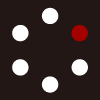
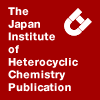
HETEROCYCLES
An International Journal for Reviews and Communications in Heterocyclic ChemistryWeb Edition ISSN: 1881-0942
Published online by The Japan Institute of Heterocyclic Chemistry
e-Journal
Full Text HTML
Received, 8th June, 2011, Accepted, 28th July, 2011, Published online, 5th August, 2011.
DOI: 10.3987/COM-11-S(P)32
■ Synthesis of Nalbuphine from Oripavine via N-Demethylation of N-Cyclobutylmethyl Oripavine
Ales Machara, D. Phillip Cox, and Tomas Hudlicky*
Department of Chemistry, Brock University, St. Catharines, Ontario, L2S 3A1, Canada
Abstract
A short synthesis of nalbuphine from oripavine is reported via N-demethylation of N-cyclobutylmethyloripavine ammonium bromide.INTRODUCTION
Morphine (1), codeine (2), and other medicinally important opiates such as thebaine (3) and oripavine (4), Figure 1, are only available from natural sources. All of the semisynthetic derivatives that are widely used in medicine are prepared from these naturally occurring alkaloids as there is no direct large scale synthetic route available to any of these important compounds at the present time.1 The most efficient synthesis of morphine to date is that of Rice,2 however, the potential cost of this very efficient synthesis at an industrial scale would likely be much higher than the current pricing of morphine derived from the opium poppy (Papaver somniferum) as either opium or morphine concentrate of poppy straw (CPS). The preparation of the C-14 hydroxylated derivatives such as oxycodone and oxymorphone is accomplished on large scales by oxidation of thebaine or oripavine.3 To access those derivatives that contain different alkyl groups on the nitrogen, such as naltrexone, naloxone, nalbuphine, or buprenorphine, N-demethylation followed by alkylation is required. Nalbuphine is a mixed agonist-antagonist, semi-synthetic opioid used commercially as an analgesic under a variety of trade names, including Nubain™. Its analgesic potency is essentially equivalent to that of morphine on a milligram basis. Its onset of action occurs within 2 to 3 minutes after intravenous administration, and in less than 15 minutes following subcutaneous or intramuscular injection. It is noteworthy in part for the fact that at low dosages, it is found much more effective by women than by men, and may even increase pain in men, leading to its discontinuation in the UK in 2003.
The predominant route for the commercial manufacture of the nal- series of active pharmaceutical ingredients (naloxone, naltrexone, nalbuphine) is the alkylation or acylation of noroxymorphone. These synthetic strategies rely on the conversion of oripavine to noroxymorphone and are summarized in Figure 2 along with the projected N-demethylation route.
Comparison of the three commonly used routes to nalbuphone require alkylation (path A), condensation (path B), or acylation (path C) of noroxymorphone 12. Noroxymorphone is in turn synthesized by the N-demethylation of oxymorphone, which, in addition to the N-demethylation step itself requires protection of the C-1 and C-14 hydroxy groups prior to the demethylation and then deprotection after the N-demethylation step. Oxymorphone is prepared from oripavine in two steps. Synthesis of nalbuphone (as well as the related naltrexone and naloxone) by the current methods therefore requires a number of synthetic steps. The routes A, B, and C, depicted in Figure 2 proceed in six, seven, and nine steps from oripavine, respectively.
The step counts shown for the current routes do not necessarily indicate overall efficiency and/or cost evaluation of the processes. However, having a shorter route to nalbuphone that does not rely on either expensive or toxic reagents would seem to be a worthwhile task, especially if better alternatives to the N-demethylation protocols could be developed.
There are many demethylation protocols available in the literature and these range from the von Braun reaction,4 which employs cyanogen bromide, chloroformate-induced demethylations,5 and various oxidative demethylation protocols catalyzed by transition metals.6 There are recent reports in the literature on iron-catalyzed demethylation of N-oxides7 as well as biological protocols that use fungal cytochromes.8 A quaternization/N-demethylation protocol was reported for the synthesis of pergolide from 8,9-dihydroelymoclavine in one-pot.9 In this synthesis, a propyl group was exchanged for an N-methyl group by first quaternizing the tertiary N-methyl group and then selective removal of the N-methyl group using sodium methane-thiolate. Recently, we reported a synthesis of buprenorphine from oripavine via N-demethylation of quaternary salts by sodium thiolate.10 Such protocol allowed for the synthesis of N-cyclopropylmethyl nororipavine that was then be converted to buprenorphine by methods already described in the literature. In this paper we describe a shorter synthesis of nalbuphine, relative to current literature methods, by a similar strategy.
RESULTS AND DISCUSSION
The synthesis of nalbuphine commenced with the quaternization of oripavine with cyclobutylmethyl bromide to produce a mixture of diastereomers (S:R = 3:1) of the ammonium salt 15 in excellent yield as shown in Scheme 1.
The assignment of R- and S- configuration for 15 was made by HPLC in analogy with the elution characteristics of R- and S- cyclopropylmethyl salts of oripavine investigated previously.10 In that case the R-isomer yielded R-methylnaltrexone and was assigned on comparison with an authentic sample. The elution characteristics were as follows: S-isomer: 22 min; R-isomer: 24 min [C18 column, MeOH/phosphate buffer, flow = 1.3 mL/min; 280 nm]. The two isomers of 15, analyzed under identical conditions, gave the following characteristics: S-isomer: 31 min; R-isomer: 34 min.
The N-demethylation of the salt was accomplished with sodium thiolate derived from 1-dodecanethiol in DMSO at elevated temperature providing the desired N-cyclobutylmethyl nororipavine 16 in consistent yields of 60%. Improved yields (73%) of N-cyclobutylmethyl nororipavine were obtained when t-dodecanethiol and t-BuONa were employed for the demethylation in DMSO at 80 °C on a scale of one gram. Oxidation of the diene moiety with peracetic acid11 followed by hydrogenation afforded nalbuphone (9), whose reduction12 furnished nalbuphine (10), identical in all respects with the compound described in the literature.
CONCLUSIONS
A synthesis of nalbuphine from oripavine has been developed requiring only five chemical transformations and proceeding with an overall yield of 45%. Since the conversion of 16 to 9 is normally carried out in a one-pot operation, the potential exists to convert oripavine to nalbuphine in three or at most four unit operations.
EXPERIMENTAL
N-Cyclobutylmethyl oripavine ammonium bromide (15)
To a flame-dried, argon-purged round bottom flask with attached reflux condenser was charged a suspension of oripavine (2.0 g, 6.73 mmol) in anhydrous DMF (10 mL). (Bromomethyl)cyclobutane (3.01 g, 20.2 mmol, 3.0 equiv) was added to the vigorously stirred suspension of oripavine as one portion and at room temperature. The reaction mixture was immersed in a pre-heated oil-bath at 85 °C and allowed to stir under argon atmosphere for 18 h. The reaction mixture was removed from heat and an aliquot was analyzed by HPLC (285 nm) and determined to contain approximately 9.5% (AUC) oripavine (as the HBr salt). Potassium carbonate (88 mg, 0.68 mmol, 9.5 mol%) was added to the reaction mixture and allowed to stir for 1 h prior to the addition of (bromomethyl)cyclobutane (0.5 g, 3.4 mmol, 0.5 equiv) at room temperature. The reaction mixture was immersed in the pre-heated oil-bath at 85 °C for an additional 5 h prior to analysis by HPLC (285 nm). It was observed that approximately 3% of oripavine remained in the reaction mixture. The reaction mixture (fine beige slurry) was cooled to room temperature. The reaction mixture was poured to a vigorously stirred toluene (~ 100 mL). The precipitate was subjected to column chromatography (silicagel, eluent DCM + 15% MeOH), affording 2.65 g (88%) of the title compound (15), as a white solid and a mixture of diastereomers (isomeric ratio determined by HPLC (S:R=3:1). R-isomer: mp 230-233 °C (MeOH); RF 0.39 (DCM + 20% MeOH); [α]20D -77.34 (c 0.5, MeOH:AcOH/1:1); IR (KBr) ν 3004, 2953, 2920, 1590, 1496, 1460, 1300, 1240, 1116, 1012, 928 cm-1; 1H NMR (600 MHz, DMSO) δ 9.37 (s, 1H), 6.62 (d, J=8.2 Hz, 1H), 6.55 (d, J=8.2 Hz, 1H), 6.00 (d, J=6.6 Hz, 1H), 5.41 (s, 1H), 5.28 (d, J=6.7 Hz, 1H), 4.52 (d, J= 7.2, 1H), 3.75 (dd, J=13.7, 6.6 Hz, 1H), 3.69 (d, J= 19.5, 1H), 3.60 (s, 3H), 3.55 (dd, J=13.8, 6.6 Hz, 1H), 3.40 (dd, J=13.2, 4.4 Hz, 1H), 3.29 (ddd, J=13.5, 13.5, 3.9, 1H), 3.05 (s, 3H), 3.00 (dd, J=19.6, 7.3 Hz, 1H), 2.93 (m, 1H), 2.54 (td, J=13.8, 5.1Hz, 1H), 2.16 (m, 2H), 1.91 (m, 3H), 1.84 (dd, J=14.3, 3.0 Hz, 1H), 1.78 (m, 1H); 13C NMR (150 MHz, DMSO) δ 154.6, 143.5, 140.4, 132.3, 124.0, 122.5, 120.2, 119.9, 117.6, 96.0, 87.2, 68.0, 67.5, 55.6, 54.4, 46.0, 44.0, 31.4, 30.3, 29.1, 28.0, 27.9, 19.1; MS (FAB+) m/z (%): 44 (50), 58 (23), 126 (55), 211 (7), 239 (18), 366 (100); HRMS calcd for C23H28NO3+ 366.2064, found 366.2016. S-isomer: mp 185-189 °C (MeOH); RF 0.32 (DCM + 20% MeOH); [α]20D -35.03 (c 1, MeOH); 1H NMR (600 MHz, DMSO) δ 9.37 (s, 1H), 6.62 (d, J=8.0 Hz, 1H), 6.56 (d, J=8.0 Hz, 1H), 5.94 (d, J=6.6 Hz, 1H), 5.39 (s, 1H), 5.27 (d, J=6.6 Hz, 1H), 4.62 (d, J=7.0 Hz, 1H), 3.71 (d, J=19.6 Hz, 1H), 3.60 (m, 1H), 3.60 (s, 3H), 3.46 (dd, J=13.2, 7.2 Hz, 1H), 3.42 (m, 1H), 3.31 (ddd, J=13.5, 13.5, 3.6 Hz, 1H), 3.12 (s, 3H), 3.02 (m, 1H), 2.91 (m, 1H), 2.56 (td, J=14.0, 4.8 Hz, 1H), 2.11 (m, 2H), 1.91 (m, 2H), 1.84 (m, 1H), 1.76 (m, 2H); 13C NMR (150 MHz, DMSO) δ 154.6, 143.4, 140.4, 132.5, 124.1, 122.6, 120.2, 119.7, 117.6, 96.0, 87.2, 68.7, 64.4, 55.6, 54.4, 48.4, 43.6, 31.3, 30.5, 29.3, 28.4, 27.7, 19.0; MS (FAB+) m/z (%): 44 (50), 58(23), 126 (55), 211 (7), 239 (18), 366 (100); HRMS calcd for C23H28NO3+ 366.2064, found 366.2069.
N-Cyclobutylmethyl nororipavine (16)
A. With 1-dodecanethiol and EtONa: To a slurry of sodium ethoxide (0.46 g, 6.82 mmol) in freshly distilled DMSO (4.5 mL) was added dodecanethiol (1.38 g, 6.82 mmol, distilled) in one portion. The resulting mixture was vigorously stirred and immersed in a preheated oil bath at 90 °C for 10 min prior to decreasing the temperature to 80 °C. A solution of N-cyclobutylmethyl oripavine ammonium bromide (1.01 g, 2.27 mmol) in DMSO (4.5 mL) at room temperature was added to the preformed mixture of dodecanethiolate at 80 °C over 10 min. An abrupt color change from a clear, slightly yellow solution to a black colored mixture occurred after the first several drops of the N-cyclobutylmethyl oripavine ammonium bromide solution. The reaction mixture was allowed to stir at 80 °C for 50 min after the addition was completed and monitored by HPLC (285 nm). After consumption of starting material was observed the reaction mixture was allowed to cool to room temperature with stirring and poured into H2O (40 mL). The pH of the aqueous mixture was adjusted to pH = 2 with HCl (6 M) and washed with hexanes (2 x 15 mL). The pH of the aqueous mixture (milky yellow suspension) was adjusted to pH = 8 with NaOH (aq, 15%). A fine, white precipitate was observed upon pH adjustment and was cleared by extraction with EtOAc (1 x 20 mL, 1 x 10 mL). The pH of the aqueous phase was adjusted again to pH = 8 (white precipitate observed) and extracted with EtOAc (3 x 10 mL). The organic layers were combined and washed with H2O (1 x 10 mL) and brine (1 x 10 mL). The organic layers were dried over MgSO4, filtered, and concentrated. The material was purified by chromatography on silica gel (20% MeOH/EtOAc) to afford 0.46 g (58% yield) of N-cyclobutylmethyl nororipavine (13) as a pale-yellow solid.
B. With t-dodecanethiol and t-BuONa: Esentially the same procedure was followed on a scale of 1 gram (2.73 mmol) of N-cyclobutylmethyl oripavine ammonium bromide in DMSO at 80 °C for 50 min. Yield of 16: 0.70 g (73%).
(16): mp 114-116 °C (toluene); RF 0.44 (EtOAc + 20% MeOH); [α]20D -150.06 (c 1, CHCl3); IR (CHCl3) ν 3568, 2962, 2929, 1608, 1507, 1454, 1329, 1263, 1023, 868 cm-1; 1H NMR (600 MHz, CDCl3) δ 6.65 (d, J=8.0 Hz, 1H), 6.55 (d, J=8.0 Hz, 1H), 5.55 (d, J=6.4 Hz, 1H), 5.29 (s, 1H), 5.08 (d, J=6.4 Hz, 1H), 3.73 (d, J=7.0 Hz, 1H), 3.62 (s, 3H), 3.33 (d, J=18.0 Hz, 1H), 2.89 (t, J=11.7 Hz, 1H), 2.71 (m, 4H), 2.60 (hept, J=7.4 Hz, 1H), 2.23 (ddd, J=12.7, 12.7, 4.9 Hz, 1H), 2.10 (m, 2H), 1.91 (m, 1H), 1.81 (m, 1H), 1.74 (pent, J=8.7 Hz, 2H), 1.68 (dd, J=12.4, 1.8 Hz, 1H); 13C NMR (150 MHz, CDCl3) δ 152.2, 143.0, 138.7, 133.1, 132.8, 126.8, 119.8, 116.4, 111.9, 96.4, 89.4, 60.2, 59.0, 55.0, 46.8, 44.1, 36.3, 34.5, 31.2, 27.9, 27.9, 18.9; MS (FAB+) m/z (%): 41 (34), 69 (14), 112 (35), 211 (17), 241 (20), 351 (81), 352 (100); HRMS calcd for C22H26NO3+ 352.1907, found 352.1844.
Nalbuphone (9)
A solution of N-cyclobutylmethyl nororipavine (16) (0.09 g, 0.256 mmol) in 2 mL H2O/HOAc (1:1 v/v) was chilled to 5 °C with stirring. A solution of peracetic acid (0.066 g, 32 wt%) was added dropwise over 1 min. The mixture was allowed to stir at 5 °C for 2 h. The reaction was monitored by TLC (EtOAc + 20% MeOH). The reaction mixture at room temperature was diluted with i-PrOH (2.0 mL), palladium on charcoal (11 mg, 10 wt%) was added, and the reaction mixture subjected to a hydrogen atmosphere (Parr shaker, 50 Psi) for 15 h. The mixture was filtered through a pad of Celite® and washed with i-PrOH. Acetic acid was removed as an azeotrope with toluene prior to concentration to dryness. The material was purified by chromatography on silica gel (EtOAc + 20% MeOH) to afford 0.075 g (82% yield) of nalbuphone (9) as a white solid; mp 168-169 °C (cyclohexane), mp 170-172 °C (acetone), [lit.13 mp 173-174 °C (CHCl3/pentane)]; RF 0.64 (EtOAc + 20% MeOH); [α]20D -180.44 (c 1, MeOH); IR (CHCl3) ν 3561, 3454, 2966, 2931, 2830, 1720, 1616, 1457, 1318, 1142, 1057, 944 cm-1; 1H NMR (600 MHz, CDCl3) δ 6.74 (d, J=8.0 Hz, 1H), 6.61 (d, J=8.0 Hz, 1H), 5.64 (bs, 1H, OH), 4.72 (s, 1H), 3.11 (d, J=18.4 Hz, 1H), 3.04 (ddd, J=14.4, 14.4, 3.6 Hz, 1H), 2.92 (d, J=4.9 Hz, 1H), 2.57 (m, 5H), 2.42 (ddd, J=12.4, 12.4, 4.4 Hz, 1H), 2.33 (d, J=14.4 Hz, 1H), 2.20 (ddd, J=12.0, 12.0, 2.2 Hz, 1H), 2.11 (m, 2H), 1.95 (m, 1H), 1.90 (m, 2H), 1.87 (m, 2H), 1.66 (ddd, J=13.6, 13.6, 2.2 Hz, 1H), 1.56 (d, J=12.6 Hz, 1H); 13C NMR (150 MHz, CDCl3) δ 209.7, 143.5, 138.7, 129.0, 124.3, 119.9, 117.7, 90.6, 70.3, 62.7, 60.5, 50.9, 43.7, 36.2, 33.7, 31.3, 30.7, 27.0, 26.8, 23.0, 18.8; MS (FAB+) m/z (%): 41 (27), 69 (9), 98 (5), 300 (88), 355 (38), 356 (100); HRMS calcd for C21H26NO4+ 356.1856, found 356.1855.
Nalbuphine (10)
The mixture of nalbuphone (0.085 g, 0.239 mmol), Adams catalyst (0.0014 g, 0.006 mmol) in 1.2 mL of solvent system (i-PrOH:water/1:2, pH set up to 12.7) was subjected to hydrogenation in Parr shaker (50 Psi) for 4 h. Then the mixture was diluted with water and pH was adjusted to 9. Product was extracted with EtOAc (4x5 mL). Combined organic layers were washed with brine and dried over MgSO4. The material was purified by chromatography on silica gel (EtOAc + 20% MeOH) to afford 0.058 g (86% yield) of the title compound as a white solid, which was analyzed by HPLC (285 nm) and determined to contain approximately 94% (AUC) nalbuphine; mp >230 °C (EtOH) [lit.14 mp 229-231 °C]; RF 0.64 (EtOAc + 20% MeOH); 1H NMR (600 MHz, DMSO) δ 8.81 (bs, 1H), 6.55 (d, J=8.0 Hz, 1H), 6.42 (d, J=8.0 Hz, 1H), 4.75 (bs, 1H, OH), 4.43 (d, J=5.6 Hz, 1H), 4.38 (d, J=4.5 Hz, 1H), 4.01 (m, 1H), 2.96 (d, J=18.4 Hz, 1H), 2.72 (d, J=6.5 Hz, 1H), 2.53 (dd, J=18.4, 6.6 Hz, 1H), 2.50 (m, 1H), 2.45 (m, 1H), 2.39 (m, 1H), 2.11 (m, 2H), 2.00 (m, 2H), 1.85 (m, 2H), 1.81 (m, 1H), 1.63 (m, 2H), 1.45 (m, 2H), 1.31 (m, 2H), 0.97 (m, 1H); 13C NMR (150 MHz, DMSO) δ 146.5, 138.5, 131.4, 124.9, 118.4, 117.6, 90.7, 69.9, 65.8, 62.6, 60.5, 47.0, 43.5, 33.9, 33.6, 29.3, 26.9, 26.7, 23.5, 23.1, 18.8; MS (FAB+) m/z (%): 41 (17), 154 (5), 302 (41), 340 (18), 358 (100); HRMS calcd for C21H28NO4+ 358.2013, found 358.1974.
ACKNOWLEDGEMENTS
The authors are grateful to the following agencies for financial support of this work: Noramco, Inc., Natural Sciences and Engineering Research Council of Canada (NSERC) (Idea to Innovation and Discovery Grants); Canada Research Chair Program, Canada Foundation for Innovation (CFI), Research Corporation, TDC Research, Inc., TDC Research Foundation, and Brock University. We also thank Dr. Alfred Stutz, Hans-Ulrich Bichsel, and Dr. Harald Hofmeier of the Labor für Prozessforschung, Organische-chemisches Institut, Universität Zürich-Irchel, Winterthurerstrasse 190, CH-8057 Zürich for the provision of optimized procedure for the selective hydrogenation of nalbuphone to 6-alpha-nalbuphine (unpublished work) from a general procedure developed by Bailey & Rezaie (reference 12).
References
1. For reviews of syntheses of morphine alkaloids see: U. Rinner and T. Hudlicky, Top. Curr. Chem., 2012, 309, 33; CrossRef T. Hudlicky and J. W. Reed, ‘The Way of Synthesis: Evolution of Design and Methods for Natural Products,’ Wiley-VCH, Weinheim, 2007, Chapter 4.7, pp. 727-757; J. Zezula and T. Hudlicky, Synlett, 2005, 388; CrossRef D. F. Taber, T. D. Neubert, and M. F. Schlecht, Strategies Tactics Org. Synth., 2004, 5, 353; P. R. Blakemore and J. D. White, Chem. Commun., 2002, 1159; CrossRef B. H. Novak, T. Hudlicky, J. W. Reed, J. Mulzer, and D. Trauner, Curr. Org. Chem., 2000, 4, 343; CrossRef T. Hudlicky, G. Butora, S. P. Fearnley, A. G. Gum, and M. R. Stabile, ‘Studies in Natural Product Chemistry,’ ed. by A. U. Rahman, Elsevier, Amsterdam, 1996, 18, p. 43; M. Maier, ‘Organic Synthesis Highlights II,’ ed. by H. Waldman, VCH, Weinheim, 1995, pp. 357–370. CrossRef
2. K. C. Rice, J. Med. Chem., 1977, 20, 164. CrossRef
3. Reference for the formation of oxymorphone from oripavine: Dung et al. US 7,851,482; Huang, US 20080125592, US 20100274019, US 20110009634; Wang et al. US 20100113787. References for the formation of oxymorphone from thebaine via 14-hydroxycodeinone; Weiss et al. J. Org. Chem., 1957, 22, 1505. CrossRef
4. J. von Braun, Chem. Ber., 1900, 33, 1438. CrossRef
5. J. H. Cooley and E. J. Evain, Synthesis, 1989, 1. CrossRef
6. R. J. Carroll, H. Leisch, E. Scocchera, T. Hudlicky, and D. P. Cox, Adv. Synth. Catal., 2008, 350, 2984. CrossRef
7. G. B. Kok and P. J. Scammells, Org. Biomol. Chem., 2011, 9, 1008; CrossRef G. B. Kok and P. J. Scammells, P. J. Bioorg. Med. Chem. Lett., 2010, 20, 4499; CrossRef G. B. Kok, C. C. Pye, R. D. Singer, and P. J. Scammells, J. Org. Chem., 2010, 75, 4906; CrossRef G. Kok, T. D. Asten, and P. J. Scammells, Adv. Synth. Catal., 2009, 351, 283; CrossRef Z. Dong and P. J. Scammells, J. Org. Chem., 2007, 72, 9881. CrossRef
8. V. Chaudhary, H. Leisch, A. Moudra, B. Allen, V. De Luca, D. P. Cox, and T. Hudlicky, Collect. Czech. Chem. Commun., 2009, 74, 1179. CrossRef
9. J. W. Misner, US 546306.
10. L. Werner, A. Machara, D. R. Adams, D. P. Cox, and T. Hudlicky, J. Org. Chem., 2011, 76, 4628; CrossRef T. Hudlicky, R. Carroll, H. Leisch, A. Machara, L. Werner, and D. R. Adams, PCT Int. Appl., 2010, WO 2010121369, A1 20101028, CAN 153:555396, AN 2010:1342585.
11. See reference 3.
12. T. S. Bailey and R. Rezaie, WO2010017573A1.
13. R. A. Olofson and J. P. Pepe, US 4,161,597.
14. H. Blumberg, US 3332950; http://www.wolframalpha.com/entities/chemicals/nalbuphine/9k/1p/uh/