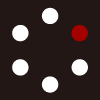
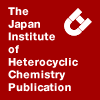
HETEROCYCLES
An International Journal for Reviews and Communications in Heterocyclic ChemistryWeb Edition ISSN: 1881-0942
Published online by The Japan Institute of Heterocyclic Chemistry
e-Journal
Full Text HTML
Received, 6th June, 2011, Accepted, 30th June, 2011, Published online, 7th July, 2011.
■ Synthesis of Fused Tricyclic Heterocycles by Condensation, Cyclization, Dipolar Cycloaddition Cascade of α-Benzenesulfonyl and α-Phenylthio Substituted Aldehydes
Iain Coldham,* Adam J. M. Burrell, Luke Watson, Niall Oram, and Nathaniel G. Martin
Department of Chemistry, University of Sheffield, Sheffield, S3 7HF, U.K.
Abstract
Heating α-benzenesulfonyl- or α-phenylthio-aldehydes that contain a tethered alkyl chloride and alkene originating from the α- position of the aldehyde with hydroxylamine sets up a cascade (tandem) reaction sequence involving condensation to an intermediate oxime, then cyclization to an intermediate nitrone, then intramolecular dipolar cycloaddition. The fused tricyclic products are formed with complete stereochemical control and were converted in two steps either to the natural product (±)-myrioxazine A or to a 1,3-oxazine that is present in some yuzurimine type Daphniphyllum alkaloids.INTRODUCTION
Many natural products, particularly alkaloids, consist of polycyclic ring systems containing one nitrogen atom. A wide variety of approaches have been reported to such compounds and, in the interests of efficiency, cascade processes involving more than one ring formation in a single pot are desirable.1 Methodology that uses the combination of a cyclization then an in situ intramolecular cycloaddition can provide a way to access three rings in one reaction sequence.2–8 This chemistry provides, very rapidly, a high degree of molecular complexity often with high levels of stereocontrol and has found application for the preparation of several natural products. Of note are the impressive examples of the group of Padwa describing the synthesis of some indole alkaloids using a cascade involving cyclization of a metallocarbene (derived from a diazocarbonyl compound) with a tethered carbonyl of a carboxylic amide or lactam, followed by intramolecular dipolar cycloaddition of the resulting carbonyl ylide.8
Our work in this area stemmed from an interest in intramolecular dipolar cycloaddition reactions of azomethine ylides (for the synthesis of alkaloids containing a pyrrolidine ring).9–16 We were interested in reports by Pearson and co-workers,17,18 who showed that an imine could undergo cyclization onto a tethered alkyl halide to give an iminium ion which, after destannylation, provided an azomethine ylide that was amenable to dipolar cycloaddition with an activated dipolarophile. As a result, we prepared the aldehyde 1 that contains a tethered alkyl chloride (for initial cyclization of the intermediate imine) and a tethered (unactivated) dipolarophile. We were pleased to find that treatment of the aldehyde 1 with glycine ethyl ester or hydroxylamine resulted in the formation of the tricyclic products 2 or 3 respectively (Scheme 1).19,20 In each case a single stereoisomer was formed and the stereochemistry was verified by single crystal X-ray analysis of derivatives of 2 and 3. Formation of the intermediate azomethine ylide by decarboxylation using glycine followed by cycloaddition was possible using the activated substrate 4 and gave a mixture of diastereoisomers 5 and 6 (Scheme 1). Removal of the phenylsulfonyl group with sodium amalgam led to different outcomes, with the sulfone 5 providing the product 7, and the sulfone 6 giving the elimination product 8.19
The successful demonstration of the cascade cyclization–cycloaddition chemistry with simple chloroaldehydes led to its subsequent application to a synthesis of some aspidosperma alkaloids and to the core of the stenine alkaloids.19–21 In all these cases an ethyl substituent was located alpha to the aldehyde and this avoids any complications due to potential enolization or enamine formation. Indeed, the cascade chemistry was more difficult using an aldehyde with an α C–H.22 Therefore, as a possible method to prepare tricyclic products that contain a hydrogen atom at all three ring junction carbon atoms, we decided to explore the use of aldehydes containing a phenylthio or benzenesulfonyl substituent at the alpha position that could potentially be removed after the cycloaddition reaction. Here we report the investigation of this chemistry and its application in synthesis.
RESULTS AND DISCUSSION
Commercially available (phenylsulfonyl)acetonitrile 9 was treated with lithium diisopropylamide (LDA) and 5-bromopentene to give the nitrile 10 [which could be separated from a small amount (11%) of the dialkylated product by column chromatography] (Scheme 2). The mixture was heated to promote complete reaction in this step and in the subsequent alkylation to give the nitrile 11. In this second step, in addition to the chloride 11, some of the corresponding bromide product was obtained, due to the presence of bromide ions in solution; so the mixture was heated with lithium chloride to give solely the chloride 11. We had anticipated that reduction of the nitrile with diisobutylaluminium hydride (DIBAL-H) would provide, after aqueous work-up, the desired aldehyde. However, we were disappointed to find that treatment with DIBAL-H in CH2Cl2, THF or toluene led to recovery of nitrile 11 together with over-reduction to amine 12. None of the desired aldehyde was obtained.
We therefore turned to the alkylation of (phenylthio)acetonitrile 13 with 5-bromopentene, which gave the desired nitrile 14 (together with some dialkylated product) (Scheme 3). This step, and the second alkylation, with 1-bromo-3-chloropropane, took place at room temperature and the chloride 15 was isolated without contamination with the corresponding bromide. Reduction of nitrile 15 with DIBAL-H gave aldehyde 16 in excellent yield. Oxidation of the sulfide 16 using mCPBA was slow and attempted isolation of the sulfoxide intermediate resulted in decomposition. A better method was the use of Oxone® in acetone–water (0 °C to room temp., 5 h), which produced the aldehyde 17 in good yield.
With the aldehydes 16 and 17 in hand, we attempted the cascade condensation, cyclization, cycloaddition chemistry. We were disappointed to find that heating either of these aldehydes with glycine or glycine ethyl ester in toluene (our standard conditions)20 resulted only in decomposition or by-products (crude 1H NMR spectroscopy showed a mixture of products and suggested that the alkene was still present). Therefore these substrates appear to be unsuitable for our cascade chemistry with cycloaddition using an intermediate azomethine ylide.
However, we were pleased to find that treatment of either aldehyde 16 or 17 with hydroxylamine resulted in successful reaction (Scheme 4). Remarkably, very high yields of a single stereoisomer were obtained using either aldehyde. The stereochemistry of cycloadduct 20 was confirmed by single crystal X-ray analysis (Figure 1). The stereochemistry of cycloadduct 18 is depicted as being in accord with that of 20 and that of the related product 3 (Scheme 1). In addition, the large coupling constant (J = 11.5 Hz) in the 1H NMR spectrum across the ring junction protons is in agreement with the trans arrangement of these atoms.20
Treatment of cycloadduct 18 with Raney nickel led predominantly to decomposition, but reduction using zinc and acetic acid gave the product 19 in quantitative yield. The cycloadduct 20 was treated with sodium amalgam and this resulted (predominantly) in reduction of both the N–O and C–S bonds, with retention of configuration, to give the amino-alcohol 21 in high yield. This compound was heated with paraformaldehyde, as previously reported,22,23 to give the natural product (±)–myrioxazine A. This route to the amino-alcohol 21 also completes a formal synthesis of myrionidine and schoberine, alkaloids with antimalarial activity.24
The cascade chemistry using the substrates 16 and 17 involves a cyclization step to set up a piperidine ring, followed by an intramolecular cycloaddition that provides another six-membered ring together with a five-membered ring. To test the chemistry with a related substrate, we chose the aldehyde 24 that would lead, in the cyclization step, to a five-membered rather than six-membered ring. This was prepared by alkylation of the nitrile 14 to give the alcohol 22 followed by chlorination to give 23 and DIBAL-H reduction (Scheme 5). In the same way as the formation of the sulfone 17, oxidation of the sulfide 24 gave the sulfone 25. The low yield in this step was due to side reactions (possible elimination of the sulfone or intermediate sulfoxide).
Heating the aldehyde 24 with hydroxylamine at 60 °C gave the oxime 26 (Scheme 6). Using higher temperatures (heating under reflux in PhMe) resulted only in a lower yield of this oxime, rather than in situ tricycle formation. However, heating the isolated oxime alone in PhMe gave the desired tricyclic product 27 in excellent yield as a single stereoisomer. The all-cis stereochemistry was confirmed by single crystal X-ray structure analysis (Figure 2) of the amino-alcohol 28, formed after treatment of the product 27 with zinc and acetic acid. This cis stereochemistry is in line with related cyclization–intramolecular cycloaddition reactions leading to the same ring sizes.20 Treatment of the isoxazolidine 27 with sodium amalgam (Na/Hg, Na2HPO4, MeOH) did not remove the phenylthio group but gave the amino-alcohol 28 (80% yield). Other reductive conditions (lithium in liquid ammonia, Raney nickel or Bu3SnH/AIBN) gave only recovered isoxazolidine 27.
Heating the aldehyde 25 with hydroxylamine under reflux in PhMe promoted oxime formation then in situ cyclization and cycloaddition to give the tricyclic product 29. This was formed as a single diastereoisomer and the stereochemistry was confirmed by 1H NOESY studies. Treatment of the sulfone 29 with sodium amalgam gave the amino-alcohol 30. This arises from reduction of the N–O bond together with the loss of the benzenesulfonyl group (the intermediate anion must undergo elimination rather than protonation).
Finally, we converted the amino-alcohol 28 to the 1,3-oxazine 31 using paraformaldehyde and acid. The 1,3-oxazine 31 has the same core structure as the yuzurimine type Daphniphyllum alkaloids daphcalycic acid, daphcalycine and daphcalycinosidine A.25-29
Hence, we have demonstrated that reaction of several different aldehydes (16, 17, 24, 25) with hydroxylamine leads to tricyclic products by a cascade of condensation, cyclization and dipolar cycloaddition. The reaction is stereoselective, providing a single stereoisomer in each case. So far we have not been able to remove the phenylthio group from the products, however the benzenesulfonyl group can be removed using sodium amalgam. The chemistry has provided an efficient total synthesis of (±)-myrioxazine A and a synthesis of the tricyclic core of some yuzurimine type Daphniphyllum alkaloids.
EXPERIMENTAL
2-Benzenesulfonylhept-6-enenitrile 10: n-Butyllithium (4.61 mL, 11.5 mmol, 2.5 M in hexanes) was added to diisopropylamine (1.78 mL, 12.6 mmol) in THF (10 mL) at –78 °C. After 10 min, phenylsulfonylacetonitrile 9 (1.99 g, 11 mmol) was added. After 10 min, 5-bromopentene (1.37 mL, 11 mmol) was added. After 30 min, the mixture was allowed to warm to room temperature and then was heated under reflux. After 18 h, saturated aqueous ammonium chloride solution (10 mL) was added and the mixture was extracted with Et2O (3 × 20 mL). The organic layers were dried (MgSO4) and evaporated. Purification by column chromatography, eluting with petrol–EtOAc (9:1), gave the nitrile 10 (2.02 g, 74%) as an oil [and the dialkylated nitrile (296 mg, 11%) as an oil]; Rf 0.24 [petrol–EtOAc (9:1)]; νmax(film)/cm–1 3075, 2940, 2235, 1640, 1445, 1440, 1320, 1315; 1H NMR (400 MHz, CDCl3) δ = 8.02–7.98 (2H, m, ArH), 7.79–7.74 (1H, m, ArH), 7.67–7.61 (2H, m, ArH), 5.73 (1H, ddt, J 17, 10.5, 6.5, CH=CH2), 5.06–4.98 (2H, m, CH=CH2), 3.92 (1H, dd, J 11, 4.5, CHCN), 2.24–2.07 (3H, m, 3 × CH), 1.90 (1H, dtd, J 13.5, 10.5, 5, CH), 1.80–1.68 (1H, m, CH), 1.63–1.51 (1H, m, CH); 13C NMR (100 MHz, CDCl3) δ = 136.7, 135.6, 135.3, 129.7, 129.6, 116.2, 114.0, 57.4, 32.7, 26.1, 25.7; HRMS (ES) Found: 250.0909, C13H16NO2S requires MH+, 250.0902; LRMS m/z (ES) 272 (100%), 250 (90). Data consistent with the literature.30
2-Benzenesulfonyl-2-(3-chloropropyl)hept-6-enenitrile 11: In the same way as the nitrile 10, diisopropylamine (1.83 mL, 12.9 mmol), n-butyllithium (4.79 mL, 11.7 mmol, 2.45 M in hexanes), the nitrile 10 (1.95 g, 7.82 mmol) and 1-bromo-3-chloropropane (2.34 mL, 23.5 mmol) gave, after heating under reflux for 18 h and purification by column chromatography, eluting with petrol–EtOAc (9:1), a mixture of the chloride 11 and the corresponding bromide (ratio 4:1 by 1H NMR spectroscopy). This mixture and LiCl (585 mg, 13.9 mmol) in DMF (15 mL) were heated to 80 °C. After 3 h, the mixture was allowed to cool to room temperature and EtOAc (10 mL) and ice-cold water (10 mL) were added. The organic layer was washed with water (2 × 10 mL), dried (MgSO4) and evaporated. Purification by column chromatography, eluting with petrol–EtOAc (17:3), gave the nitrile 11 (2.26g, 89%) as an oil; Rf 0.43 [petrol–EtOAc (17:3)]; νmax(film)/cm–1 3075, 2940, 2240, 1640, 1445, 1440, 1325, 1310; 1H NMR (400 MHz, CDCl3) δ = 8.93–7.98 (2H, m, ArH), 7.79–7.74 (1H, m, ArH), 7.67–7.61 (2H, m, ArH), 8.70 (1H, ddt, J 17, 10.5, 6.5, CH=CH2), 5.03–4.96 (2H, m, CH=CH2), 3.61–3.49 (2H, m, CH2Cl), 2.20–1.90 (8H, m, 4 × CH2), 1.71–1.52 (2H, m, CH2); 13C NMR (100 MHz, CDCl3) δ = 136.7, 135.3, 134.3, 130.7, 129.4, 116.6, 116.2, 65.6, 44.0, 33.2, 31.5, 29.5, 27.8, 23.8; HRMS (ES) Found: 326.0992, C16H21ClNO2S requires MH+, 326.0982; LRMS m/z (ES) 328 (20%), 326 (100).
2-Benzenesulfonyl-2-(3-chloropropyl)hept-6-enylamine 12: DIBAL-H (7.8 mL, 7.8 mmol, 1.0 M in hexanes) was added dropwise to the nitrile 11 (1.27 g, 3.89 mmol) in CH2Cl2 (20 mL) at –78 °C. After 1.5 h, aqueous HCl (10 mL, 2 M) was added. After 30 min, the mixture was allowed to warm to room temperature and was extracted with Et2O (40 mL). The organic layer was washed with aqueous HCl (10 mL, 2 M) and the aqueous portions were extracted with Et2O (5 × 75 mL). The organic layers were dried (MgSO4) and evaporated. Purification by column chromatography, eluting with petrol–EtOAc (9:1), gave the nitrile 11 (532 mg, 42%) as an oil. Further elution with CH2Cl2–MeOH (19:1) gave the amine 12 (720 mg, 56%) as an oil; Rf 0.53 [CH2Cl2–MeOH (19:1)]; νmax(film)/cm–1 2945, 2860, 1640, 1445; 1H NMR (400 MHz, CDCl3) δ = 8.02–7.97 (2H, m, ArH), 7.73–7.67 (1H, m, ArH), 7.63–7.57 (2H, m, ArH), 5.71 (1H, ddt, J 17, 10, 7, CH=CH2), 5.01–4.91 (2H, m, CH=CH2), 3.65–3.51 (2H, m, CH2Cl), 3.21 (2H, br s, CH2NH2), 2.09–1.88 (6H, m, 3 × CH2), 1.73–1.66 (2H, m, CH2), 1.58–1.47 (2H, m, CH2); 13C NMR (100 MHz, CDCl3) δ = 137.2, 134.9, 133.8, 130.4, 129.7, 116.0, 65.8, 44.7, 41.6, 33.6, 28.8, 26.2, 26.1, 22.0; HRMS (ES) Found: 330.1294, C16H25ClNO2S requires MH+, 330.1295; LRMS m/z (ES) 332 (13%), 330 (100).
2-(Phenylthio)hept-6-enenitrile 14: n-Butyllithium (5.0 mL, 11.3 mmol, 2.24 M in hexanes) was added to diisopropylamine (1.80 mL, 12.1 mmol) in THF (10 mL) at –78 °C. After 10 min, phenylthioacetonitrile 13 (1.5 mL, 11.2 mmol) was added. After 10 min, 5-bromopentene (1.33 mL, 11.2 mmol) was added. After 30 min, the mixture was allowed to warm to room temperature. After 30 min, saturated aqueous ammonium chloride solution (10 mL) was added and the mixture was extracted with Et2O (3 × 20 mL). The organic layers were dried (MgSO4) and evaporated. Purification by column chromatography, eluting with petrol–EtOAc (97:3), gave the nitrile 14 (1.94 g, 79%) as an oil [and the dialkylated nitrile (243 mg, 15%) as an oil]; Rf 0.53 [petrol–EtOAc (9:1)]; νmax(film)/cm–1 3080, 2930, 2860, 2240, 1640, 1475, 1440; 1H NMR (400 MHz, CDCl3) δ = 7.61–7.58 (2H, m, ArH), 7.41–7.37 (3H, m, ArH), 5.76 (1H, ddt, J 17, 10.5, 6.5, CH=CH2), 5.06–4.97 (2H, m, CH=CH2), 3.69 (1H, t, J 7.5, CH), 2.10 (2H, qt, J 6.5, 1, CH2), 1.88–1.81 (2H, m, CH2), 1.72–1.63 (2H, m, CH2); 13C NMR (100 MHz, CDCl3) δ = 137.3, 134.5, 129.55, 129.5, 129.4, 119.2, 115.7, 37.0, 32.8, 31.8, 26.1; HRMS (ES) Found: 218.0999, C13H16NS requires MH+, 218.1003; LRMS m/z (ES) 218 (100%).
2-(3-Chloropropyl)-2-(phenylthio)hept-6-enenitrile 15: In the same way as the nitrile 14, diisopropylamine (1.14 mL, 8.06 mmol), n-butyllithium (3.5 mL, 7.6 mmol, 2.15 M in hexanes), the nitrile 14 (1.095 g, 5.04 mmol) and 1-bromo-3-chloropropane (1.49 mL, 15.1 mmol) gave, after purification by column chromatography, eluting with petrol–EtOAc (24:1), the nitrile 15 (1.38 g, 93%) as an oil; Rf 0.53 [petrol–EtOAc (9:1)]; νmax(film)/cm–1 3075, 2950, 2855, 2240, 1640, 1475, 1440; 1H NMR (400 MHz, CDCl3) δ = 7.68–7.63 (2H, m, ArH), 7.49–7.37 (3H, m, ArH), 5.75 (1H, ddt, J 17, 10.5, 6.5, CH=CH2), 5.06–4.96 (2H, m, CH=CH2), 3.58–3.52 (2H, m, CH2Cl), 2.15–1.82 (6H, m, 3 × CH2), 1.78–1.59 (4H, m, 2 × CH2); 13C NMR (100 MHz, CDCl3) δ = 137.3, 137.1, 130.4, 129.3, 129.0, 120.8, 115.7, 48.8, 44.2, 36.2, 34.2, 33.2, 27.7, 23.7; HRMS (ES) Found: 294.1081, C16H21ClNS requires MH+, 294.1083; LRMS m/z (ES) 296 (20%), 294 (100).
2-(3-Chloropropyl)-2-(phenylthio)hept-6-enal 16: In the same way as the amine 12, the nitrile 15 (3.43 g, 11.7 mmol) and DIBAL-H (18.7 mL, 18.7 mmol, 1.0 M in hexanes) gave, after purification by column chromatography, eluting with petrol–EtOAc (49:1), the aldehyde 16 (3.12 g, 90%) as an oil; Rf 0.53 [petrol–EtOAc (9:1)]; νmax(film)/cm–1 3075, 2940, 2860, 1715, 1640, 1475, 1440; 1H NMR (400 MHz, CDCl3) δ = 9.32 (1H, s, CHO), 7.39–7.25 (5H, m, ArH), 5.75 (1H, ddt, J 17, 10.5, 6.5, CH=CH2), 5.05–4.96 (2H, m, CH=CH2), 3.51 (2H, t, J 6, CH2Cl), 2.10–2.00 (3H, m, CH2 and CH), 1.31–1.51 (6H, m, 3 × CH2), 1.34–1.23 (1H, m, CH); 13C NMR (100 MHz, CDCl3) δ = 194.9, 137.6, 136.9, 129.8, 129.1, 128.8, 115.5, 63.0, 44.7, 33.7, 29.5, 26.9, 26.6, 23.2; HRMS (ES) Found: 297.1088, C16H22ClOS requires MH+, 297.1080; LRMS m/z (ES) 299 (20%), 297 (100).
2-Benzenesulfonyl-2-(3-chloropropyl)hept-6-enal 17: To the aldehyde 16 (2.25 g, 7.59 mmol) in acetone (30 mL) at room temperature was added Oxone® (11.7 g, 19.0 mmol) in water (54 mL). After 5 h, water (30 mL) and CH2Cl2 (50 mL) were added. The mixture was extracted with CH2Cl2 (2 × 20 mL), dried (MgSO4) and evaporated. Purification by column chromatography, eluting with petrol–EtOAc (9:1), gave the aldehyde 17 (1.82 g, 73%) as an oil; Rf 0.47 [petrol–EtOAc (17:3)]; νmax(film)/cm–1 3070, 2955, 2870, 1725, 1640, 1445, 1305, 1140; 1H NMR (400 MHz, CDCl3) δ = 9.66 (1H, s, CHO), 7.74–7.66 (3H, m, ArH), 7.58–7.52 (2H, m, ArH), 5.72 (1H, ddt, J 17, 10, 7, CH=CH2), 5.05–4.96 (2H, m, CH=CH2), 3.52 (2H, t, J 6, CH2Cl), 2.15–1.93 (7H, m, 3 × CH2 and CH), 1.79–1.68 (1H, m, CH), 1.61–1.48 (1H, m, CH), 1.32–1.19 (1H, m, CH); 13C NMR (100 MHz, CDCl3) δ = 196.5, 137.0, 135.5, 134.6, 129.6, 129.3, 116.0, 76.3, 44.7, 33.8, 26.2, 25.7, 24.2, 22.3; HRMS (ES) Found: 351.0801, C16H21ClNaO3S requires MNa+, 351.0798; LRMS m/z (ES) 353 (6%), 351 (100).
(2aR*,5aR*,8bS*)-5a-(Phenylthio)octahydro-1-oxa-8a-azaacenaphthylene 18: The aldehyde 16 (302 mg, 1.02 mmol), hydroxylamine hydrochloride (108 mg, 1.53 mmol) and N,N-diisopropylethylamine (0.53 mL, 3.05 mmol) in PhMe (10 mL) were heated under reflux. After 2 h, the solvent was evaporated. Purification by column chromatography, eluting with petrol–EtOAc (3:2), gave the cycloadduct 18 (269 mg, 96%) as an oil, which solidified on standing; mp 97–99 °C; Rf 0.44 [petrol–EtOAc (1:1)]; νmax(film)/cm–1 3080, 2930, 2860, 1470; 1H NMR (500 MHz, CDCl3) δ = 7.57–7.53 (2H, m, ArH), 7.38–7.29 (3H, m, ArH), 4.21 (1H, dd, J 8, 6.5, CH), 3.38 (1H, dd, J 10, 6.5, CH), 3.27–3.23 (1H, m, CH), 2.76 (1H, d, J 11.5, CH), 2.67 (1H, ddd, J 14, 10.5, 3.5, CH), 2.62–2.46 (2H, m, 2 × CH), 1.96–1.90 (1H, m, CH), 1.82–1.71 (3H, m, 3 × CH), 1.68–1.61 (2H, m, 2 × CH), 1.38–1.28 (2H, m, 2 × CH), 0.98 (1H, qd, J 12.5, 3.5, CH); 13C NMR (125 MHz, CDCl3) δ = 137.9, 130.8, 130.0, 128.6, 71.2, 69.8, 53.2, 51.4, 39.3, 38.7, 26.6, 26.0, 23.4, 21.8; HRMS (ES) Found: 276.1427, C16H22NOS requires MH+, 276.1422; LRMS m/z (ES) 276 (100%); Anal. Calcd for C16H21NOS: C, 69.78; H, 7.69; N, 5.09; S, 11.64. Found: C, 69.73; H, 7.93; N, 5.04; S, 11.71%.
((4aR*,8R*,8aS*)-4a-(Phenylthio)decahydroquinolin-8-yl)-methanol 19: Zn powder (135 mg, 2.07 mmol) was added to the cycloadduct 18 (95 mg, 0.35 mmol) in AcOH–H2O (1:2, 3 mL) and the mixture was heated at 70 °C. After 1.5 h, the mixture was allowed to cool to room temperature, filtered, washed with CH2Cl2 and the solvent was evaporated. The residue was partioned between ammonia solution (5 mL) and CH2Cl2 (10 mL). The aqueous layer was extracted with CH2Cl2 (3 × 15 mL), and the organic fractions were dried (MgSO4), filtered and evaporated to give the amino-alcohol 19 (96 mg, 100%) as an oil which solidified on standing; mp 50–52 °C; Rf 0.13 [CH2Cl2–MeOH (19:1)]; νmax(film)/cm–1 3385, 2925, 2850, 1475; 1H NMR (500 MHz, CDCl3) δ = 7.48–7.44 (2H, m, ArH), 7.40–7.31 (3H, m, ArH), 3.57 (1H, dd, J 10.5, 3.5, CH), 3.39 (1H, t, J 10.5, CH), 2.91–2.85 (2H, m, 2 × CH), 2.59 (1H, d, J 11, CH), 2.26–2.08 (3H, m, 3 × CH), 1.65–1.53 (2H, m, 2 × CH), 1.52–1.36 (5H, m, 5 × CH), 0.66 (1H, qd, J 12.5, 4.5, CH); 13C NMR (125 MHz, CDCl3) δ = 137.8, 130.6, 129.1, 128.7, 70.6, 63.2, 54.7, 39.2, 39.1, 35.3, 28.1 (2 × CH2), 22.2, 21.2; HRMS (ES) Found: 278.1565, C16H24NOS requires MH+, 278.1579; LRMS m/z (ES) 278 (100%).
(2aR*,5aR*,8bS*)-5a-Benzenesulfonyloctahydro-1-oxa-8a-azaacenaphthylene 20: The aldehyde 17 (260 mg, 0.79 mmol), hydroxylamine hydrochloride (73 mg, 1.03 mmol) and N,N-diisopropylethylamine (0.36 mL, 2.06 mmol) in PhMe (10 mL) were heated under reflux. After 1 h, the solvent was evaporated. Purification by column chromatography, eluting with petrol–EtOAc (1:1), gave the cycloadduct 20 (226 mg, 93%) as a solid, which recrystallised from CH2Cl2–Et2O–petrol as cubes; mp 192–193 °C (dec); Rf 0.41 [petrol–EtOAc (1:1)]; νmax(film)/cm–1 2935, 2870, 1445, 1305, 1280; 1H NMR (500 MHz, CDCl3) δ = 7.98–7.95 (2H, m, ArH), 7.67–7.62 (1H, m, ArH), 7.57–7.53 (2H, m, ArH), 4.18 (1H, dd, J 8.5, 6.5, CH), 3.37 (1H, dd, J 10.5, 6.5, CH), 3.20 (1H, d, J 11.5, CH), 3.18–3.14 (1H, m, CH), 2.65–2.53 (2H, m, 2 × CH), 2.36 (1H, qt, J 14, 5, CH), 2.03–1.93 (2H, m, 2 × CH), 1.87 (1H, td, J 13.5, 4.5, CH), 1.83–1.72 (2H, m, 2 × CH), 1.69–1.72 (1H, m, CH), 1.59–1.53 (1H, m, CH), 1.36 (1H, qt, J 13.5, 3.5, CH), 1.02 (1H, qd, J 12.5, 3.5, CH); 13C NMR (125 MHz, CDCl3) δ = 136.1, 133.8, 130.7, 128.8, 69.8, 67.8, 63.8, 49.7, 38.9, 31.4, 25.7, 22.6, 21.9, 21.1; HRMS (EI) Found: 307.1247, C16H21NO3S requires M+, 307.1242; LRMS m/z (EI) 307 (21%), 165 (100); Anal. Calcd for C16H21NO3S: C, 62.51; H, 6.89; N, 4.56; S, 10.43. Found: C, 62.25; H, 6.93; N, 4.26; S, 10.61%. X-Ray data deposited at CCDC 827991.
(4aS*,8R*,8aR*)-1-(Decahydroquinolin-8-yl)methanol 21: Freshly crushed sodium mercury amalgam (3.58 g) was added to cycloadduct 20 (161 mg, 0.52 mmol) and Na2HPO4 (595 mg, 4.2 mmol) in MeOH (10 mL) at –15 °C, then the mixture was allowed to warm to 0 °C. After 2 h, the mixture was allowed to warm to room temperature. After 2 h, aqueous ammonium hydroxide solution (10%, 10 mL) and CH2Cl2 (20 mL) were added and the mixture was extracted with CH2Cl2 (2 × 15 mL). The organic portions were dried (MgSO4) and evaporated. Purification by chromatography, eluting with CH2Cl2–MeOH–NH3 (8:2:0.1), gave a small amount of the desulfonylated cycloadduct (10 mg, 11%) and the amino-alcohol 21 (79 mg, 89%) as a solid; mp 84–86 °C; Anal. Calcd for C10H19NO: C, 70.96; H, 11.31; N, 8.28. Found: C, 70.62; H, 11.74; N, 8.15%; data as reported.22
Myrioxazine A: The amino-alcohol 21 (78 mg, 0.46 mmol), paraformaldehyde (55 mg, 1.84 mmol) and TsOH·H2O (5 mg, 0.02 mmol) in CHCl3 (3 mL) were heated under reflux. After 12 h, the mixture was allowed to cool to room temperature and H2O (5 mL) and potassium carbonate (0.5 g) were added. The mixture was extracted with CH2Cl2 (2 × 10 mL) and the organic layer was dried (MgSO4) and evaporated. Purification by column chromatography, eluting with EtOAc–MeOH (9:1), gave myrioxazine A (79 mg, 95%) as an oil; Rf 0.43 [EtOAc–MeOH (9:1)]; HRMS (ES) Found: 182.1550, C11H20NO requires MH+, 182.1545; data as reported.22,23
2-(2-Hydroxyethyl)-2-(phenylthio)hept-6-enenitrile 22: n-Butyllithium (3.00 mL, 7.5 mmol, 2.5 M in hexanes) was added to diisopropylamine (1.15 mL, 8.20 mmol) in THF (10 mL) at –78 °C. After 10 min, the nitrile 14 (1.55 g, 7.13 mmol) was added. After 10 min, the 2-bromoethyl trimethylsilyl ether (1.55 g, 7.85 mmol) was added and the mixture was allowed to warm to room temperature. After 1 h, HCl (10 mL, 2 M) was added. After 1 h, the mixture was extracted with Et2O (3 × 30 mL). The organic layers were dried (MgSO4) and evaporated. Purification by column chromatography, eluting with petrol–EtOAc (4:1), gave the nitrile 22 (1.99 g, 89%) as an oil; Rf 0.30 [petrol–EtOAc (4:1)]; νmax(film)/cm–1 3450, 2935, 2250, 1475, 1440; 1H NMR (400 MHz, CDCl3) δ = 7.73–7.67 (2H, m, ArH), 7.53–7.41 (3H, m, ArH), 5.78 (1H, ddt, J 17, 10, 6.5, HC=CH2), 5.09–4.98 (2H, m, HC=CH2), 3.96 (2H, t, J 6.5, CH2), 2.17–1.98 (4H, m, 2 × CH2), 1.91–1.62 (4H, m, 2 × CH2); 13C NMR (100 MHz, CDCl3) δ = 137.4, 137.1, 130.4, 129.3, 128.9, 120.8, 115.6, 59.1, 47.6, 39.1, 36.8, 33.2, 23.9; HRMS (EI) Found: 261.1196, C15H19NOS requires M+, 261.1187; LRMS m/z (EI) 261 (10%), 110 (100).
2-(2-Chloroethyl)-2-(phenylthio)hept-6-enenitrile 23: The alcohol 22 (1.65 g, 6.30 mmol) was added to PPh3 (2.48 g, 9.50 mmol) and freshly recrystallised N-chlorosuccinimide (0.93 g, 6.90 mmol) in THF (12 mL) at 0 °C and the mixture was allowed to warm to room temperature. After 16 h, the solvent was evaporated, the residue was partitioned between Et2O and water, and the mixture was extracted with Et2O (3 × 30 mL). The organic layers were dried (MgSO4) and evaporated. Petrol was added, the mixture was filtered over Celite® and the solvent was evaporated. Purification by column chromatography, eluting with petrol–EtOAc (99:1), gave the chloride 23 (1.41 g, 80%) as an oil; Rf 0.20 [petrol–EtOAc (99:1)]; νmax(film)/cm–1 2935, 2230, 1475, 1440; 1H NMR (250 MHz, CDCl3) δ = 7.77–7.58 (2H, m, ArH), 7.57–7.37 (3H, m, ArH), 5.79 (1H, ddt, J 17, 10, 6.5, HC=CH2), 5.11–4.97 (2H, m, HC=CH2), 3.82–3.63 (2H, m, CH2Cl), 2.30–2.19 (2H, m, CH2), 2.17–2.04 (2H, m, CH2), 1.85–1.61 (4H, m, 2 × CH2); 13C NMR (100 MHz, CDCl3) δ = 137.2, 137.1, 130.7, 129.5, 129.3, 120.0, 115.8, 48.1, 39.5, 38.9, 36.5, 33.1, 23.9; HRMS (ES) Found: 279.0861, C15H18ClNS requires MH+, 279.0848; LRMS m/z (ES) 304 (10%), 302 (100), 301 (40).
2-(2-Chloroethyl)-2-(phenylthio)hept-6-enal 24: In the same way as the amine 12, the nitrile 23 (1.40 g, 5.04 mmol) and DIBAL-H (11.4 mL, 11.4 mmol, 1.0 M in hexanes) gave, after purification by column chromatography, eluting with petrol–EtOAc (99:1), the aldehyde 24 (0.78 g, 55%) as an oil; Rf 0.20 [petrol–EtOAc (99:1)]; νmax(film)/cm–1 2935, 1715, 1560, 1475, 1440; 1H NMR (400 MHz, CDCl3) δ = 9.32 (1H, s, CHO), 7.44–7.33 (5H, m, ArCH), 5.80 (1H, ddt, J 17, 10, 6.5, HC=CH2), 5.09–5.01 (2H, m, HC=CH2), 3.79 (1H, td, J 10.5, 5.5, CH), 3.58 (1H, td, J 10.5, 5.5, CH), 2.20–1.99 (4H, m, 2 × CH2), 1.73–1.60 (3H, m, 3 × CH), 1.38–1.32 (1H, m, CH); 13C NMR (100 MHz, CDCl3) δ = 193.9, 137.4, 137.0, 130.0, 129.3, 128.2, 115.7, 62.4, 39.5, 33.6, 32.3, 29.6, 23.4; HRMS (EI) Found: 282.0845, C15H19ClOS requires M+, 282.0845; LRMS m/z (ES) 307 (20%), 305 (100), 283 (10).
2-(2-Chloroethyl)-2-(phenylsulfonyl)hept-6-enal 25: In the same way as the sulfone 17, the aldehyde 24 (3.46 g, 12.30 mmol) and Oxone® (18.0 g, 29.4 mmol) gave, after purification by column chromatography, eluting with petrol–EtOAc (49:1), the aldehyde 25 (1.03 g, 27%) as an oil; Rf 0.20 [petrol–EtOAc (49:1)]; νmax(film)/cm–1 2920, 2850, 1725, 1445, 1305; 1H NMR (250 MHz, CDCl3) δ = 9.68 (1H, s, CHO), 7.80–7.71 (3H, m, ArCH), 7.66–7.57 (2H, m, ArCH), 5.76 (1H, ddt, J 17, 10, 6.5, HC=CH2), 5.12–5.00 (2H, m, HC=CH2), 3.94–3.81 (1H, m, CHCl), 3.64–3.50 (1H, m, CHCl), 2.42–2.32 (2H, m, CH2), 2.22–1.96 (4H, m, 2 × CH2), 1.68–1.48 (1H, m, CH), 1.40–1.20 (1H, m, CH); 13C NMR (100 MHz, CDCl3) δ =195.4, 136.8, 135.2, 134.8, 129.6, 129.4, 116.2, 76.3, 38.5, 33.6, 30.3, 26.0, 22.5; HRMS (ES) Found: 315.0817, C15H20ClO3S requires MH+, 315.0822; LRMS m/z (ES) 315 (100%).
2-(2-Chloroethyl)-2-(phenylthio)hept-6-enal oxime 26: The aldehyde 24 (0.50 g, 1.80 mmol), hydroxylamine hydrochloride (0.18 g, 2.70 mmol) and iPr2NEt (0.92 mL, 5.30 mmol) in PhMe (17 mL) were heated under reflux. After 16 h, the mixture was adsorbed onto silica. Purification by column chromatography, eluting with CH2Cl2–MeOH (49:1), gave the oxime 26 (0.34 g, 66%) as a mixture of isomers [(E):(Z) ratio 1:1] as an oil; Rf 0.3 [CH2Cl2–MeOH (49:1)]; νmax(film)/cm–1 3385, 2935, 1570, 1460; 1H NMR (400 MHz, CDCl3) δ = 7.60–7.53 (2H, m, ArH), 7.49–7.38 (3H, m, ArH), 6.83 (0.5H, d, J 1.5, HC=N), 6.83 (0.5H, d, J 1.5, HC=N), 5.80 (1H, ddt, J 17, 10, 7, HC=CH2), 5.09–4.98 (2H, m, HC=CH2), 3.73–3.60 (1H, m, CHCl), 3.18–3.06 (1H, m, CHCl), 2.44–2.36 (2H, m, CH2), 2.15–2.09 (2H, m, CH2), 1.88–1.43 (4H, m, 2 × CH2); 13C NMR (100 MHz, CDCl3) δ = 138.3, 137.6, 137.3, 130.0, 129.9, 129.3, 115.6, 61.8, 61.4, 38.1, 33.5, 32.8, 24.2; m/z could not be obtained.
(4aR*,7aS*,7bS*)-4a-Phenylthio-octahydro-2-oxa-2a-aza-cyclopenta[cd]indene 27: The oxime 26 (470 mg, 1.56 mmol) in PhMe (16 mL) was heated under reflux. After 16 h, the mixture was adsorbed onto silica. Purification by column chromatography, eluting with CH2Cl2–MeOH (99:1), gave the cycloadduct 27 (400 mg, 98%) as an oil; Rf 0.30 [CH2Cl2–MeOH (99:1)]; νmax(film)/cm–1 2935, 1440, 1250; 1H NMR (500 MHz, CDCl3) δ = 7.52–7.49 (2H, m, ArH), 7.37–7.30 (3H, m, ArH), 3.84 (1H, t, J 8, CH), 3.60–3.53 (3H, m, 3 × CH), 3.34 (1H, ddd, J 13.5, 6.5, 2, CH), 2.84 (1H, tq, J 10, 3.5, CH), 2.08 (1H, ddd, J 13.5, 11, 6.5, CH), 1.95–1.87 (2H, m, CH2), 1.80 (1H, ddd, J 13.5, 6.5, 2, CH), 1.66–1.54 (2H, m, CH2), 1.53–1.46 (1H, m, CH), 1.44–1.36 (1H, m, CH); 13C NMR (125 MHz, CDCl3) δ = 137.0, 132.0, 129.0, 128.7, 72.2, 70.7, 57.7, 56.4, 41.7, 37.7, 35.6, 25.0, 17.4; HRMS (ES) Found: 262.1270, C15H20NOS requires MH+, 262.1266; LRMS m/z (ES) 262 (100%).
((3aR*,7S*,7aS*)-3a-(Phenylthio)octahydro-1H-indol-7-yl)methanol 28: In the same way as the alcohol 19, Zn powder (200 mg, 3.04 mmol) and the cycloadduct 27 (450 mg, 1.71 mmol) in AcOH–H2O (1:2, 9 mL) gave, after heating at 70 °C for 5 h, the amino-alcohol 28 (400 mg, 98%) as needles; mp 135–137 °C; Rf 0.1 [CH2Cl2–MeOH (1:1)]; νmax(film)/cm–1 3280, 3055, 2940, 1445, 1435, 1260; 1H NMR (500 MHz, CDCl3) δ = 7.54–7.45 (2H, m, ArH), 7.37–7.27 (3H, m, ArH), 3.70 (1H, dd, J 11, 5, CH), 3.59 (1H, dd, J 11, 5, CH), 3.04–2.97 (2H, m, 2 × CH), 2.91 (1H, td, J 10, 3.5, CH), 2.35 (2H, bs, OH, NH), 2.20–2.09 (2H, m, 2 × CH), 1.84 (1H, qt, J 13.5, 4, CH), 1.76–1.70 (1H, m, CH), 1.59–1.48 (3H, m, 3 × CH), 1.42 – 1.32 (2H, m, 2 × CH); 13C NMR (125 MHz, CDCl3) δ = 136.6, 131.9, 128.7, 128.7, 66.5, 63.3, 56.0, 43.3, 41.0, 37.0, 30.9, 22.6, 21.4; HRMS (ES) Found: 264.1418, C15H22NOS requires MH+, 264.1422; LRMS m/z (ES) 264 (100%); Anal. Calcd for C15H21NOS: C, 68.40; H, 8.04; N, 5.32; S, 12.17. Found: C, 68.40; H, 7.97; N, 5.45; S, 12.09%. X-Ray data deposited at CCDC 827990.
(5aR*,8aS*,8bS*)-5a-(Phenylsulfonyl)heptahydro-2H-isoxazolo[4,3,2-hi]indole 29: The aldehyde 25 (0.58 g, 1.84 mmol), hydroxylamine hydrochloride (0.19 g, 2.76 mmol) and N,N-diisopropylethylamine (0.96 mL, 5.53 mmol) in PhMe (18 mL) were heated under reflux. After 16 h, the mixture was adsorbed onto silica. Purification by column chromatography, eluting with CH2Cl2–MeOH (99:1), gave the cycloadduct 29 (0.32 g, 60%) as an oil; Rf 0.3 [CH2Cl2–MeOH (99:1)]; νmax(film)/cm–1 2935, 1440, 1305, 1250; 1H NMR (500 MHz, CDCl3) δ = 7.95–7.90 (2H, m, ArH), 7.71–7.66 (1H, m, ArH), 7.61–7.55 (2H, m, ArH), 4.35 (1H, d, J 8, CH), 3.97 (1H, t, J 8, CH), 3.56 (1H, dd, J 8, 3, CH), 3.36–3.29 (1H, m, CH), 3.25–3.14 (1H, m, CH), 3.07–2.98 (1H, m, CH), 2.41 (1H, ddd, J 14, 6, 2, CH), 2.27–2.16 (1H, m, CH), 2.12–2.01 (1H, m, CH), 1.71–1.60 (2H, m, 2 × CH), 1.60–1.30 (3H, m, 3 × CH); 13C NMR (125 MHz, CDCl3) δ = 135.8, 134.0, 130.5, 129.1, 73.2, 71.9, 64.6, 54.4, 41.0, 35.6, 30.3, 26.8, 16.4; HRMS (ES) Found: 294.1167, C15H20NO3S requires MH+, 294.1164; LRMS m/z (ES) 294 (100%).
(3-(2-Aminoethyl)cyclohex-2-enyl)methanol 30: Freshly crushed sodium mercury amalgam (5 g) was added to cycloadduct 29 (200 mg, 0.68 mmol) and Na2HPO4 (0.75 g, 5.28 mmol) in MeOH (11 mL) at 0 °C. The mixture was allowed to warm slowly to room temperature over 16 h, then was filtered, washed with MeOH and the solvent was evaporated. Purification by column chromatography, eluting with CH2Cl2–MeOH (4:1), gave the amino-alcohol 30 (50 mg, 51%) as an oil; Rf 0.2 [CH2Cl2–MeOH (4:1)]; νmax(film)/cm–1 3300, 2935, 1440; 1H NMR (400 MHz, CDCl3) δ = 5.41–5.37 (1H, m, CH), 3.52–3.44 (2H, m, CH2), 2.76 (2H, t, J 6.5, CH2), 2.30 (1H, bs, OH), 2.11 (2H, t, J 6.5, CH2), 1.95–1.88 (2H, m, 2 × CH), 1.85–1.68 (3H, m, 3 × CH), 1.61–1.47 (1H, m, CH), 1.39–1.28 (1H, m, CH); 13C NMR (125 MHz, CDCl3) δ =124.1, 123.5, 66.7, 41.5, 39.4, 38.0, 28.4, 25.2, 21.4; HRMS (EI) Found: 155.1306, C9H17NO requires M+, 155.1310; LRMS m/z (EI) 155 (10%), 125 (76), 108 (63), 95 (100).
(6aR*,9aS*,9bS*)-6a-(Phenylthio)decahydro-[1,3]oxazino[5,4,3-hi]indole 31: Paraformaldehyde (0.09 g, 3.04 mmol) was added to the amino-alcohol 28 (200 mg, 0.76 mmol) and TsOH·H2O (8 mg, 0.04 mmol) in CHCl3 (15 mL) and the mixture was heated under reflux. After 16 h, the mixture was allowed to cool to room temperature and was adsorbed onto silica. Purification by column chromatography, eluting with CH2Cl2–MeOH–NH3 (99.5:0.5:0.05), gave the acetal 31 (150 mg, 72%) as an oil; Rf 0.3 [CH2Cl2–MeOH–NH3 (99.5:0.5:0.05)]; νmax(film)/cm–1 2935, 2855, 1475, 1440, 1275; 1H NMR (400 MHz, CDCl3) δ = 7.54–7.50 (2H, m, ArH), 7.38–7.31 (3H, m, ArH), 4.60 (1H, d, J 7, CH), 3.85 (1H, d, J 11.5, CH), 3.58 (1H, d, J 7, CH), 3.54 (1H, dd, J 11.5, 2.5, CH), 3.00 (1H, td, J 8.5, 2.5 Hz, CH), 2.25 (1H, td, 11.5, 2.5, CH), 2.18–2.11 (2H, m, 2 × CH), 2.00–1.73 (4H, m, 4 × CH), 1.69 (1H, td, J 13, 3, CH), 1.62–1.48 (3H, m, 3 × CH); 13C NMR (125 MHz, CDCl3) δ = 136.0, 132.1, 128.7, 128.4, 85.8, 71.4, 67.3, 55.3, 46.6, 38.5, 33.4, 33.0, 24.4, 22.1; HRMS (ES) Found: 276.1419, C16H22NOS requires MH+, 276.1422. LRMS m/z (ES) 276 (100%).
ACKNOWLEDGEMENTS
We thank the EPSRC, the University of Sheffield, Lilly UK, and AstraZeneca for support of this work. Harry Adams (University of Sheffield) is thanked for X-ray crystallographic analyses.
References
1. K. C. Nicolaou and J. S. Chen, Chem. Soc. Rev., 2009, 38, 2993. CrossRef
2. M. Brasholz, J. M. Macdonald, S. Saubern, J. H. Ryan, and A. B. Holmes, Chem. Eur. J., 2010, 16, 11471. CrossRef
3. M. Brasholz, B. A. Johnson, J. M. Macdonald, A. Polyzos, J. Tsanaktsidis, S. Saubern, A. B. Holmes, and J. H. Ryan, Tetrahedron, 2010, 66, 6445. CrossRef
4. A. C. Flick, M. J. A. Caballero, and A. Padwa, Tetrahedron, 2010, 66, 3643. CrossRef
5. A. C. Flick, M. J. A. Caballero, H. I. Lee, and A. Padwa, J. Org. Chem., 2010, 75, 1992. CrossRef
6. C. J. Stearman, M. Wilson, and A. Padwa, J. Org. Chem., 2009, 74, 3491. CrossRef
7. M. S. Karatholuvhu, A. Sinclair, A. F. Newton, M.-L. Alcaraz, R. A. Stockman, and P. L. Fuchs, J. Am. Chem. Soc., 2006, 128, 12656. CrossRef
8. A. Padwa, Chem. Soc. Rev., 2009, 38, 3072. CrossRef
9. I. Coldham, S. J. Coles, K. M. Crapnell, J.-C. Fernàndez, T. F. N. Haxell, M. B. Hursthouse, J. D. Moseley, and A. B. Treacy, Chem. Commun., 1999, 1757. CrossRef
10. I. Coldham, K. M. Crapnell, J.-C. Fernàndez, J. D. Moseley, and R. Rabot, J. Org. Chem., 2002, 67, 6181. CrossRef
11. I. Coldham, S. M. Pih, and R. Rabot, Synlett, 2005, 1743. CrossRef
12. I. Coldham, B. C. Dobson, A. I. Franklin, and S. R. Fletcher, Tetrahedron Lett., 2007, 48, 873. CrossRef
13. I. Coldham, B. C. Dobson, S. R. Fletcher, and A. I. Franklin, Eur. J. Org. Chem., 2007, 2676. CrossRef
14. I. Coldham, S. Jana, L. Watson, and N. G. Martin, Org. Biomol. Chem., 2009, 7, 1674. CrossRef
15. A. J. M. Burrell and I. Coldham, Curr. Org. Synth., 2010, 7, 312. CrossRef
16. A. I. Franklin, D. Bensa, H. Adams, and I. Coldham, Org. Biomol. Chem., 2011, 9, 1901. CrossRef
17. W. H. Pearson, P. Stoy, and Y. Mi, J. Org. Chem., 2004, 69, 1919. CrossRef
18. W. H. Pearson, J. E. Kropf, A. L. Choy, I. Y. Lee, and J. W. Kampf, J. Org. Chem., 2007, 72, 4135. CrossRef
19. I. Coldham, A. J. M. Burrell, L. E. White, H. Adams, and N. Oram, Angew. Chem. Int. Ed., 2007, 46, 6159. CrossRef
20. A. J. M. Burrell, I. Coldham, L. Watson, N. Oram, C. D. Pilgram, and N. G. Martin, J. Org. Chem., 2009, 74, 2290. CrossRef
21. A. J. M. Burrell, L. Watson, N. G. Martin, N. Oram, and I. Coldham, Org. Biomol. Chem., 2010, 8, 4530. CrossRef
22. A. J. M. Burrell, I. Coldham, and N. Oram, Org. Lett., 2009, 11, 1515. CrossRef
23. V. C. Pham, A. Jossang, A. Chiaroni, T. Sévenet, and B. Bodo, Tetrahedron Lett., 2002, 43, 7565. CrossRef
24. V. C. Pham, A. Jossang, P. Grellier, T. Sévenet, V. H. Nguyen, and B. Bodo, J. Org. Chem., 2008, 73, 7565. CrossRef
25. J. Kobayashi and T. Kubota, Nat. Prod. Rep., 2009, 26, 936. CrossRef
26. H. E. Bitar, V. H. Nguyen, A. Gramain, T. Sévenet, and B. Bodo, Tetrahedron Lett., 2004, 45, 515. CrossRef
27. A. Jossang, H. E. Bitar, V. C. Pham, and T. Sévenet, J. Org. Chem., 2003, 68, 300. CrossRef
28. I. Coldham, L. Watson, H. Adams, and N. G. Martin, J. Org. Chem., 2011, 76, 2360. CrossRef
29. I. Coldham, A. J. M. Burrell, H. D. S. Guerrand, and N. Oram, Org. Lett., 2011, 13, 1267. CrossRef
30. F. Benedetti, F. Berti, S. Fabrissin, and T. Gianferrara, J. Org. Chem., 1994, 59, 1518. CrossRef