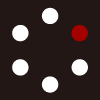
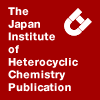
HETEROCYCLES
An International Journal for Reviews and Communications in Heterocyclic ChemistryWeb Edition ISSN: 1881-0942
Published online by The Japan Institute of Heterocyclic Chemistry
e-Journal
Full Text HTML
Received, 9th July, 2011, Accepted, 5th September, 2011, Published online, 13th September, 2011.
DOI: 10.3987/COM-11-S(P)80
■ Dendronized Bi-2-quinoline Ligands and Their Metal Complexes: Dendron Synthesis and Metalloassembly
Anil Patri, Charles N. Moorefield, and George R. Newkome*
Departments of Polymer Science and Chemistry, The University of Akron, Akron, OH 44325-4717, U.S.A.
Abstract
The synthesis of bis-biquinoline ligands possessing G1 and G2 Behera's amine-based dendrons with acid and ester termini was accomplished. Treatment with Cu(I) salts quantitatively generated the desired stable metallodendrimers with a Cu(I) core.INTRODUCTION
Iterative synthetic methodology1 is useful for the construction of dendrons possessing specific functional units at the focal position, that can be used for a variety of purposes, e.g. metal complexation, hydrogen bonding,2 molecular recognition,3-5 and catalysis.6-8 Such construction can be achieved using appropriate tailored functional monomers, spacers, and core units; whereas, characterization of the resultant stable metal complexes can be accomplished using traditional spectroscopic techniques as well as MALDI-TOF mass spectrometry and electrochemistry. These uniform, spherical metallomacromolecules remain essentially monodisperse for the first few generations, beyond which they can deviate from precise construction due to incomplete tier conversion. Such metallodendrimers possess a metal-core, which opens avenues to novel photophysical and redox properties that can be tuned by the metal and ligand choices. The judicious choice of metal core and more importantly the different ligating internal structures, derived from different metal-centered coordination, such as: bipyridines,9-15 phenanthroline,16,17 carboxylate,18-20 imidazolium,21 2,6-diiminopyridine,22 R2PCH2CH2PR2,23 diketiminato,24 8-hydroxyquinoline,25 porphyrin,26 β-diketones,27 phenylpyridine,12,28-37 bis(pyrazolyl)methane,15 α-diimine,38 cyclam,39 2-phenylbenzimid-azole,40,41 bisterpyridine,42-52 and phthalocyanine53 have started to open interesting utilitarian possibilities. These ordered dendritic networks have been shown to have applications6,8,54-58 in a variety of areas of material science, such as: molecular electronics,59 bio-molecular engineering, and liquid crystal engineering.60
Towards this goal, dendrimers with incorporated internal 2,2'-bipyridine units were designed, synthesized,61,62 and shown to form Cu(I) complexes, which possess poor structural stability. Hence, the 2,2'-biquinoline ligand was chosen due to the enhanced stability associated with its tetrahedral metal complexes. Herein, dendronized biquinoline ligands were prepared and transformed to the Cu(I) complexes.
RESULTS AND DISCUSSION
Several attempts were made to construct functionalized 6,6'-disubstituted 2,2'-biquinolines. Commercially available quinoline-6-carboxylic acid (1) was converted to the ethyl ester 2 under Fisher esterification
conditions.63 The attempted coupling of 2 using Pd/C, Rh/C or Raney nickel failed to produce the desired biquinoline; in all cases, unreacted material was recovered. Several attempts for homocoupling of 6-nitro- and 6-acetamidoquinolines were also unsuccessful. Homocoupling of 6-methylquinoline with Rh/C did, however, give 6,6'-dimethyl-2,2'-biquinoline; subsequent oxidation using KMnO4, CrO3 or Na2Cr2O7 failed to generate the desired diacid but rather ring-fragmentation was realized.
Our attention was then redirected to 4,4'-disubstituted 2,2'-biquinoline, in which the desired diacid 3 was precipitated by treating an aqueous solution of 2,2'-biquinoline-4,4'-dicarboxylic acid dipotassium salt with acetic acid. Whereas diacid 3 is insoluble in common organic solvents, treatment with freshly distilled thionyl chloride gave the diacyl dichloride 4, as a light yellow solid that was easily recrystallized from benzene. Its 13C NMR (DMSO-d6) spectrum showed nine distinct aromatic signals and a carbonyl signal at 167.5 ppm. The pure diacyl chloride 4 was then treated with 2.2 equivalents of Behera’s amine 5 in the presence of (i-pr)2EtN in anhydrous THF at 45 °C to give (80-90%) hexaester 6, after column chromatography.
The structure of 6 was established by 13C NMR spectrum, which showed nine distinct aromatic signals along with an amide carbonyl peak at 167.0 ppm and ester carbonyl peak at 172.5 ppm, apart from the other expected signals for the carbons of dendritic arms. There is a remarkable shift of the aromatic protons of the hexaester 6 in that the two doublets of the starting diacyl chloride at 8.6 and 8.4 ppm corresponding to the 8,8'- and 5,5'-positions of biquinoline ring shifted upfield to overlap and appear at 8.3 ppm due to the change in the electron-withdrawing nature of the juxtaposed carbonyl group. Molecular ion at m/z 1139.8 in the electrospray mass spectrum of 6 provides further conclusive evidence for the proposed structure.
Deprotection of the peripheral tert-butyl groups of 6 was achieved by treatment with formic acid at 25 °C for 24 h to afford (78%) hexaacid 7. Residual formic acid was removed by washing with MeOH. The structure of 7 was confirmed by 13C NMR (D2O/NaOD) spectrum, which shows a new signal at 182.5 ppm for the acid carbonyl carbon and disappearance of the signals associated with tert-butyl group at 28, 80, and 172.5 ppm.
The hexaacid 7 was coupled with amine 5 in the presence of DCC and 1-HBT64 in dry DMF to afford (60%) the 2nd generation 18-ester 8. Evidence for the formation of 8 was obtained by 13C NMR, which shows (1) a broadening of signals, (2) differences in the relative intensities of the aliphatic vs. aromatic signals, and (3) the presence of two signals at 59.1 and 57.5 ppm, corresponding to the 4°CNH of the 1st and 2nd generations, respectively, as well as the 172.7 ppm absorption for CO2tBu. Absence of the distinctive carbonyl signal at 182.5 ppm for the acid moieties also supported the transformation. The 18-ester 8 was treated with formic acid to give the desired 18-acid 9, which has the same structural characteristics as the hexaacid except for the additional signals for the new generation. The signals for 9 are broader and, unlike hexaacid 7, 9 is soluble in MeOH. The three-carbonyl signals from inside towards the periphery of the molecule appear at 169.3, 175.6, and 177.4 ppm, respectively.
The Cu(I) complexes of dendritic ester ligands 6 and 8 were prepared by reacting each with one equivalent of [Cu(MeCN)4]BF4 in MeCN solution to form complexes 10 and 11, respectively (Scheme 2). These stable purple complexes were characterized by 13C and 1H NMR, UV-VIS, and ESI-MS. There is a significant chemical shift (1H NMR) for the 8- and 8'-protons of the biquinoline ring upon complexation. One of the doublets that appears at δ 8.3 in the free ligand shifts upfield to δ 7.7 and overlaps with NH proton, which shifted downfield from δ 6.9 upon complexation. The method of continuous variation was used to confirm the formation of 2:1 complex; the free 2,2'-biquinoline and dendritic biquinoline ligand were treated with [Cu(MeCN)4]BF4 (Figure 1) in MeCN at 25 °C. The absorbance due to complexation was measured in the visible region at 540 nm with an increasing ratio of metal. The maximum absorbance was observed at 2:1 ratio of ligand to Cu metal confirming the formation of shown complex. ESI-MS spectra of the complexes also confirm the proposed structures.
CONCLUSION
In summary, the potential to incorporate biquinoline ligands into dendritic architectures by using it as a branching core capable of subsequent metal coordination has been demonstrated. Facile generation of the dendritic complexes was accomplished in high yield by treatment of the branched biquinolines with [Cu(MeCN)4]BF4. Their characterization, as well as that of the intermediates, was achieved by standard NMR and MS methods. Confirmation of complex formation for the dendritic, bisbiquinoline-Cu(I) species was obtained by observation of the UV absorption spectra at 540 nm for differing ratios of 1st generation, dendritic biquinoline 6 and metal and comparison to the spectra obtained with the ligand and metal.
EXPERIMENTAL
The 2,2'-biquinoline-4,4'-dicarboxylic acid dipotassium salt trihydrate was purchased from Aldrich and used directly. The salt [Cu(MeCN)4]PF6 was purchased from Aldrich and recrystallized from MeCN, prior to use. For the size exclusion column, Bio-beads (100) were purchased from Bio-rad laboratories and the polymer was swollen in MeCN for 12h and packed in a narrow column.
6-Cascade:2,2'-biquinoline-4,4':(2-oxo-3-azaethylidyne):tert-butyl Propanoate (6): To a stirred solution of diacid chloride 4 (800 mg, 2.09 mmol) in dry THF (100 mL), a solution of (i-pr)2EtN (596 mg, 4.61 mmol), Behera’s amine65,66 (5; 1.92 g, 4.6 mmol) in THF (20 mL) was added slowly with stirring. The mixture was heated to 45 °C for 12 h under N2, and then cooled to 25 °C. After the solvent was removed in vacuo, the white residue was dissolved in EtOAc, washed successively with sat. K2CO3, distilled H2O, saturated brine, and the organic layer dried (Na2SO4). The solvent removed in vacuo to give a white residue, which was chromatographed (Al2O3) with CH2Cl2 to give (88%) pure 6: 2.1 g; mp 82-85 °C; 1H NMR δ 1.33 (s, CH3, 54H), 2.16 (t, CH2CH2CO, 12H), 2.35 (t, CH2CO, 12H), 6.99 (s, NH, 2H), 7.54 (t, 7,7'-ArH, 3J = 7.5 Hz, 2H), 7.70 (t, 6,6'-ArH, 3J = 7.5 Hz, 2H), 8.19 (dd, 5,5'- & 8,8'-ArH, 4H), 8.71 (s, 3,3'-ArH, 2H); 13C NMR 28.0 (CH3), 29.3, 29.8 (CH2CH2CO2), 58.6 (4°CNH), 80.4 OC(CH3)3, 115.9, 124.5, 125.1, 127.8, 129.6, 130.0, 142.9, 147.9, 154.6 (CAr), 167.0 (CONH), 172.5 (CO2tBu); ESI-MS m/z 1139.8 (M+ + 1). Anal. Calcd for C64H90N4O14 (1138.64): C, 67.46; H, 7.96; N, 4.92. Found: C, 67.40; H, 7.88; N, 4.88.
6-Cascade:2,2'-biquinoline-4,4':(2-oxo-3-azaethylidyne):propanoic Acid (7). A stirred mixture of hexaester 6 (2g, 1.75 mmol) and freshly distilled formic acid (20 mL) was maintained at 25 °C for 12 h. Formic acid was removed in vacuo, and the residue washed with cold MeOH to give (78%) pure hexaacid 7: 1.1 g; 1H NMR (CD3OD) δ 2.42 (br s, CH2CH2CO2, 12H), 2.73 (br s, CH2CO2, 12H), 7.91, 8.13, 8.51, 9.05 (br, ArH, 10H); 13C NMR (D2O/NaOD) δ 30.7 (CH2CH2CO2), 31.7 (CH2CO2), 60.0 (4°CNH), 171.3, 123.6, 124.6, 128.3, 128.8, 131.2, 143.6, 146.9, 154.9 (CAr), 168.9 (CONH), 182.5 (CO2); ESI-MS m/z 803.66 (M+ + 1). Anal. Calcd for C40H42N4O14: C, 59.85; H, 5.27; N, 6.98. Found: C, 59.77; H, 5.15; N, 6.86.
18-Cascade:2,2'-biquinoline-4,4':(2-oxo-3-azaethylidyne):(3-oxo-2-azapentylidyne):tert-butyl Propanoate (8). A mixture of hexaacid 7 (1 g, 1.24 mmol), DCC (1.85 g, 8.95 mmol), and 1-HBT (1.21 g, 8.95 mmol) in dry DMF (20 mL) was stirred for 2 h at 25 °C. A white precipitate of dicyclohexylurea was noted. Amine 5 (3.72 g, 8.95 mmol) was added and the mixture was stirred at 25 °C for 48 h. After filtration, DMF was removed in vacuo to give a residue, which was dissolved in EtOAc. The organic layer was then sequentially washed with aq. HCl (10%), followed by deionized water, K2CO3 solution, saturated brine, and dried (MgSO4). The solvent was then evaporated in vacuo to give a residue, which was chromatographed (Al2O3) eluting with a mixture of EtOAc/CH2Cl2 (1:1) affording (60%) 8, as a white solid: 2.37 g; mp 81-84 °C; 13C NMR δ 27.9 (CH3), 29.8 (CH2CH2CO2t-Bu), 31.7 (CH2CH2CONH), 57.5, 59.1 (4°CNH), 80.5 (OCMe3), 116.7, 124.9, 125.4, 128.3, 130.2, 130.4, 142.9, 148.3, 155.0 (CAr), 167.5 (ArCONH), 172.4 (CONH), 172.7 (CO2t-Bu); MALDI-TOF MS: m/z 3189; Calcd for C172H276N10O44: 3188.12.
18-Cascade:2,2'-biquinoline-4,4':(2-oxo-3-azaethylidyne):(3-oxo-2-azapentylidyne): propanoic Acid (9). A mixture of ester 8 (90 mg, 28 µmol) and formic acid (5 mL) was stirred at 25 °C for 18 h. Formic acid was removed in vacuo to give a residue, which was dissolved in MeOH then concentrated in vacuo to give (94%) 9, as a white powder: 58 mg; 1H NMR (CD3OD) δ 2.17, 2.44 (br CH2CH2, 96H), 7.95, 8.10, 8.46, 8.51, 9.01 (br, ArH, 10H); 13C NMR (CD3OD) δ 29.4, 30.6 (CH2CH2CO2t-Bu), 32.1 (CH2CH2CONH), 58.8, 60.9 (4°CNH), 118.8, 126.4, 127.0, 129.9, 130.7, 133.1, 146.8, 148.1, 154.9 (CAr), 169.3 (ArCONH), 175.6 (CONH), 177.4 (CO2H); ESI-MS: m/z 2179 (M+ + 1); Calcd for C100H132N10O44: 2178.18.
Generation 1 Cu(I) Complex 10. To a solution of hexaester 6 (100 mg, 87 µmol) in MeCN (5 mL), [Cu(MeCN)4]BF4 (13.6 mg, 43.5 µmol) in MeCN was added dropwise under an N2 atmosphere. A deep purple color was immediately observed due to complex formation. The mixture was stirred for an additional 6 h, then the solvent was removed in vacuo. Fresh MeCN was added and the solution was filtered through a size exclusion column using MeCN to give (100%) the purple Cu(I) complex 10: 113 mg; 1H NMR δ 1.43 (s, CH3, 104H), 2.35, 2.41 (br CH2CH2, 48H), 7.40 (t, ArH, 4H), 7.55 (t, ArH and NH, 8H), 7.72 (d, ArH, 4H), 8.30 (d, ArH, 4H), 8.95 (s, ArH, 4H); 13C NMR δ 27.9 (CH3), 29.9 (CH2CH2), 59.4 (4°CNH), 80.5 (OCMe3), 117.7, 125.7, 126.5, 128.0, 129.6, 131.8, 144.4, 145.9, 151.3 (CAr), 166.0 (CONH), 172.7 (CO2t-Bu); λmax (MeCN): 540 nm (ε = 2800); ESI-MS m/z 2341.8 (M+ - BF4); Calcd for C128H180BCuF4N8O28 (2429.22).
Generation 2 Cu(I) Complex 11. A solution of [Cu(MeCN)4]BF4 (5 mg, 15 µmol) in MeCN was added to a stirred solution of free ligand 8 (100 mg, 30 µmol) in MeCN under N2 atmosphere. A deep purple color due to complex formation was immediately observed. After six hours, the solvent was removed in vacuo and fresh MeCN was added. The resultant solution was filtered through a size exclusion column using MeCN to give (100%) 11, as a purple solid: 101 mg; 1H NMR δ 1.42 (s, CH3, 324H), 2.2 - 2.4 (br CH2CH2, 216H), 7.4 – 9.0 (br, ArH & NH, 40H); 13C NMR δ 27.8 (CH3), 29.9 (CH2CH2), 59.4, 57.6 (4°CNH), 80.5 (OCMe3), 117.6, 125.8, 126.5, 128.0, 129.5, 131.8, 144.3, 145.9, 151.3 (CAr), 166.0, 172.4(CONH), 172.7 (CO2t-Bu); λmax (MeCN): 540 nm (ε = 2200); MALDI-TOF MS: 6439 (M-BF4); Calcd. C344H552BCuF4N20O88 (6526.59).
ACKNOWLEDGMENTS
We thank the National Science Foundation (Grant DMR-0705015 and DMR-0812337) for continued support and the Ohio Board of Regents.
References
1. G. R. Newkome and C. D. Shreiner, Chem. Rev., 2010, 110, 6338. CrossRef
2. G. R. Newkome, B. D. Woosley, E. He, C. N. Moorefield, R. Güther, G. R. Baker, G. H. Escamilla, J. Merrill, and H. Luftmann, Chem. Commun., 1996, 2737. CrossRef
3. T. Chin, Z. Gao, I. Lelouche, Y. K. Shin, A. Purandare, S. Knapp, and S. S. Isied, J. Am. Chem. Soc., 1997, 119, 12849. CrossRef
4. G. R. Newkome, C. N. Moorefield, R. Güther, and G. R. Baker, Polym. Prepr., 1995, 36, 609.
5. G. R. Newkome, L. A. Godínez, and C. N. Moorefield, Chem. Commun., 1998, 1821. CrossRef
6. D. Astruc and F. Chardac, Chem. Rev., 2001, 101, 2991. CrossRef
7. D. Astruc, K. Heuzé, S. Gatard, D. Méry, S. Nlate, and L. Plault, Adv. Synth. Catal., 2005, 347, 329. CrossRef
8. D. Méry and D. Astruc, Coord. Chem. Rev., 2006, 250, 1965.
9. T. Le Bouder, O. Maury, A. Bondon, K. Costuas, E. Amouyal, I. Ledoux, J. Zyss, and H. Le Bozec, J. Am. Chem. Soc., 2003, 125, 12284. CrossRef
10. Y.-R. Hong and C. B. Gorman, J. Org. Chem., 2003, 68, 9019. CrossRef
11. J. B. Livramento, É. Tóth, A. Sour, A. Borel, A. E. Merbach, and R. Ruloff, Angew. Chem. Int. Ed., 2005, 44, 1480. CrossRef
12. M. V. Kulikova, N. McClenaghan, and K. P. Balashev, Russ. J. Gen. Chem., 2005, 75, 665. CrossRef
13. Y.-R. Hong and C. B. Gorman, Chem. Commun., 2007, 3195. CrossRef
14. F. Grimm, K. Hartnagel, F. Wessendorf, and A. Hirsch, Chem. Commun., 2009, 1331. CrossRef
15. A. Sánchez-Méndez, E. de Jesús, J. C. Flores, and P. Gómez-Sal, Eur. J. Inorg. Chem., 2010, 141.
16. R. Ziessel, G. Pickaert, F. Camerel, B. Donnio, D. Guillon, M. Cesario, and T. Prangé, J. Am. Chem. Soc., 2004, 126, 12403. CrossRef
17. U. Hahn, F. Vögtle, G. De Pauli, M. Staffilini, and L. De Cola, Eur. J. Inorg. Chem., 2009, 2639. CrossRef
18. M. Kawa and T. Takahagi, Chem. Mater., 2004, 16, 2282. CrossRef
19. C. Pitois, A. Hult, and M. Lindgren, J. Lumin., 2005, 111, 265. CrossRef
20. S. Frein, M. Auzias, A. Sondenecker, L. Vieille-Petit, B. Guintchin, N. Maringa, G. Süss-Fink, J. Barbará, and R. Deschenaux, Chem. Mater., 2008, 20, 1340. CrossRef
21. F. P. Hubbard, Jr., G. Santonicola, E. W. Kaler, and N. L. Abbott, Langmuir, 2006, 21, 6131. CrossRef
22. M. J. Overett, R. Meijboom, and J. R. Moss, Dalton Trans., 2005, 551. CrossRef
23. H. Aït-Haddou, S. M. Leder, and M. R. Gagné, Inorg. Chim. Acta, 2004, 357, 3854.
24. R. Andrés, E. de Jesús, F. J. de la Mata, J. C. Flores, and R. Gómes, J. Organomet. Chem., 2005, 690, 939.
25. L. Shen, F. Li, Y. Sha, X. Hong, and C. Huang, Tetrahedron Lett., 2004, 45, 3961. CrossRef
26. N. Nishiyama, H. R. Stapert, G.-D. Zhang, D. Takasu, D.-L. Jiang, T. Nagano, T. Aida, and K. Kataoka, Bioconj. Chem., 2003, 14, 58. CrossRef
27. S. Li, W. Zhu, Z. Xu, J. Pan, and H. Tian, Tetrahedron, 2006, 62, 5035. CrossRef
28. S.-C. Lo, N. A. H. Male, J. P. J. Markham, S. W. Magennis, P. L. Burn, O. V. Salata, and I. D. W. Samuel, Adv. Mater., 2002, 14, 975.
29. S.-C. Lo, E. B. Namdas, P. L. Burn, and I. D. W. Samuel, Macromolecules, 2003, 36, 9721. CrossRef
30. N. Cumpstey, R. N. Bera, P. L. Burn, and I. D. W. Samuel, Macromolecules, 2005, 38, 9564. CrossRef
31. R. N. Bera, N. Cumpstey, P. L. Burn, and I. D. W. Samuel, Adv. Funct. Mater., 2007, 17, 1149. CrossRef
32. S.-C. Lo, G. J. Richards, J. P. J. Markham, E. B. Namdas, S. Sharma, P. L. Burn, and I. D. W. Samuel, Adv. Funct. Mater., 2005, 15, 1451. CrossRef
33. W.-Y. Wong, G.-J. Zhou, X.-M. Yu, H.-S. Knok, and Z. Lin, Adv. Funct. Mater., 2007, 17, 315. CrossRef
34. Q.-D. Liu, J. Lu, J. Ding, and Y. Tao, Macromol. Chem. Phys., 2008, 209, 1931. CrossRef
35. S. Li, C. N. Moorefield, C. D. Shreiner, P. Wang, R. Sarkar, J. L. Chavez, and G. R. Newkome, New J. Chem. 2011, DOI: 10.1039/c1nj20195f. CrossRef
36. C. Huang, C.-G. Zhen, S. P. Su, Z.-K. Chen, X. Liu, D.-C. Zou, Y.-R. Shi, and K. P. Loh, J. Organomet. Chem., 2009, 694, 1317. CrossRef
37. S. Gambino, S. G. Stevenson, K. A. Knights, P. L. Burn, and I. D. W. Samuel, Adv. Funct. Mater., 2009, 19, 317. CrossRef
38. B. Blom, M. J. Overett, R. Meijboom, and J. R. Moss, Inorg. Chim. Acta, 2005, 358, 3491. CrossRef
39. G. Bergamini, C. Saudan, P. Ceroni, M. Maestri, V. Balzani, M. Gorka, S.-K. Lee, J. van Heyst, and F. Vögtle, J. Am. Chem. Soc., 2004, 126, 16466. CrossRef
40. J. Ding, J. Lü, Y. Cheng, Z. Xie, L. Wang, X. Jing, and F. Wang, J. Organomet. Chem., 2009, 694, 2700. CrossRef
41. J. Ding, B. Wang, Z. Yue, B. Yao, Z. Xie, Y. Cheng, L. Wang, X. Jing, and F. Wang, Angew. Chem. Int. Ed., 2009, 48, 6664. CrossRef
42. E. C. Constable, C. E. Housecroft, M. Neuburger, S. Schaffner, and L. J. Scherer, Dalton Trans., 2004, 2635. CrossRef
43. S.-H. Hwang, K. S. Yoo, C. N. Moorefield, and G. R. Newkome, J. Polym. Sci., Part B: Polym. Phys., 2004, 42, 1487. CrossRef
44. D. Joester, V. Gramlich, and F. Diederich, Helv. Chim. Acta, 2004, 87, 2896. CrossRef
45. C. Kim and H. Kim, J. Organomet. Chem., 2003, 673, 77. CrossRef
46. G. R. Newkome, F. Cardullo, E. C. Constable, C. N. Moorefield, and A. M. W. C. Thompson, J. Chem. Soc., Chem. Commun., 1993, 925. CrossRef
47. G. R. Newkome and E. He, J. Mater. Chem., 1997, 7, 1237. CrossRef
48. G. R. Newkome, E. He, and L. A. Godínez, Macromolecules, 1998, 31, 4382. CrossRef
49. G. R. Newkome, E. He, L. A. Godínez, and G. R. Baker, J. Am. Chem. Soc., 2000, 122, 9993. CrossRef
50. G. R. Newkome, K. S. Yoo, and C. N. Moorefield, Chem. Commun., 2002, 2164. CrossRef
51. G. R. Newkome, K. S. Yoo, H. J. Kim, and C. N. Moorefield, Chem. Eur. J., 2003, 9, 3367. CrossRef
52. G. R. Newkome, K. S. Yoo, and C. N. Moorefield, Tetrahedron, 2003, 59, 3955. CrossRef
53. N. B. McKeown, Adv. Mater., 1999, 11, 67. CrossRef
54. D. Astruc, M.-C. Daniel, and J. Ruiz, Topics in Organometalic Chemistry, 2006, 8, 2751.
55. D. Astruc, C. Ornelas, and J. Ruiz, Acc. Chem. Res., 2008, 41, 841. CrossRef
56. D. Astruc, Bull. Chem. Soc. Jpn., 2007, 80, 1658. CrossRef
57. D. Astruc, C. Ornelas, and J. Ruiz, Chem. Eur. J., 2009, 15, 8936. CrossRef
58. D. Astruc, E. Boisselier, and C. Ornelas, Chem. Rev., 2010, 110, 1857. CrossRef
59. Molecular Electronics: Biosensors and Biocomputers, ed. by F. T. Hong, Plenum, New York, 1989.
60. G. R. Desiraju, Acc. Chem. Res., 1996, 29, 441. CrossRef
61. J. Issberner, F. Vögtle, L. De Cola, and V. Balzani, Chem. Eur. J., 1997, 3, 706. CrossRef
62. G. R. Newkome, A. K. Patri, and L. A. Godínez, Chem. Eur. J., 1999, 5, 1445. CrossRef
63. A. I. Vogel, Textbook of Practical Organic Chemistry, Longman, England, U.K., 1994.
64. M. Bodanzsky and A. Bodanzsky, The Practice of Peptide Synthesis, Reactivity and Structure Concepts in Organic Chemistry, Springer Verlag, New York, 1984.
65. G. R. Newkome, R. K. Behera, C. N. Moorefield, and G. R. Baker, J. Org. Chem., 1991, 56, 7162. CrossRef
66. G. R. Newkome and C. D. Weis, Org. Prep. Proced. Int., 1996, 28, 485.