1,2,3,4-butanetetrol, and (4R,5R)-α,α,α’,α’,2,2-hexa- phenyl-4,5-dimethanol-1,3-dioxalone12} was employed as a chiral auxiliary (Scheme 2).10,11 Chiral titanium complex Ti-TADDOLates 4 {4a (R1= R2= CH3), 4b (R1= Ph, R2= CH3), and 4c (R1= R2= Ph)} was prepared from TADDOL and dichlorotitanium diisopropoxide (ratio, 1 : 1) in the presence of molecular sieves 4A at room temperature (Scheme 2).11 In order to test the possibility of match-mismatch effect on the diastereoselectivity of D-A cycloaddition product endo-3, the reaction of 1,2-dihydropyridine 1 and (4S)-2 {or (4R)-2} in the presence of Ti-(2R,3R)-TADDOLate 4 was carried out in toluene at 0 oC for 24 h and the results are summarized in Table 2. In the D-A reaction of 2 equiv. of 1 and 1 equiv. of (4S)-2 using 0.3 equiv. of 4a, only endo-(4’S)-3 was obtained in 96% yield with 87% d.e. (Table 2, Entry 1). In the D-A reaction of 2 equiv. of 1 and (4S)-2 using 0.3 equiv. of 4b, only endo-(4’S)-3 was obtained in 99% yield with 92% d.e. (Entry 2).13 The D-A reaction of 2 equiv. of 1 and (4S)-2 using 0.3 equiv. of 4c afforded only endo-(4’S)-3 in 99% yield with 88% d.e. (Entry 3). However, in the D-A reactions of 2 equiv. of 1 and 1 equiv. of (4R)-2 using 0.3 equiv. of 4a-c, both the chemical yield (51% to 73%) and the diastereoselectivity (68% d.e. to 70% d.e.) of endo-(4’R)-3 are lower than those of endo-(4’S)-3 produced from the D-A reaction of 1 and (4S)-2 using 4 (Entries 4, 5, and 6). As a result, the combination of Ti-(2R,3R)-TADDOLate 4 and (4S)-2 was a matched pair to give (7R)-3 in 96% to 99% yield (up to 92% d.e.) (Table 2, Entries 1, 2, and 3), while the combination of Ti-(2R,3R)-TADDOLate 4 and (4R)-2 was a mismatched pair to afford (7S)-3 in 51% to 73% yield (up to 70% d.e.) (Entries 4, 5, and 6).
The absolute stereochemistry of the endo-(4’S)-3 was established to be (1R, 4S, 7R) by comparing the sign of the optical rotation with the literature value of (7R)-benzyl ester 5 {lit., 2a [α]D -59.92o (c 2.52, CHCl3), 97% e.e.} as follows: the reaction of the endo-(4’S)-3 (97% d.e.) with benzyl alcohol using n-BuLi as a base (PhCH2OLi) in THF afforded the corresponding (7R)-benzyl ester 5 {[α]D -81.4o (c 0.57, CHCl3), 97% e.e.} in 65% yield (Scheme 3).14
On the other hand, the absolute stereochemistry of isoquinuclidine derivative endo-(4’R)-3, which was obtained from the reaction of 1 and (4R)-2 in the presence of Ti-TADDOLate 4b, has been established to be (1S, 4R, 7S).
Based on the X-ray structure of complex of Ti-(2R,3R)-TADDOLate 4a with 3-[(E)-cinnamoyl]-1,3-oxazolidin-2-one reported by Jφrgensen et al.14, we considered the reaction mechanism of the asymmetric D-A reaction of 1,2-dihydropyridine 1 and (4S)-2 in the presence of Ti-(2R,3R)-TADDOLate 4b (Figure 2). As shown in Figure 2, in the complex of matched pair {4b and (4S)-2} 1,2-dihydropyridine 1 can approach predominantly from the si face to give (7R)-3 (up to 92% d.e.) since the re face of the acryloyl group on the dienophile is shielded by the benzyl group of dienophile (4S)-2 and the phenyl group at pseudoequatorial position of Ti-(2R,3R)-TADDOLate 4b. On the other hand, in the complex of mismatched pair {4b and (4R)-2} 1,2-dihydropyridine 1 can approach from the re face to give (7S)-3 (up to 70% d.e.) since the si face of the acryloyl group on the dienophile is shielded by the benzyl group of dienophile (4R)-2 in the complex of Ti-(2R,3R)-TADDOLate 4b. In the complex of mismatched pair {4b and (4R)-2}, the re face of the acryloyl group on the dienophile is also shielded by phenyl group at pseudoequatorial position of Ti-(2R,3R)-TADDOLate 4b. Consequently, it is assumed that both the chemical yield and the diastereoselectivity of (7S)-3 are lower than those of (7R)-3 produced from the matched pair {4b and (4S)-2}.
In conclusion, we have accomplished the synthesis of the chiral isoquinuclidine derivative endo-(7R)-3 {or endo-(7S)-3} by D-A reaction of 1,2-dihydropyridine 1 with chiral dienophile (4S)-2 {or (4R)-2} using Ti-TADDOLate 4 as a chiral Lewis acid, respectively. In the D-A reaction, the combination of Ti-TADDOLate 4 and chiral dienophile (4S)-2 {or (4R)-2} affected the chemical yield and the diastereoselectivity of the chiral isoquinuclidine derivative endo-(7R)-3 {or endo-(7S)-3}.
References
1. (a) M. E. Kuehne and I. Marko, ‘The Alkaloids. Antitumor Bisindole Alkaloids from Catharanthus roseus (L.): Syntheses of Vinblastine-type Alkaloids.,’ Vol. 37, ed. by A. Brossi and M. Suffness, Academic Press, Inc, San Diego, 1990, pp. 77-131; CrossRef (b) P. Popik and P. Skolnick, ‘The Alkaloids. Chemistry and Biology: Pharmacology of Ibogaine and Ibogaine-related Alkaloids’, Vol. 52, ed. by G. A. Cordell, Academic Press, Inc, San Diego, 1999, pp. 197-231; (c) M. O. F. Khana, M. S. Levid, C. R. Clarkc, S. Y. Ablordeppeya, S.-J. Lawd, N. H. Wilsone, and R. F. Bornef, Stud. Nat. Prod. Chem., 2008, 34, 753. CrossRef
2. (a) H. Nakano, N. Tsugawa, K. Takahashi, Y. Okuyama, and R. Fujita, Tetrahedron, 2006, 62, 10879; CrossRef (b) H. Nakano, N. Tsugawa, and R. Fujita, Tetrahedron Lett., 2005, 46, 5677; CrossRef (c) D. Y. He, N. N. McGough, A. Ravindranathan, J. Jeanblanc, M. L. Logrip, K. Phamluong, P. H. Janak, and D. Pon, J. Neurosci., 2005, 25, 619. CrossRef
3. S. F. Martin, H. Rueger, S. A. Williamson, and S. Grzejszczak, J. Am. Chem. Soc., 1987, 109, 6124. CrossRef
4. (a) N. Satoh, T. Akiba, S. Yokoshima, and T. Fukuyama, Tetrahedron, 2009, 65, 3239; CrossRef (b) Y.-Y. Yeung, S. Hong, and E. J. Corey, J. Am. Chem. Soc., 2006, 128, 6310; CrossRef (c) K. Yamatsugu, L. Yin, S. Kamijo, Y. Kimura, M. Kanai, and M. Shibasaki, Angew. Chem. Int. Ed., 2009, 48, 1070; CrossRef (d) H. Ishikawa, T. Takai, and Y. Hayashi, Angew. Chem. Int. Ed., 2009, 48, 1304. CrossRef
5. G. R. Krow, O. H. Cheung, Z. Hu, Q. Huang, J. Hutchinson, N. Liu, K. T. Nguyen, S. Ulrich, J. Yuan, Y. Xiao, D. M. Wypij, F. Zuo, and P. J. Carroll, Tetrahedron, 1999, 55, 7747. CrossRef
6. (a) Y. Matsumura, Y. Nakamura, T. Maki, and O. Onomura, Tetrahedron Lett., 2000, 41, 7685; CrossRef (b) D. C. dos Santos, R. P. de Freitas Gil, L. Gil, and C. Marazano, Tetrahedron Lett., 2001, 42, 6109; CrossRef (c) C. Marazano, Y. Yannic, M. Mehmandoust, and B. C. Das, Tetrahedron Lett., 1990, 31, 1995; CrossRef (d) C. Kouklovsky, A. Pouilhes, and Y. Langlois, J. Am. Chem. Soc., 1990, 112, 6672; CrossRef (e) M. Hirama, Y. Kato, C. Seki, H. Matsuyama, N. Oshikiri, and M. Iyoda, Chem. Lett., 2008, 37, 924; CrossRef (f) M. Hirama, Y. Kato, C. Seki, H. Nakano, M. Takeshita, N. Oshikiri, M. Iyoda, and H. Matsuyama, Tetrahedron, 2010, 66, 7618. CrossRef
7. (a) Pd-POZ catalyst: see Ref. 2a; (b) Cr(III) salen complex catalyst: N. Takenaka, Y. Huang, and V. H. Rawal, Tetrahedron, 2002, 58, 8299. CrossRef
8. F. W. Fowler, J. Org. Chem., 1972, 37, 1321. CrossRef
9. (a) G.-J. Ho and D. J. Mathre, J. Org. Chem., 1995, 60, 2271; CrossRef (b) D. A. Evans, K. T. Chapman, and J. Bisaha, J. Am. Chem. Soc., 1988, 110, 1238. CrossRef
10. D. Seebach, A. K. Beck, and A. Heckel, Angew. Chem. Int. Ed. Engl., 2001, 40, 92. CrossRef
11. (a) K. Narasaka, N. Iwasawa, M. Inoue, T. Yamada, M. Nakashima, and J. Sugimoto, J. Am. Chem. Soc., 1989, 111, 5340; CrossRef (b) K. Mikami, Y. Motoyama, and M. Terada, J. Am. Chem. Soc., 1994, 116, 2812. CrossRef
12. (a) B. M. Smith and A. E. Graham, Tetrahedron Lett., 2006, 47, 9317; CrossRef (b) J. Irurre, C. Alonso-Alija, J. F. Piniella, and A. Alvarez-Larena, Tetrahedron: Asymmetry, 1992, 3, 1591. CrossRef
13. Cycloaddition of 1-phenoxycarbonyl-1,2-dihydropyridine 1 with dienophile (4S)-2 in the presence of Ti-TADDOLate 4b: To the toluene (3 mL) solution of TADDOL (R1 = Ph, R2 = Me) (79 mg, 0.15 mmol) and molecular sieves 4A (200 mg) was added Ti(i-PrO)2Cl2 (36 mg, 0.15 mmol) at room temperature and the solution was stirred for 1 h under nitrogen. Then (4S)-2 (116 mg, 0.50 mmol) was added and the solution was stirred at room temperature for 30 min, and the solution was cooled to 0 oC. To this solution was added the toluene (2 mL) solution of 1-phenoxycarbonyl-1,2-dihydropyridine 1 (201 mg, 1.00 mmol) and the solution was stirred at 0 oC for 24 h. Then saturated NaHCO3 and water were added and the product was extracted with chloroform. The organic layer was dried over anhydrous sodium sulfate and filtered. After purification of crude product by column chromatography on silica gel (20% ethyl acetate in hexane), the endo-(4’S)-3 was obtained in 99% yield (214 mg, 0.495 mmol) with 92% d.e. endo-(4’S)-3: [α]D –58.3o (c 0.50, CHCl3). 1H NMR [ppm] (500 MHz, CDCl3, 20 oC): δ 1.54-1.59 (0.5H, m), 1.72-1.77 (0.5H, m), 2.14-2.19 (0.5H, m), 2.31-2.36 (0.5H, m), 2.71 (1H, dd, J=13.3, 9.8 Hz), 2.89-2.93 (1H, m), 3.09 (0.5H, td, J=10.6, 2.6 Hz), 3.22 (0.5H, td, J=10.4, 2.5 Hz), 3.29 (1H, d, J=13.3 Hz), 3.40 (0.5H, dd, J=10.6, 1.9 Hz), 3.56 (0.5H, dd, J=10.3, 1.9 Hz), 4.13-4.16 (2H, m), 4.21-4.29 (1H, m), 4.52-4.60 (1H, m), 5.17-5.19 (0.5H, m), 5.24-5.26 (0.5H, m), 6.48-6.51 (1H, m), 6.54-6.62 (1H, m), 7.12-7.14 (1H, m), 7.16-7.21 (4H, m), 7.24-7.28 (1H, m), 7.30-7.37 (4H, m); 13C NMR [ppm] (125 MHz, CDCl3, 20 oC): δ 28.58, 30.84, 37.79, 45.17, 46.66, 47.75, 55.60, 66.32, 121.81, 125.22, 127.42, 129.04, 129.23, 129.26, 129.38, 129.42, 130.73, 132.45, 133.57, 134.86, 135.20, 151.32, 152.65, 153.44, 173.01.
14. (7R)-benzyl ester 5 (97% e.e.): The enantiomeric excess (97% e.e.) of (7R)-benzyl ester 5 {[α]D -81.4o (c 0.57, CHCl3)} as shown in Scheme 3 was determined by HPLC analysis using a DAICEL chiralcel AD-H column; eluent 5% 2-propanol/hexane, flow rate 0.8 mL/min, tR = 28.7 min (minor), 32.0 min (major). (7S)-benzyl ester 5 (99% e.e.): [α]D +90.4o (c 0.71, CHCl3); [α]D +74.46o (c 0.39, DMSO); (7R)-benzyl ester 5 (97% e.e.): lit.2b [α]D -59.92o (c 2.52, DMSO).
15. K. V. Gothelf, K. R. G. Hazell, and K. A. Jφrgensen, J. Am. Chem. Soc., 1995, 117, 4435. CrossRef