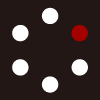
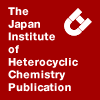
HETEROCYCLES
An International Journal for Reviews and Communications in Heterocyclic ChemistryWeb Edition ISSN: 1881-0942
Published online by The Japan Institute of Heterocyclic Chemistry
e-Journal
Full Text HTML
Received, 22nd June, 2011, Accepted, 10th August, 2011, Published online, 17th August, 2011.
■ Catalytic Dynamic Resolution Applied to the Synthesis of 2,6-Disubstituted Piperidines: Preparation of (+)-Lupetidine and (–)-Epidihydropinidine
Timothy K. Beng and Robert E. Gawley*
Department of Chemistry and Biochemistry, University of Arkansas, Fayetteville, Arkansas 72701, U.S.A.
Abstract
The diastereoselective synthesis of trans-2,6-disubstituted piperidines has been rendered enantioselective by incorporating a catalytic dynamic resolution into the first alkylation step. The method has been applied to the synthesis of (–)-epidihydropinidine and (+)-trans-lupetidine.INTRODUCTION
A number of optically active piperidines found in natural products contain the 2,6-substitution pattern. Examples include lupetidine, pinidine, dihydropinidine, and solenopsin A (Figure 1A).1 We recently reported the highly enantioselective synthesis of 2-substituted piperidines by catalytic dynamic resolution (CDR) of N-Boc-2-lithiopiperidine 1 using diastereomeric ligands (S,S)-2 and (S,R)-2 (Figure 1B).2,3 Using Taylor’s conditions, we synthesized enantioenriched 2-allyl and benzyl piperidines by transmetalation to an organozinc species, followed by copper-mediated coupling.4 Campos’ conditions were also utilized to prepare 2-aryl piperidines via palladium-catalyzed coupling5 of the intermediate organozinc species derived from the resolved N-Boc-2-lithiopiperidine. We further used the Negishi coupling conditions to synthesize optically active 2-vinyl piperidines.3 We hereby extend our CDR methodology to the synthesis of enantioenriched 2-methyl piperidines, which serve as precursors for the enantioselective synthesis of 2,6-disubstituted piperidines.
RESULTS AND DISCUSSION
Our approach to 2,6-disubstituted piperidines consists of two steps: (i) catalytic dynamic resolution and methylation of N-Boc-2-lithiopiperidine 1, which furnishes the 2-substituted product; (ii) a second lithiation-substitution to obtain the diastereomeric 2,6-disubstituted products. It is well known that the alkyl group of N-Boc-2-alkylpiperidines resides in the axial orientation due to A1,3-strain. Stereoelectronic factors dictate the diastereoselectivity in favor of the trans-2,6-disubstituted piperidine in a second lithiation/substitution sequence.6,7 Deprotonation of N-Boc-piperidine (3, Figure 2) using s-BuLi leads to racemic 1, in which the amide π-bond and the p-orbital of the carbanionic center are orthogonal to each other. This arrangement is enforced by the stabilization arising from the chelation of the lithium to the carbonyl oxygen8 as well as the HOMO-HOMO repulsion between the amide π system and the carbanion electrons.9-11 Stereoretentive alkylation (SE2ret) of the equatorially lithiated species gives 4, which undergoes a ring flip to relieve the destabilizing A1,3-strain, giving 2-substituted piperidine conformer 5. Lithiation at C-6 furnishes intermediate 6. Subsequent SE2ret alkylation affords trans-2,6-disubstituted piperidine 7. In 2000, Beak showed that, when the second electrophilic quench introduces a carbonyl at C-6,1 another case of A1,3-strain could provide enough driving force for equilibration in favor of the cis-2,6-substituted piperidine, 8.
Catalytic dynamic resolution of 1 using 10 mmol of 3 and 10 mol% of (S,S)-2, followed by methylation was evaluated. After stirring for 3 h at –45 °C, cooling to –80 °C and quenching with precooled methyl iodide afforded S-9 in 62% yield and 93:7 er. Using Me2SO4, the yield and enantiomer ratio were improved to 71% and 96:4 (S:R) respectively. On one occasion using 1 mmol of 3, enantiopure S-9 was obtained but we were unable to reproduce the result on larger scales. There are two contrasting reports on the kinetics of deprotonation of 9. While Adamo and coworkers12 deprotonated S-9 (obtained in 44% yield from a classical resolution using (S)-mandelic acid) for 2 h at –20 °C, Knochel13 et al. recently reported complete lithiation of rac-9 after 4 h at –80 °C. We monitored the extent of lithiation of S-9 (96:4 er) using GC-MS by quenching aliquots with MeOD and checking for deuterium incorporation. In our hands, after 4 h of lithiation at –80 °C, only ~45% of S-9 was converted to 10. We had better results by raising the temperature to –45 °C for 3 h after adding the s-BuLi base at –80 °C. As indicated in entry 1, no racemization was observed. Beak previously reported that the deuterium is incorporated trans to the methyl group.6,7
With enantioenriched S-9 in hand, a solution was added to a cloudy mixture of s-BuLi/TMEDA/Et2O at –80 °C. After 30 min, the mixture was transferred to a second thermostatted bath at –45 °C and stirred for 3 h prior to cooling to –80 °C and addition of the desired electrophile. After workup, the diastereomeric products listed in Table 1 were obtained. Quenching with Me2SO4 provided trans-11 in 89% yield and 96:4 er (entry 2). Hydrolysis of the carbamate with CF3CO2H afforded (S,S)-12, corresponding to the relative configuration of (+)-lupetidine (Scheme 1).
With phenyl isocyanate, S-9 of 93:7 er gave the anilide 13 in 73% yield, 75:25 dr, and 93:7 er (entry 3). CSP-SFC analysis showed four peaks, one for each enantiomer of the diastereomers (Figure 3A). Methyl chloroformate yielded 14 in 85:15 dr with no loss of er (entry 4). Starting from S-9 of 96:4 er, quenching with Me3SiCl afforded trans-15 as the only diastereomer in 75% yield and 96:4 er as judged by CSP-GC (Figure 3B and Table 1, entry 5). When Bu3SnCl was employed, the trans isomer of the stannane 16 was obtained in 67% yield with no loss of er (entry 6).
Adamo and coworkers have reported a synthesis of (–)-epidihydropinidine whereby enantiopure S-9 is lithiated and alkylated with propyl iodide.12 In our hands, direct alkylation of lithiated 9 using 1-bromopropane proved to be problematic. In addition to the product trans-17, we detected significant amounts of the enamine S-18 along with S-9, with both compounds showing partial racemization from er 96:4 to 80:20 (S:R). Such byproducts are usually formed via a single electron transfer (SET).14-16 Under copper-mediated coupling conditions,2,17-19 quenching with allyl bromide afforded 19 in 57% yield, 76:24 dr (trans:cis), and 96:4 er (entry 8). Hydrogenation of trans-19 yielded trans-17, which was deprotected to give (–)-epidihydropinidine in just three steps starting from S-9 (Scheme 2). Starting from (R)-N-Boc-2-piperidine ethanol, Passarella et al. synthesized (2R,6S)-19 in five steps and used it to prepare both enantiomers of epidihydropinine.20 Highly regioselective Wacker oxidation21 of trans-19 afforded the pelletierine derivative 20 in 92% yield.
Electrophilic quench with β-bromostyrene under palladium-catalyzed coupling conditions3 afforded 21 in 61% yield, 89:11 dr (trans:cis), and 96:4 er for both diastereomers (entry 9). Substituted vinyl piperidines such as 21 serve as important intermediates for the enantio- and diastereoselective synthesis of fused bicyclic lactams.26 With bromobenzene, (2R,6S)-22 was obtained as the major diastereomer (entry 10). Knochel and coworkers recently demonstrated that when the diastereoselective arylation of rac-9 is carried out using a 1:1 mixture of Pd(dba)2:RuPhos in place of a 1:2 mixture of Pd(OAc)2:t-Bu3P·HBF4, the cis-2-methyl-5-phenyl piperidine is formed by a 1,2-palladium migration. With piperidines in a single chair conformation, these authors observed >95:5 dr for arylations at the 2-position.13
Lithiation-substitution with aldehydes was also investigated. After quenching with para-methoxybenzaldehyde and warming to room temperature, MeOH was added. Under these conditions, in situ cyclization of the initially formed β-amino alcohols gave an inseparable mixture of two diastereomeric oxazolidinones 23 in 82% yield, 67:33 dr (Figure 4a), and 96:4 er for both diastereomers (Table 1, entry 11). Base hydrolysis of the oxazolidinones provided the unprotected piperidinols, which were separable by column chromatography. It is worth noting that after quenching lithiated rac-9 with para-methoxybenzaldehyde and using different workup conditions (warming to –20 °C for 30 min), Beak reported that only the threo isomer cyclized to the corresponding oxazolidinone.7 Our spectroscopic data for 23b are in agreement with those of the threo isomer.
Quenching with 1-naphthaldehyde afforded two diastereomeric oxazolidinones 24a and 24b in 69% yield and 95:5 dr (entry 12). The observed 1H NMR spectrum showed that the oxazolidinone proton of the major isomer is more shielded than that of the minor isomer (Figure 4b). DFT calculations of oxazolidinones 24a and 24b indicate that, in the 1H NMR spectrum, the chemical shift for the oxazolidinone proton of 24a should be higher than that of 24b. From these data, and in analogy with 23b, we conclude that 24b is the major diastereomer.
Curiously, when S-9 of 93:7 er was lithiated and quenched with acetaldehyde, the alcohol 19 was obtained in 73% yield as a mixture of only two diastereomers in 84:16 dr with no loss in er (entry 13).
In summary, the enantioselective synthesis of optically active 2,6-disubstituted piperidines has been accomplished through catalytic dynamic resolution of N-Boc-2-lithiopiperidine and direct methylation of the resolved mixture, followed by a second-lithiation substitution under direct trapping, zinc-mediated, copper-mediated or palladium-catalyzed coupling conditions in the absence of a chiral ligand. In all cases high er’s and good dr’s are obtained. The method is very general since either configuration of 9 can be obtained using diastereomeric ligands (S,S)-2 and (S,R)-2. Near the completion of these studies, it occurred to us that excess TMEDA affects the kinetics of deprotonation of 9 as well as the dr’s. These results along with methods to obtain cis-2,6-disubstitution will be communicated in due course.
EXPERIMENTAL
All experiments involving organolithium reagents were carried out under an inert atmosphere of argon or nitrogen and using freshly distilled solvents. Et2O and THF were distilled from sodium benzophenone ketyl. The ligands TMEDA, conjugate acids of (S,S)-2 and (S,R)-2 were purified by Kugelrohr distillation from CaH2. Solutions of ZnCl2 (1 M in Et2O or THF) were obtained from commercial sources. Solid ZnCl2, CuCN, LiCl were flame-dried under vacuum prior to use. The concentration of commercial s-BuLi (solution in cyclohexane) was determined prior to use by No-D NMR spectroscopy.27 All electrophiles that were not newly purchased were distilled immediately before use. Newly purchased electrophiles with less than 99.5% purity were also distilled immediately before use. Column chromatography was performed on silica gel (230-400 mesh). Thin-layer chromatography (TLC) was performed on silica plates. Visualization of the TLC plates was aided by UV irradiation at 254 nm or by KMnO4 staining. For all enantiomer ratio (er) analyses, authentic racemic compounds were used to establish the method of separation of the enantiomers. The reaction temperatures were controlled by a thermostatted cooling coil and all reported temperatures were internal to a reaction vessel. The enantiomer ratios were determined by CSP-SFC. The following chiral columns were utilized; Regis Technologies Pirkle-Whelk-O-1 and Daicel Chiralcel OD-H. In some cases the enantiomer ratios were determined by CSP-GC on a β-cyclodextrin-permethylated 120 fused silica capillary column (30 m × 0.25 mm i.d., 20% permethylated β-cyclodextrin in SPB-35 poly(35% diphenyl/65% dimethyl)siloxane). Unless otherwise indicated, 1H, 13C, DEPT-135, COSY 45, HMQC, HMBC, NOESY NMR spectra were acquired using CDCl3 as solvent at ambient temperature. Chemical shifts are quoted in parts per million, referenced to residual CHCl3 at 7.27 ppm. DFT calculations were performed on the B3LYP level using the 6-311g (d,p) basis set.
Synthesis
N-Boc-piperidine, the alcohol precursors to ligands (S,S)-2 and (S,R)-2 were synthesized according to previously reported methods.2,3
(S)-N-Boc-2-methylpiperidine S-9
In an oven-dried, septum-capped 250 mL round bottom flask equipped with a stir bar, N-Boc-piperidine (1.85 g, 10 mmol) and freshly distilled TMEDA (6.0 mL, 40 mmol, 4.0 equiv) were dissolved in freshly distilled Et2O (100 mL) under argon. The solution was cooled to –80 °C and freshly titrated s-BuLi (9.3 mL, 1.3 M in cyclohexanes, 12 mmol, 1.2 equiv) was added slowly by means of a syringe over a ten minute period. The mixture was stirred for 3 h to effect deprotonation, affording rac-1·TMEDA. The diamino alcohol, precursor of (S,S)-2 (214 mg, 10 mol%) in Et2O (10 mL) was treated with s-BuLi (1.6 mL, 20 mol%). After deprotonation of N-Boc-piperidine, the preformed alkoxide (S,S)-2 was then added and the flask was quickly transferred to a second thermostatted bath at –45 °C, and allowed to stir for 3 h. The mixture was cooled to –80 °C and rapidly quenched with precooled dimethyl sulfate (1.5 mL, 15 mmol, 1.5 equiv). After 4 h, MeOH was added and the mixture was warmed to room temperature before 2 M HCl (20 mL) was added. The layers were separated and the aqueous layer was extracted with Et2O (3 x 20 mL). The combined organic layers were dried over MgSO4 and evaporated to obtain the crude product. Purification by flash chromatography on silica eluting with hexane-EtOAc (99:1) afforded 1.51 g of S-9 as a colorless oil in 71% yield, 96:4 er, data as reported.28 [α]D22 +39.5 (c 1, CHCl3), lit.29 for S-9 (100:0 er, +50.9, c 0.83, CHCl3). The er was determined by CSP-SFC as follows: Column: Pirkle Whelk-O-1, Flow Rate = 1.0 mL/min, Polarity Modifier = 1.0% EtOH. The R-enantiomer elutes after ~10.5 min and the S-enantiomer elutes after ~11.0 min.
General Procedure A: Lithiation of (S)-N-Boc-2-methylpiperidine followed by direct trapping with the electrophile.
To an oven-dried, septum-capped round bottom flask equipped with a stir bar, was added freshly distilled TMEDA (4.0 equiv) and distilled Et2O under argon. The solution was cooled to –80 °C and a solution of s-BuLi in cyclohexane (1.2 equiv) was added. A solution of S-9 (1.0 equiv) in Et2O was added to the flask containing the TMEDA/s-BuLi mixture. After 30 min at this temperature, the mixture was warmed to –45 °C and allowed to stir for 3 h. After cooling to –80 °C, the mixture was quenched with the electrophile (~1.1 to 1.5 equiv). After 2 – 4 h, MeOH was added and the mixture was stirred for 5 min (in some cases, MeOH was added after warming to room temperature). After warming to room temperature, 2 M HCl was added. The layers were separated and the aqueous layer was extracted with Et2O. The combined organic layers were dried over MgSO4 and evaporated to obtain the crude product. Purification by flash chromatography on silica was accompanied by er and dr determination.
General Procedure B: Lithiation of (S)-N-Boc-2-methylpiperidine followed by transmetalation and palladium-catalyzed arylation or vinylation.
To an oven-dried, septum-capped round bottom flask equipped with a stir bar, was added freshly distilled TMEDA (1.2 mL, 8.0 mmol, 4.0 equiv) in Et2O (10 mL) under argon. The solution was cooled to –80 °C and s-BuLi (1.2 equiv) was added. A precooled solution of S-9 of 96:4 er (398 mg, 2.0 mmol) in Et2O (5 mL) was added to the flask containing the TMEDA/s-BuLi mixture. After 30 min at this temperature, the mixture was transferred to the bath at –45 °C and allowed to stir for 3 h. After cooling to –80 °C, a solution of ZnCl2 (1.2 mL, 1.0 M in Et2O, 1.2 mmol, 0.6 equiv) was added. After 30 min, the mixture was warmed to room temperature slowly. After 30 min at room temperature, Pd(OAc)2 (10 mg, 0.04 mmol, 4 mol%), t-Bu3P·HBF4 (23 mg, 0.08 mmol, 8 mol%) and the aryl or vinyl bromide (1.1 equiv), were added sequentially. After stirring for 24 to 48 h at room temperature, NH4OH (10 mL, 10% aqueous solution) was added dropwise and the mixture was stirred for 30 min. The resulting slurry was filtered through Celite and rinsed with 10 mL Et2O. The filtrate was washed with 1 M HCl(aq) (20 mL), then with water (2 x 10 mL), dried over Na2SO4 and evaporated under reduced pressure to obtain the crude product.
Monitoring the extent of deprotonation of S-9: Quenching with MeOD
To an oven-dried, septum-capped 5 mL vial equipped with a stir bar, was added freshly distilled TMEDA (0.5 mL, 0.24 M solution in Et2O, 4.0 equiv), S-9 (0.5 mL, 0.06 M solution in Et2O, 1.0 equiv) under argon. It was cooled to –80 °C and a solution of s-BuLi in cyclohexane (1.2 equiv) was added slowly. After 30 min, 0.10 mL of CH3OD was added. The mixture was diluted with freshly distilled Et2O (ca 1 mL). The ethereal layer was filtered through Celite. The sample was placed in a GC vial and analyzed by GC-MS for deuterium incorporation using chemical ionization. When the deprotonation is complete, there is a noticeable shift of the protonated molecular ion peak from MH+ to MH++1. In most cases, the base peak at m/z 145 was utilized for analytical purposes. CSP-SFC conditions: Column: Pirkle Whelk-O-1, Flow Rate = 1.0 mL/min, Polarity Modifier = 1.0% EtOH. The (2S,6S)-10 elutes after ~10.5 min and the (2R,6R)-10 elutes after ~11.0 min. GC: Rt 5.98, MS: m/z 201 (MH+), 185, 173, 145 (base peak, MH+-CMe3), 129, 101, 57.
Electrophilic quench with dimethyl sulfate: Synthesis of trans-N-Boc-(+)-lupetidine trans-11
Using General Procedure A, S-9 of 96:4 er (398 mg, 2.0 mmol), TMEDA (1.2 mL, 8.0 mmol, 4.0 equiv), Et2O (10 mL), s-BuLi (2.4 mL, 2.4 mmol, 1.0 M, 1.2 equiv), Me2SO4 (0.29 mL, 3.0 mmol, 1.5 equiv) for 18 h prior to addition of 2 mL MeOH, gave the crude product as an oil. Purification by silica gel chromatography eluting with hexane-EtOAc (98:2) afforded 381 mg of trans-11 as a colorless liquid in 89% yield and 96:4 er, all other spectroscopic data as reported.12 [α]D22 +53.6 (c 1.25, MeOH), lit.12 for enantiopure trans-11, +59 (c 1.25, MeOH). The enantiomer ratio was evaluated by CSP-GC-MS, on a β-cyclodextrin-permethylated 120 fused silica capillary column [30 m × 0.25 mm i.d., 20% permethylated β-cyclodextrin in SPB-35 poly(35% diphenyl/65% dimethyl)siloxane, initial temperature = 120 °C, final temperature = 200 °C, hold time = 5 min, rate = 1 °C/min. The minor enantiomer elutes after 14.6 min and the major enantiomer elutes after 14.9 min. MS: m/z 214 (MH+), 198, 186, 158 (base peak, MH+-CMe3), 142, 114, 98, 57. On one occasion, enantiopure trans-11 was obtained from enantiopure S-9 (see CSP-GC-MS trace below) but typical er’s ranged from 93:7 to 96:4 (S:R).
N-Boc-deprotection
To trans-11 (214 mg, 1.0 mmol) dissolved in CH2Cl2 (5.0 mL), was added CF3CO2H (1.5 mL) under argon at 0 °C. The mixture was stirred for 5 h at this temperature and concentrated in vacuo to obtain the salt. The salt was basified to pH 10 – 12 with 20% NaOH(aq). The layers were separated and the aqueous layer was extracted with CH2Cl2 (2 x 5 mL). The combined organic layers were washed with brine, dried over MgSO4 and concentrated under reduced pressure to give 109 mg of trans-(+)-lupetidine 12 in 96% yield, [α]D22 +10.3 (c 0.50, EtOH), lit.12 [α]D +12.5 (c 0.5, EtOH).
Electrophilic quench with phenyl isocyanate: Synthesis of 13
Using General Procedure A, S-9 of 93:7 er (398 mg, 2.0 mmol), TMEDA (1.2 mL, 8.0 mmol, 4.0 equiv), Et2O (10 mL), s-BuLi (2.4 mL, 2.4 mmol, 1.0 M, 1.2 equiv), precooled phenyl isocyanate (0.33 mL in 1.0 mL Et2O, 3.0 mmol, 1.5 equiv) for 2 h prior to addition of 2 mL MeOH, gave the crude product as a yellowish solid. Purification by silica gel chromatography eluting with hexane-EtOAc (90:10) afforded 464 mg of 13 as a white solid in 73% yield, 75:25 dr and 93:7 & 92:8 er for the trans and cis diastereomers respectively. GC (Rt 11.8 min), MS: m/z 319 (MH+), 291, 245, 219, 170, 142 (base peak), 122, 94, 57. Data for the trans-isomer; 1H NMR (300 MHz, CDCl3) δ = 8.4 (1H, s, CONH), 7.33–6.85 (5H, m, Ph), 4.19 (1H, br, NCH), 3.91 (1H, br, NCH), 1.95–1.41 (15H, m), 1.29 (3H, d, CH3) 13C NMR (75.5 MHz, CDCl3) δ = 172.3 (C=O), δ = 156.9 (C=O), 138.3 (C), 129.0 (CH), 123.8 (CH), 119.5 (CH), 81.0 (C), 55.1 (CH), 48.4 (CH), 28.4 (CH3), 27.1 (CH2), 23.5 (CH2), 19.1 (CH3) and 14.2 (CH2). The enantiomer ratio of the crude mixture was evaluated by CSP-SFC, monitoring at 210 or 254 nm, by comparison with an authentic racemic sample, under the following column conditions: Column: Daicel Chiralcel OD-H, Flow Rate = 2.0 mL/min, Polarity Modifier = 5.0% EtOH. For trans-13, the minor enantiomer elutes after 5.6 min and the major elutes after 7.6 min. For cis-13, the minor enantiomer elutes after 4.5 min and the major elutes after 6.6 min.
Electrophilic quench with methyl chloroformate: Synthesis of 14
Using General Procedure A, S-9 of 93:7 er (398 mg, 2.0 mmol), TMEDA (1.2 mL, 8.0 mmol, 4.0 equiv), Et2O (10 mL), s-BuLi (2.4 mL, 2.4 mmol, 1.0 M, 1.2 equiv), precooled methyl chloroformate (0.23 mL in 0.5 mL Et2O, 3.0 mmol, 1.5 equiv) for 2 h prior to addition of 2 mL MeOH, gave the crude product as an oil. Purification by silica gel chromatography eluting with hexane-EtOAc (95:5) afforded 416 mg of 14 in 81% yield, 85:15 dr (trans:cis) and 93:7 er for both diastereomers. GC (Rt 8.16 for major diastereomer, 8.21 for minor diastereomer), MS: m/z 258 (MH+), 242, 230, 184, 158 (base peak, MH+-Boc), 142, 98, 57.
Data for the trans-isomer; 1H NMR (300 MHz, CDCl3) δ = 4.25–4.05 (2H, m, NCH), 3.65 (3H, s, OCH3), 1.91–0.92 (15H, m), 0.75 (3H, d, CH3); 13C NMR (75.5 MHz, CDCl3) δ = 174.5 (C=O), δ = 156.3 (C=O), 80.2 (C), 54.1 (CH), 52.2 (CH3), 47.2 (CH), 28.5 (CH3), 28.0 (CH2), 26.8 (CH2), 17.5 (CH2) and 13.4 (CH3). The enantiomer ratio of the major diastereomer was evaluated by CSP-GC-MS, on a β-cyclodextrin-permethylated 120 fused silica capillary column [30 m × 0.25 mm i.d., 20% permethylated β-cyclodextrin in SPB-35 poly(35% diphenyl/65% dimethyl)siloxane, initial temperature = 100 °C, final temperature = 200 °C, hold time = 10 min, rate = 5 °C/min. The minor enantiomer elutes after 26.8 min and the major enantiomer elutes after 26.9 min.
Electrophilic quench with TMSCl: Synthesis of 15
Using General Procedure A, S-9 of 96:4 er (199 mg, 1.0 mmol), TMEDA (0.6 mL, 4.0 mmol, 4.0 equiv), Et2O (10 mL), s-BuLi (1.2 mL, 1.2 mmol, 1.0 M, 1.2 equiv), Me3SiCl (144 mg, 1.2 mmol, 1.2 equiv) for 4 h prior to addition of 2 mL MeOH, gave the crude product as an oil. Purification by silica gel chromatography eluting with hexane-EtOAc (98:2) afforded 203 mg of trans-15 in 75% yield, as a single diastereomer in 96:4 er. GC (Rt 6.78), MS: m/z 272 (MH+), 228, 214, 200 (base peak, MH+-SiMe3), 172, 156, 57. 1H NMR (300 MHz, CDCl3) δ = 4.4–4.2 (1H, m, NCH), 2.5 (1H, m, NCH), 1.8–1.5 (6H, m), 1.4 (9H, s, CH3), 1.2 (3H, d, CH3) 0.05 (9H, s, CH3); 13C NMR (75.5 MHz, CDCl3) δ = 155.3, 78.6 (C), 48.2 (CH), 42.2 (CH), 30.0 (CH2), 28.5 (CH3), 26.5 (CH2), 20.5 (CH2), 17.0 (CH3), and -0.3 (CH3). The er was evaluated by CSP-GC-MS on a β-cyclodextrin-permethylated 120 fused silica capillary column [30 m × 0.25 mm i.d., 20% permethylated β-cyclodextrin in SPB-35 poly(35% diphenyl/65% dimethyl)siloxane], initial temperature = 90 °C, final temperature = 120 °C, hold time = 5 min, rate = 1 °C/min.
Electrophilic quench with tributylstannyl chloride: Synthesis of 16
Using General Procedure A, S-9 of 96:4 er (199 mg, 1.0 mmol), TMEDA (0.6 mL, 4.0 mmol, 4.0 equiv), Et2O (10 mL), s-BuLi (1.2 mL, 1.2 mmol, 1.0 M, 1.2 equiv), precooled Bu3SnCl (0.32 mL in 0.5 mL Et2O, 1.2 mmol, 1.2 equiv) for 4 h prior to addition of 2 mL MeOH, gave the crude product as an oil. Purification by silica gel chromatography eluting with hexane-EtOAc (99:1) afforded 323 mg of trans-16 in 67% yield, as a single diastereomer in 96:4 er. 1H NMR (300 MHz, CDCl3) δ = 4.44–4.32 (1H, m, NCH), 2.9 to 2.8 (1H, m, NCH), 1.8–1.6 (6H, m), 1.6 to 1.1 (21H, m), 1.1 to 0.85 (18H, m,); 13C NMR δ = 156.5, 78.8 (C), 47.7 (CH), 38.3 (CH), 30.7 (CH2), 30.5 (CH2), 29.3 (CH2), 28.3 (CH3), 27.6 (CH2), 21.4 (CH2), 16.0 (CH3), 13.7 (CH3), and 12.2 (CH2). The enantiomers were resolved by CSP-SFC under the following conditions: Column: Pirkle Whelk-O-1, Flow Rate = 1.0 mL/min, Polarity Modifier = 1.0% EtOH, Outlet Pressure = 150 psi, Oven Temperature = 35 °C.
Electrophilic quench with allyl bromide: Synthesis of 19
To an oven-dried, septum-capped round bottom flask equipped with a stir bar, was added freshly distilled TMEDA (1.2 mL, 8.0 mmol, 4.0 equiv) in Et2O (10 mL) under argon. The solution was cooled to –80 °C and s-BuLi (2.4 mL, 2.4 mmol, 1.0 M in cyclohexanes, 1.2 equiv) was added. A solution of S-9 of 96:4 er (398 mg, 2.0 mmol) in Et2O (5 mL) was added to the flask containing the TMEDA/s-BuLi mixture. After 30 min at this temperature, the mixture was transferred to the bath at –45 °C and allowed to stir for 3 h. After cooling to –80 °C, a solution of ZnCl2 (180 mg, 1.3 mmol, 1.3 equiv) in THF (2 mL), was added. After 30 min, a solution of CuCN·2LiCl [prepared from CuCN (214 mg, 2.4 mmol, 1.2 equiv) and LiCl (214 mg, 5.0 mmol, 2.5 equiv)] in THF (3 mL) was added. After 30 min, allyl bromide (150 mg, 1.5 mmol, 1.5 equiv) was added. The mixture was allowed to stir for 10 h at this temperature prior to addition of MeOH (2 mL) and warming to room temperature. A solution of NH4Cl (5 mL) was added and the aqueous layer was extracted with Et2O. The combined organic layers were dried over Na2SO4 and evaporated to give the crude product. Purification by silica gel chromatography eluting with hexane-EtOAc (98:2) afforded 272 mg of 19 as a colorless oil in 57% yield, 76:24 dr (trans:cis) and 96:4 er for the major diastereomer (the minor diastereomer could not be resolved on our CSP-GC nor CSP-SFC columns). Data for the trans isomer; [α]D22 +20.3 (c = 1.5, CHCl3), lit.20 [α]D25 +23.7 (c = 1.5, CHCl3). All other data as reported.20 The er was evaluated by CSP-GC-MS on a β-cyclodextrin-permethylated 120 fused silica capillary column [30 m × 0.25 mm i.d., 20% permethylated β-cyclodextrin in SPB-35 poly(35% diphenyl/65% dimethyl)siloxane, initial temperature = 100 °C, final temperature = 200 °C, hold time = 10 min, rate = 5 °C/min.
Synthesis of (–)-N-Boc-epidihydropinidine, trans-17
Pd(OH)2 (24 mg, 0.17 mmol, 40 mol%) was added to a solution of trans-19 (100 mg, 0.42 mmol, 1.0 equiv) in freshly distilled MeOH (5 mL) under hydrogen (1 atm) at room temperature. The reaction mixture was stirred for 2 days at this temperature, filtered through a plug of Celite and concentrated under reduced pressure to give 92 mg of 17 in 91% yield. [α]D22 +37.7 (c 0.25, CHCl3), lit.20 [α]D25 +40.4 (c 0.25, CHCl3). All other data as reported.20
N-Boc-deprotection of trans-17: Synthesis of (–)-epidihydropinidine
To trans-17 (85 mg, 0.35 mmol) dissolved in CH2Cl2 (1.0 mL), was added CF3CO2H (0.5 mL) under argon at 0 °C. The mixture was stirred for 5 h at this temperature and concentrated in vacuo to obtain the salt. The salt was basified to pH 10 – 12 with 20% NaOH(aq). The layers were separated and the aqueous layer was extracted with CH2Cl2 (2 x 2 mL). The combined organic layers were washed with brine, dried over MgSO4 and concentrated under reduced pressure to give 50 mg of (–)-epidihydropinidine in 100% yield, [α]D22 –2.2 (c 0.25, CHCl3), lit.20 for enantiopure (–)-epidihydropinidine [α]D25 –2.7 (c 0.2, CHCl3).
Synthesis of trans-20
CuCl (42 mg, 0.42 mmol, 1 equiv) and PdCl2 (8 mg, 0.042 mmol, 10 mol%) were dissolved in 1.0 mL of DMF/H2O (10:1) and the resulting suspension was stirred for 1 h at room temperature under an O2 atmosphere. A solution of trans-19 (100 mg, 0.42 mmol, 1.0 equiv) in 1.0 mL of DMF:H2O (10:1) was added to the reaction mixture and stirred for 18 h. After complete conversion of trans-19 as indicated by TLC analysis, the reaction mixture was quenched with 20% KHSO4(aq) (1 mL) and extracted with Et2O (3 x 2 mL). The combined organic layers were washed with saturated NaHCO3(aq) (1 mL), then with brine (1 mL) and dried over Na2SO4. Concentration of the organic layer and purification by column chromatography on silica, eluting with hexane-EtOAc (80:20) afforded 98 mg of 20 in 92% yield. 1H NMR: δ 4.6 (br, m, 1H), 4.3 (br, m, 1H), 2.8-2.5 (m, 2H), 2.2 (s, 3H), 1.8-1.3 (m, 15H), 1.1 (d, 3H); 13C NMR (75.5 MHz): δ 206.5, 154.6, 79.1, 48.3, 45.4, 29.9, 29.7, 28.4 (3C), 27.7, 20.0, 13.5.
Electrophilic quench with β-bromostyrene: Synthesis of 21
Using General Procedure B, quenching with β-bromostyrene (0.15 mL, 1.1 mmol, 1.1 equiv) for 48 h gave the crude product as an oil. Purification by silica gel column chromatography eluting with hexane-EtOAc (98:2) afforded 368 mg of 21 in 61% yield, 89:11 dr (trans:cis), 96:4 er for both diastereomers. 1H NMR: δ 7.38-7.15 (m, 5H), 6.39 (dd, 1H), 6.18 (dd, 1H), 4.95 (br, s, 1H), 4.41 (m, 1H), 1.82-1.40 (m, 15H), 1.1 (d, 3H); 13C NMR (75.5 MHz): δ 154.8, 137.2, 131.7, 129.9, 128.4 (2C), 122.3, 126.2 (2C), 78.8, 51.1, 45.9, 38.6, 30.0, 28.4 (3C), 25.6, 18.6, 15.6. The er was determined by CSP-SFC as follows: Column: Pirkle-Whelk-O-1, Flow Rate: 2.0 mL/min, Polarity Modifier %: 2.0% EtOH, Outlet Pressure = 150 psi, Oven Temperature = 35 °C.
Electrophilic quench with bromobenzene: Synthesis of 22
Using General Procedure B, quenching with phenyl bromide (0.25 mL, 1.1 mmol, 1.1 equiv) gave the crude product as an oil. Purification by silica gel column chromatography eluting with hexane-EtOAc (98:2) afforded 401 mg of 22 in 73% yield, 87:13 dr (trans:cis), 96:4 er for both diastereomers. The er was determined by CSP-SFC as follows: Column: Pirkle-Whelk-O-1, Flow Rate: 1.0 mL/min, Polarity Modifier %: 3.0% EtOH, Outlet Pressure = 150 psi, Oven Temperature = 35 °C.
Electrophilic quench with 4-methoxybenzaldehyde: Synthesis of 23
Using General Procedure A, S-9 of 96:4 er (398 mg, 2.0 mmol), TMEDA (1.2 mL, 8.0 mmol, 4.0 equiv), Et2O (10 mL), s-BuLi (2.4 mL, 2.4 mmol, 1.0 M, 1.2 equiv), 4-methoxybenzaldehyde (0.37 mL, 3.0 mmol, 1.5 equiv) for 2 h prior to warming to room temperature and addition of 2 mL MeOH, gave the crude product as a pale yellow oil. Purification by silica gel chromatography eluting with hexane-EtOAc (60:40) afforded 430 mg of 17 in 82% yield, 67:33 dr and 96:4 er for both diastereomers. GC (Rt 11.86 min for either diastereomer), MS: m/z 262 (base peak, MH+), 218, 154. 1H NMR (300 MHz, CDCl3) 7.33–7.21 (2H, d, major), 7.20–7.11 (2H, d, minor), 6.92–6.76 (4H, m, major + minor), 5.57–5.52 (1H, d, minor), 4.96–4.90 (1H, d, major), 4.22 to 4.05 (2H, br, NCH major and minor), 3.91 (1H, br, NCH for minor), 3.8 to 3.72 (6H, two singlets, OCH3 for major and minor), (3.51 (1H, br, NCH for major), 1.95–1.22 (12H, m), 1.22 to 1.11 (6H, two doublets, CH3 for major and minor). 13C NMR (75.5 MHz, CDCl3) δ = 159.5, 158.9, 156.9, 156.3, 132.1 and 129.7, 128.5 and 127.4, 114.2 and 113.8, 82.3 and 77.8, 58.2, 55.4, 55.31, 55.24, 54.77, 45.6, 45.1, 29.9, 29.0, 28.9, 26.9, 17.9, 17.7, 16.4, 15.7.
Base Hydrolysis of 23
To 23 (261 mg, 1.0 mmol) was added solid NaOH (160 mg, 4 mmol, 4 equiv) and absolute EtOH (5 mL). The resulting suspension was heated at reflux. After 2 h, the mixture was concentrated in vacuo and the orange residue was dissolved in H2O (5.0 mL). Et2O (10 mL) was added and the layers were separated. The aqueous layer was extracted with Et2O (3 x 10 mL). The combined organic layers were dried over K2CO3 and concentrated under reduced pressure to give the crude product. Purification by silica gel chromatography eluting with MeOH-CH2Cl2-NH3(aq) (10:5:1) afforded 219 mg of the unprotected piperidinol in 93% yield. 1H NMR (300 MHz, CDCl3) 7.4–7.21 (2H, d), 7.0–6.8 (2H, d), 4.68 (1H, d), 3.8 (3H, s, OCH3), 3.2 to 2.8 (2H, br, NCH), 1.95–1.22 (6H, m), 1.22 to 1.11 (3H, d, CH3). 13C NMR (75.5 MHz, CDCl3) δ = 158.9 (C), 134.5 (C), 127.6 (CH), 113.6 (CH), 73.9 (CH), 55.8 (CH), 55.2 (CH3), 46.8 (CH), 31.1 (CH2), 25.6 (CH2), 19.4 (CH3), and 18.8 (CH2). The product is easily oxidized; as such it should be stored under nitrogen in the freezer.
Electrophilic quench with 1-naphthaldehyde: Synthesis of 24
Using General Procedure A, S-9 of 93:7 er (398 mg, 2.0 mmol), TMEDA (1.2 mL, 8.0 mmol, 4.0 equiv), Et2O (10 mL), s-BuLi (2.4 mL, 2.4 mmol, 1.0 M, 1.2 equiv), 1-naphthaldehyde (0.40 mL, 3.0 mmol, 1.5 equiv) for 2 h prior to warming to room temperature and addition of 2 mL MeOH, gave the crude product as a pale yellow oil. Purification by silica gel chromatography eluting with hexane-EtOAc (60:40) afforded 388 mg of 24 in 69% yield, >99:1 dr (syn:anti), 93:7 er for the major diastereomer. GC (Rt 11.13 min), MS: m/z 282 (base peak, MH+), 266, 238, 154, 57. 1H NMR: δ 7.8-7.15 (m, 7H), 5.8 to 5.74 (d, 1H), 4.3 (m, 1H), 3.7 (m, 1H), 1.98-1.12 (m, 6H), 1.1 (d, 3H); 13C NMR (75.5 MHz, CDCl3) δ = 156.3 (C=O), 133.8 (C), 133.5 (C), 131.1 (C), 129.2 (CH), 126.7 (CH), 125.9 (CH), 125.4 (CH), 123.5 (CH), 122.5 (CH) 121.9 (CH), 79.4 (C), 57.8 (CH), 45.3 (CH), 30.7 (CH2), 29.0 (CH2), 17.8 (CH2), 16.0 (CH3).
Electrophilic quench with acetaldehyde: Synthesis of 25
Using General Procedure A, S-9 of 93:7 er (398 mg, 2.0 mmol), TMEDA (1.2 mL, 8.0 mmol, 4.0 equiv), Et2O (10 mL), s-BuLi (2.4 mL, 2.4 mmol, 1.0 M, 1.2 equiv), acetaldehyde (0.16 mL, 3.0 mmol, 1.5 equiv) for 2 h prior to addition of 2 mL MeOH, gave the crude product as a pale yellow oil. Purification by silica gel chromatography eluting with hexane-EtOAc (60:40) afforded 354 mg of 25 in 73% yield, 84:16 dr, and 93:7 er for both diastereomers.
Data for the major diastereomer; GC (Rt 9.45 min), MS: m/z 244 (MH+), 198, 188, 170, 144 (base peak, MH+-Boc), 126, 57. 13C NMR (75.5 MHz, CDCl3) δ = 155.8 (C=O), 79.3 (C), 68.0 (CH), 57.8 (CH), 49.1 (CH), 28.5 (CH2), 28.1 (CH3), 22.7 (CH2), 20.2 (CH3), 18.1 (CH3) and 17.2 (CH2).
Data for the minor diastereomer; GC (Rt 9.51 min), MS: m/z 244 (MH+), 198, 188, 170, 144 (base peak, MH+-Boc), 126, 57. 13C NMR (75.5 MHz, CDCl3) δ = 157.8 (C=O), 80.2 (C), 70.7 (CH), 58.3 (CH), 48.4 (CH), 28.5 (CH3), 27.0 (CH2), 23.4 (CH2), 21.7 (CH3), 19.8 (CH3) and 14.8 (CH2). The enantiomers were resolved by CSP-SFC under the following conditions: Column: Daicel Chiralcel OD-H, Flow Rate: 2.0 mL/min, Polarity Modifier%: 1.0% EtOH, Outlet Pressure = 150 psi, Oven Temperature = 35 °C. For the major diastereomer, the major enantiomer elutes after 4.2 min and minor enantiomer elutes after 4.7 min. For the minor diastereomer, the major enantiomer elutes after 5.3 min and minor enantiomer elutes after 5.8 min.
ACKNOWLEDGEMENTS
This work was supported by the National Science Foundation (CHE 1011788) and the Arkansas Biosciences Institute. Core facilities were funded by the National Institutes of Health (P30 R031154) and the Arkansas Biosciences Institute. The authors are grateful to Professor Peter Pulay for help with computational studies.
References
1. T. Wilkinson, N. Stehle, and P. Beak, Org. Lett., 2000, 2, 155. CrossRef
2. T. K. Beng and R. E. Gawley, J. Am. Chem. Soc., 2010, 132, 12216. CrossRef
3. T. K. Beng and R. E. Gawley, Org. Lett., 2011, 13, 394. CrossRef
4. J. P. N. Papillon and R. J. K. Taylor, Org. Lett., 2002, 4, 119. CrossRef
5. K. R. Campos, A. Klapars, J. H. Waldman, P. G. Dormer, and C. Chen, J. Am. Chem. Soc., 2006, 128, 3538. CrossRef
6. P. Beak and W. K. Lee, J. Org. Chem., 1990, 55, 2578. CrossRef
7. P. Beak and W. K. Lee, J. Org. Chem., 1993, 58, 1109. CrossRef
8. D. Stead, G. Carbone, P. O'Brien, K. R. Campos, I. Coldham, and A. Sanderson, J. Am. Chem. Soc., 2010, 132, 7260. CrossRef
9. L. J. Bartolotti and R. E. Gawley, J. Org. Chem., 1989, 54, 2980. CrossRef
10. R. D. Bach, M. L. Braden, and G. J. Wolber, J. Org. Chem., 1983, 48, 1509. CrossRef
11. N. G. Rondan, K. N. Houk, P. Beak, W. J. Zajdel, J. Chandrasekhar, and P. v. R. Schleyer, J. Org. Chem., 1981, 46, 4108. CrossRef
12. M. F. A. Adamo, V. K. Aggarwal, and M. A. Sage, Synth. Commun., 1999, 29, 1747. CrossRef
13. S. Seel, T. Thaler, K. Takatsu, C. Zhang, H. Zipse, B. F. Straub, P. Mayer, and P. Knochel, J. Am. Chem. Soc., 2011, 133, 4774. CrossRef
14. K. S. Rein, Z. H. Chen, P. T. Perumal, L. Echegoyen, and R. E. Gawley, Tetrahedron Lett., 1991, 32, 1941. CrossRef
15. R. E. Gawley, Q. Zhang, and A. T. McPhail, Tetrahedron: Asymmetry, 2000, 11, 2093. CrossRef
16. R. E. Gawley, D. B. Eddings, M. Santiago, and D. A. Vicic, Org. Biomol. Chem., 2006, 4, 4285. CrossRef
17. I. Coldham, S. Raimbault, P. T. Chovatia, J. J. Patel, D. Leonori, N. S. Sheikh, and D. T. E. Whittaker, Chem. Commun., 2008, 4174. CrossRef
18. I. Coldham and D. Leonori, J. Org. Chem., 2010, 75, 4069. CrossRef
19. I. Coldham, S. Raimbault, D. T. E. Whittaker, P. T. Chovatia, D. Leonori, J. J. Patel, and N. S. Sheikh, Chem. Eur. J., 2010, 16, 4082. CrossRef
20. D. Passarella, S. Riva, G. Grieco, F. Cavallo, B. Checa, F. Arioli, E. Riva, D. Comi, and B. Danieli, Tetrahedron: Asymmetry, 2009, 20, 192. CrossRef
21. G. Cheng, X. Wang, D. Su, H. Liu, F. Liu, and Y. Hu, J. Org. Chem., 2010, 75, 1911. CrossRef
22. A. P. Dobbs and S. J. J. Guesne, Synlett, 2005, 2101. CrossRef
23. H. Takahata, K. Inose, N. Araya, and T. Momose, Heterocycles, 1994, 38, 1961. CrossRef
24. H. Takahata, M. Kubota, S. Takahashi, and T. Momose, Tetrahedron: Asymmetry, 1996, 7, 3047. CrossRef
25. S. Yamauchi, S. Mori, Y. Hirai, and Y. Kinoshita, Biosci., Biotechnol., Biochem., 2004, 68, 676. CrossRef
26. S. H. Lim, S. Ma, and P. Beak, J. Org. Chem., 2001, 66, 9056. CrossRef
27. T. R. Hoye, B. M. Eklov, T. D. Ryba, M. Voloshin, and L. J. Yao, Org. Lett., 2004, 6, 953. CrossRef
28. P. Beak and W. K. Lee, Tetrahedron Lett., 1989, 30, 1197. CrossRef
29. D. Doller, R. Davies, and S. Chackalamannil, Tetrahedron: Asymmetry, 1997, 8, 1275.
CrossRef