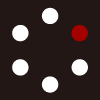
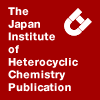
HETEROCYCLES
An International Journal for Reviews and Communications in Heterocyclic ChemistryWeb Edition ISSN: 1881-0942
Published online by The Japan Institute of Heterocyclic Chemistry
e-Journal
Full Text HTML
Received, 3rd August, 2011, Accepted, 6th September, 2011, Published online, 9th September, 2011.
DOI: 10.3987/COM-11-S(P)94
■ Regioselective Glycosylation of Unprotected Methyl Hexopyranoside by Transient Masking with Arylboronic Acid
Takashi Nishino, Yohei Ohya, Rie Murai, Tatsuya Shirahata, Daisuke Yamamoto, Kazuishi Makino, and Eisuke Kaji*
School of Pharmacy, Kitasato University, Shirokane 5-9-1, Minato-ku, Tokyo 108-8641, Japan
Abstract
Unprotected methyl α/β-D-galactopyranoside, α/β-D-glucopyranoside, α-L-fucopyranoside, and α-L-rhamnopyranoside were regioselectively glycosylated by treatment with per-O-pivaloyl-α-D-glycopyranosyl bromide to give glycosyl-β(1→3)-galactopyranoside, glycosyl- β(1→2/3)-glucopyranoside, glycosyl-β(1→2)-fucopyranoside, and glycosyl- β(1→4)-rhamnopyranoside, respectively. The reaction mainly occurred at the secondary hydroxy group, even in the presence of a primary hydroxy group, which was masked with arylboronic acid.INTRODUCTION
Oligosaccharides are important target molecules in chemical and biological research. To ensure sufficient quantities of oligosaccharides available for studying their biological functions, suitable synthetic methods are required, especially when only limited quantities can be isolated from natural sources.1 At present, many methods based on a conventional protection/deprotection strategy are available for synthesizing oligosaccharides,2 but such methods typically require several steps for extensive manipulation of protecting groups, leading to a lengthy and impractical synthetic scheme. Regioselective glycosylation is a promising approach to overcoming these drawbacks because the reaction should be much more selective, rapid, and productive.
Although regioselective protection of hydroxy-free sugars has been frequently reported,3 there are few precedents for regioselective glycosylation; in particular, stannylene-mediated4-7 or boronate-mediated8 regioselective glycosylation of unprotected hexopyranoside has been reported for one-pot assembly of certain oligosaccharides. However, these methods are limited to producing α/β-(1→6)-linked disaccharides, except for 6-deoxyhexosides; in other words, glycosylation of the primary hydroxy group of a hexose is preferred. Furthermore, the yield of the glycosylation reaction is not always high.4-8 Accordingly, a novel regioselective glycosylation method is needed that can be applied to secondary hydroxy groups, even in the presence of a primary hydroxy group.
A potential method for regio- and stereo-selective glycosylation of fully unprotected methyl hexopyranoside is in situ masking hydroxy groups with a suitable arylboronic acid.9 Promoted by Ag(I) on silica alumina, glycosylation of unprotected methyl hexopyranoside by treatment with glycosyl bromide in a one-pot procedure is shown in this study to yield β-(1→3)- or β-(1→2)-linked disaccharides in good to moderate yields.
RESULTS AND DISCUSSION
We focused on arylboronic acids, which have been utilized in molecular recognition of sugar hydroxy groups since they can distinguish cis-1,2-diols and 1,3-diols to form five- and six-membered boronates, respectively. The preferred positions for boronate formation in various hexopyranosides are shown in Figure 1.
Accordingly, our synthetic plan for regioselective glycosylation should proceed via intermediary boronate formation, followed by glycosylation and aqueous workup to provide a disaccharide through a one-pot procedure (Scheme 1). The regioselectivity should be controlled by boronate formation, in which the boron atom reduces the nucleophilicity of the neighboring oxygen atom; hence glycosylation will occur at only the free hydroxy group in the acceptor molecule to afford (1→3)- or (1→2)-linked disaccharide. This selectivity would compliment the stannylene acetal method, which mainly provides (1→6)-linked disaccharides.
Since glycosyl β-(1→3)-linked D-galactopyranosides are the core disaccharide units of many immunologically relevant oligosaccharides,10 we first examined our method by using unprotected methyl β-D-galactopyranoside (1) as the glycosyl acceptor. For β-selective glycosylation, we chose per-O-pivaloyl-α-D-glucopyranosyl bromide (2) as the glycosyl donor, because the stereoelectronic effects of the 2-O-pivaloyl group are suited to β selectivity. In addition, this donor hardly forms an orthoester under the glycosylation reaction conditions.11
As in the stannylene-activation method for regioselective glycosylation of methyl β-D-galactopyranoside (1) with per-O-benzoylated glycopyranosyl bromide, we selected Ag(I) on silica alumina12 as a promoter, and screened the solvent, temperature, arylboronic acid substituent, and other reaction conditions.
We first examined solvent effects in a two-stage one-pot procedure where the boronate intermediate was prepared in the first solvent (solvent 1), solvent 1 was removed, and the donor in a second solvent (solvent 2) was added, yielding disaccharides as shown in Scheme 2. Solvent effects are summarized in Table 1.
NMR spectra were consistent with the generation of the boronate intermediate (cf. Experimental). We found that 4,6-boronate 4a formed by refluxing 1 (1 equiv) and 3a (2 equiv) in MeOH for 3 h. With MeOH as solvent 1 and 1,2-dichloroethene (DCE) as solvent 2, β-(1→3)-glycoside 5 was obtained in 37% yield along with β-(1→2)- and β-(1→6)-linked disaccharides in 6% and 3% yields, respectively (Run 1). When THF (Run 2) or MeCN (Run 3) was used as solvent 2, regioselectivity was higher but the yield of 5 was lower in comparison with Run 1. Using dichloromethane (DCM) as solvent 1 and DCE as solvent 2 improved the yield of 5, but the selectivity became lower (Runs 4 and 5). Without boronic acid, the reaction became less effective, giving mainly β-(1→6)-linked disaccharide in only 13% yield (Run 6).
On the basis of the above results, we changed the procedure into a one-stage one-pot procedure, where the all reagents (donor, acceptor, and boronic acid) were mixed simultaneously in DCE and stirred at room temperature for 16 h, followed by addition of the promoter (Ag (I) on silica alumina) and stirring at 50 °C for 24 h (Scheme 3). Compared with the results shown in Table 1 (two-stage one-pot), the yields of disaccharides through the one-stage procedure were substantially improved. The results are listed in Table 2.
In the one-stage one-pot procedure, the effects on the regioselective glycosylation of the arylboronic acid para substituent were investigated. All the substituents tested other than the NO2 group (Run 3) resulted in increased reaction yields up to 70% (Runs 1–5), and the OMe group gave the best yield (Run 1). However, regioselectivity remained approximately 3.5 to 1 in favor of the (1→3) linkage over the (1→2) linkage.
Then effects of reaction temperature on disaccharide yield as well as on regioselectivity were examined. The results are summarized in Table 3.
Temperatures between −20 to 50 °C were tested. The reaction conditions of 0 °C for 24 h provided the best yield (78%) of the disaccharides with good regioselectivity ((1→3)/(1→2) = 12/1). Subsequently, these conditions were extended to other glycosyl donors such as 2,3,4,6-tetra-O-pivaloyl-α-D- galactopyranosyl bromide (7) and 2,3,4,6-tetra-O-pivaloyl-α-D-mannopyranosyl bromide (8) (Scheme 4).
Glycosylation of galactoside 1 by treatment with galactosyl bromide 7 in the presence of boronic acid 3a provided galactosyl-β-(1→3)-galactoside 9 and galactosyl-β-(1→2)-galactoside 10 in 62% and 5% yields, respectively, similar to the results for glucosyl donor 2. Regioselectivity in this case was estimated to about 12/1. The structures of 9 and 10 were determined from their NMR spectra: the β-configuration was determined from a coupling constant, J1’,2’ = 8.0 Hz, and glycosylated carbons of 9 and 10 were identified from 13C-NMR chemical shifts at δ 81.34 (C-3) and δ 80.24 (C-2), respectively. Furthermore, a second order H/C-NMR spectra, i.e., 1H-Detected Multiple-bond Heteronuclear Bond Coorelation (HMBC) showed a distinct coupling signal (3JC,H) between H-1’ and C-3 of the compound 9, which clearly provides the structure of (1→3)-linked disaccharide. On the other hand, HMBC spectra of 10 showed a coupling signal (3JC,H) between H-1’ and C-2 designating a (1→2)-linked disaccharide.
In contrast, the mannosyl donor 8 gave low yield and poor regioselectivity: mannosyl-α-(1→3)-galactoside 11 and mannosyl-α-(1→2)-galactoside 12 were obtained in only 6% and 8% yields, respectively. The α-D-manno configuration was determined by JC1-H1 value (11: 171.0 Hz, 12: 174.7 Hz) and the intersaccharide linkages were found to be similar to those in the galactosyl case described in the experimental section.
Finally, our method was applied to various unprotected acceptors (Table 4). Glycosylation of methyl α-D-galactopyranoside (13) afforded similar results as β-D-galactopyranoside (1) with moderate yield and regioselectivity (Run 2). Methyl α- and β-D-glucopyranosides had different selectivity: the methyl β-D-glucoside (16) gave low yield and poor regioselectivity (Run 3) . On the other hand, use of methyl α-D-glucopyranoside (19) resulted in a slightly better yield with reversed regioselectivity such that the β-(1→2)-disaccharide was favored over the β-(1→3)-disaccharide by about 6 to 1 (Run 4).
The regioselectivity appeared to arise from stereochemical factors. The good β-(1→3) selectivity of galactopyranosides (1 and 13) might attributable to the relative openness around the C-3 hydroxy group compared with the C-2 hydroxy group; this openness would allow for easier glycosylation. In the β-glucoside (16), there would be little difference in the stereochemical space around the C-3 and C-2 hydroxy groups, whereas in α-glucoside 19 the donor molecule might be accessible from the β-side of the C-2 hydroxy group, owing to the wider space in comparison with the C-3 hydroxy group, such that β-(1→2)-glucoside 20 is preferred (cf. Figure 2).
6-Deoxyhexoside (e.g., methyl α-L-fucopyranoside (22) and methyl α-L-rhamnopyranoside (24)), afforded a single product since the intermediary boronate might mask the 1,2-cis-hydroxy group and leave only one hydroxyl group free, which could be regiospecifically glycosylated. In fact, glucosyl-β-(1→2)-fucopyranoside (23) and glucosyl-β-(1→4)-rhamnopyranoside (25) were obtained in 44% and 61% yields, respectively. The structures of the disaccharides were determined by NMR spectroscopy, mainly from the glycosylation shift of the glycosylated carbon in 13C-NMR and HMBC spectra.
In summary, when promoted by Ag(I) on silica alumina, glycosylation of unprotected methyl β-D-galactopyranoside with per-O-pivaloyl-α-D-glucopyranosyl bromide in the presence of 4-methoxyphenyl boronic acid was found to give mainly β-(1→3)-linked disaccharide in 72% yield in a one-pot reaction. This regioselectivity was rationalized by generation of a 4,6-boronate intermediate, which might be formed in situ through the reaction between 4,6-hydroxy groups of methyl β-D-galactopyranoside and arylboronic acid. Thus, the intermediary boronate functions as a transient masking group present only in the reaction medium. Several unprotected sugars were regioselectively glycosylated at a secondary hydroxy group, even in the presence of primary hydroxy group, through this one-pot procedure. This method might be an alternative of the conventional protection/deprotection strategies. Further development and improvement of this methodology are in progress.
EXPERIMENTAL
General. Physical and spectral data were recorded on the following instruments; Mp: Yamato MP-1 apparatus and Yanagimoto micro melting-point apparatus (uncorrected); [α]D: JASCO DIP-150 digital polarimeter; MS: JEOL JMS-AX505HA and JMS-AX700 MStation mass spectrometer; 1H and 13C NMR: Varian VXR-300 and XL-400 spectrometers. TLC was carried out on silica-gel 60 F254 (Merk Art. 5735) developed with the same solvent systems as used for column chromatography in the individual experimental section. The spots were made visible by UV light (254 nm) or by charring with 10% aqueous H2SO4. Column chromatography was achieved on silica-gel 60 (Merck Art. 7734). Glycosyl acceptors employed were purchased from Tokyo Kasei Co., Wako Co., or Aldrich Co. Glycosyl donor 211 was prepared according to the reported method. NMR data of boronate-masked methyl β-D-galactopyranoside 4a was shown in this section. In general, chemical shifts of methyl group of the methoxy and pivaloyl group for methyl 2,3,4,6-tetra-O-pivaloylglycosides are as follows. OCH3 : 1Hδ = ca. 3.5 ; 13C δ = ca. 57; Pivaloyl (CH3)3CCO : 1H δ = 1.1-1.3; 13C δ = 26.9-27.2; (CH3)3CCO 13C δ =38.6-39.2; (CH3)3CCO 13C δ =176-179.
General procedure for boronate-mediated glycosylation using glycosyl bromide donor: A mixture of unprotected methyl hexopyranoside (an acceptor, 0.25 mmol), arylboronic acid (0.25 mmol), per-O-pivaloyl-α-D-glycosyl bromide (0.50 mmol), and MS-3A (powder, 500 mg) in anhydrous Cl(CH2)2Cl (5.0 mL) was stirred at room temperature for 16 h. To a resulting suspension including the boronate was added Ag (I)-silica alumina12 (900 mg) in one portion. The mixture was stirred in the dark at 0 °C for 24 h. After dilution with Cl(CH2)2Cl (30 mL), the mixture was filtered through Celite, and the filtrate was washed with 5% NaHCO3 aq (30 mL) and water (3×30 mL), dried (Na2SO4), and evaporated. The residue was purified by silica-gel column chromatography (CHCl3 : MeOH, 20:1) to give the disaccharide (products and yields: see Tables).
1,2,3,4,6-Penta-O-pivaloyl-β-D-galactopyranose: A mixture of D-galactose (21.8 g, 0.12 mol), pivaloyl chloride (91.0 g, 0.75 mol), pyridine (90 mL), and CHCl3 (150 mL) was stirred under reflux for 4 days. The reaction mixture was evaporated in vacuo and the residue was dissolved in Et2O (300 mL), washed with 1 M H2SO4 (50 mL), 5% NaHCO3 aq (5 x 250 mL), and water (2 x 250 mL). The organic phase was dried (Na2SO4) and evaporated to give the residue, which was crystallized from EtOH to afford 53.1 g (74% yield) of the title compound as colorless crystals. Mp 123~125 °C. [α]D26.7 +8.12 (c 1.00, CHCl3). MS (FAB) m/z: 623 [M+Na]+. Anal. Calcd for C31H52O11:C 61.98, H 8.72. Found: C 61.79, H 8.69. 1H-NMR (300 MHz, CDCl3) δ: 3.99 (1H, dd, J5,6a = 6.5, J6a,6b = 10.0 Hz, H-6a), 4.09 (1H, ddd, J4,5 = 1.0, J5,6a = 6.5, J5,6b = 6.5, H-5), 4.17 (1H, dd, J5,6b = 6.5, J6a,6b = 10.0 Hz, H-6b), 5.17 (1H, dd, J2,3 = 10.0, J3,4 = 3.5 Hz, H-3), 5.43 (1H, dd, J3,4 = 3.5, J4,5 = 1.0 Hz, H-4), 5.58 (1H, dd, J1,2 = 8.0, J2,3 = 10.0 Hz, H-2), 5.72 (1H, d, J1,2 = 8.0 Hz, H-1). 13C-NMR (75 MHz, CDCl3) δ: 60.70 (C-6), 66.48 (C-4), 67.70 (C-2), 71.07 (C-3), 71.78 (C-5), 92.13 (C-1).
1,2,3,4,6-Penta-O-pivaloyl-α-D-mannopyranose: Prepared similarly as described above for per-O- pivaloyl-β-D-galactopyranose. Isolation of the anomeric isomers was performed by recrystallization from ethanol.
White powder (Yield 16%). Mp 154~161 °C. [α]D29.1 +40.7 (c 1.00, CHCl3). MS (FAB) m/z: 623 [M+Na]+. Anal. Calcd for C31H52O11: C 61.98, H 8.72. Found: C 61.95, H 8.67. 1H-NMR (300 MHz, CDCl3) δ: 4.03 (1H, ddd, J4,5 = 11.0, J5,6a = 2.0, J5,6b = 4.0 Hz, H-5), 4.12 (1H, dd, J5,6a = 2.0, J6a,6b = 12.0 Hz, H-6a), 4.18 (1H, dd, J5,6b = 4.0, J6a,6b = 12.0 Hz, H-6b), 5.28 (1H, dd, J1,2 = 2.0, J2,3 = 3.5 Hz, H-2), 5.38 (1H, dd, J2,3 = 3.5, J3,4 = 11.0 Hz, H-3), 5.54 (1H, t, J3,4 = J4,5 = 11.0 Hz, H-4), 6.01 (1H, d, J1,2 = 2.0 Hz, H-1). 13C-NMR (75 MHz, CDCl3) δ: 61.70 (C-6), 64.51 (C-4), 68.21 (C-2), 69.36 (C-3), 71.17 (C-5), 90.72 (JC-1,H-1 = 176.0 Hz, C-1).
1,2,3,4,6-Penta-O-pivaloyl-β-D-mannopyranose:
White powder (Yield 71%). Mp 125~127 °C. [α]D28.5 –19.2 (c 1.00, CHCl3). MS (FAB) m/z: 623 [M+Na]+. Anal. Calcd for C31H52O11: C 61.98, H 8.72. Found: C 61.86, H 8.80. 1H-NMR (300 MHz, CDCl3) δ: 3.84 (1H, ddd, J4,5 = 10.0, J5,6a = 2.0, J5,6b = 4.0 Hz, H-5), 4.16 (1H, dd, J5,6a = 2.0, J6a,6b = 12.0 Hz, H-6a), 4.21 (1H, dd, J5,6b = 4.0, J6a,6b = 12.0 Hz, H-6b), 5.16 (1H, dd, J2,3 = 3.5, J3,4 = 10.0 Hz, H-3), 5.46 (1H, dd, J1,2 = 1.0, J2,3 = 3.5 Hz, H-2), 5.47 (1H, t, J3,4 = J4,5 = 10.0 Hz, H-4), 5.83 (1H, d, J1,2 = 1.0 Hz, H-1). 13C-NMR (75 MHz, CDCl3) δ: 61.49 (C-6), 64.69 (C-4), 68.13 (C-2), 70.98 (C-3), 73.15 (C-5), 90.67 (JC-1,H-1 = 160.9 Hz, C-1).
Methyl β-D-galactopyranoside 4,6-(p-methoxyphenyl)boronic ester (4a): Although the intermediary boronates were not isolated under one-pot glycosylation, generation of the boronic ester (4a) was identified by its NMR spectra.
White amorphous powder. HRMS (FAB) m/z: Calcd for C14H19BO7Na [M+Na]+ 333.1116; found 333.1108. 1H-NMR (400MHz, CD3OD) δ: 3.49 (1H, dd, J1,2 = 7.5, J2,3 = 9.5 Hz, H-2), 3.64 (1H, dd, J2,3 = 9.5, J3,4 = 3.5 Hz, H-3), 3.92 (1H, ddd, J4,5 = 1.0, J5,6a = 2.0, J5,6b = 1.5 Hz, H-5), 4.16 (1H, dd, J5,6a = 2.0, J6a,6b = 12.0 Hz, H-6a), 4.24 (1H, d, J1,2 = 7.5, H-1), 4.26 (1H, dd, J5,6b = 1.5, J6a,6b = 12.0 Hz, H-6b), 4.34 (1H, dd, J3,4 = 3.5, J4,5 = 1.0 Hz, H-4). 13C-NMR (100MHz, CD3OD) δ: 65.73 (C-6), 69.92 (C-5), 71.87 (C-2), 72.12 (C-4), 74.25 (C-3), 105.68 (C-1).
Methyl O-2,3,4,6-tetra-O-pivaloyl-β-D-glucopyranosyl-(1→3)-β-D-galactopyranoside (5):
Colorless syrup. [α]D26.0 +2.44 (c 1.00, CHCl3). HRMS (FAB) m/z: Calcd for C33H56O15Na [M+Na]+ 715.3511; found 715.3550. 1H-NMR (400 MHz, CDCl3) δ: 3.53 (1H, ddd, J4,5 = 1.0, J5,6a = 5.0, J5,6b = 6.5 Hz, H-5), 3.60 (1H, dd, J2,3 = 9.5, J3,4 = 3.5 Hz, H-3), 3.74 (1H, dd, J1,2 = 7.5, J2,3 = 9.5 Hz, H-2), 3.74 (1H, ddd, J4’,5’ = 10.0, J5’,6’a = 2.0, J5’,6’b = 5.5 Hz, H-5’), 3.82 (1H, dd, J5,6a = 5.0, J6a,6b = 12.0 Hz, H-6a), 3.96 (1H, dd, J5’,6’b = 5.5, J6’a,6’b = 12.5 Hz, H-6’b), 3.98 (1H, dd, J5,6b = 6.5, J6a,6b = 12.0 Hz, H-6b), 4.02 (1H, dd, J3,4 = 3.5, J4,5 = 1.0 Hz, H-4), 4.16 (1H, d, J1,2 = 7.5 Hz, H-1), 4.33 (1H, dd, J5’,6’a = 2.0, J6’a,6’b = 12.5 Hz, H-6’a), 4.90 (1H, d, J1’,2’ = 8.0 Hz, H-1’), 5.04 (1H, dd, J1’,2’ = 8.0, J2’,3’ = 9.5 Hz, H-2’), 5.11 (1H, dd, J3’,4’ = 9.5, J4’,5’ = 10.0 Hz, H-4’), 5.35 (1H, t, J2’,3’ = J3’,4’ = 9.5 Hz, H-3’). 13C-NMR (100 MHz, CDCl3) δ: 61.64 (C-6’), 62.38 (C-6), 67.79 (C-4’), 68.65 (C-4), 70.73 (C-2), 71.42 (C-2’), 71.68 (C-3’), 72.49 (C-5’), 74.10 (C-5), 81.10 (C-3), 101.01 (C-1’), 103.73 (C-1).
Methyl O-2,3,4,6-tetra-O-pivaloyl-β-D-glucopyranosyl-(1→2)-β-D-galactopyranoside (6):
Colorless syrup. [α]D27.5 +6.65 (c 1.00, CHCl3). HRMS (FAB) m/z: Calcd for C33H56O15Na [M+Na]+ 715.3511; found 715.3535. 1H-NMR (400 MHz, CDCl3) δ: 3.52 (1H, ddd, J4,5 = 1.5, J5,6a = 4.5, J5,6b = 6.0 Hz, H-5), 3.60 (1H, dd, J1,2 = 7.0, J2,3 = 9.0 Hz, H-2), 3.63 (1H, dd, J2,3 = 9.0, J3,4 = 3.0 Hz, H-3), 3.74 (1H, ddd, J4’,5’ = 10.0, J5’,6’a = 2.0, J5’,6’b =3.5 Hz, H-5’), 3.85 (1H, dd, J5’,6’a = 2.0, J6’a,6’b = 12.5 Hz, H-6a), 3.96 (1H, dd, J5,6b = 6.0, J6a,6b = 12.0 Hz, H-6b), 3.98 (1H, dd, J3,4 = 3.0, J4,5 = 1.5 Hz, H-4), 4.10 (1H, dd, J5’,6’b =3.5, J6’a,6’b = 12.5 Hz, H-6’b), 4.25 (1H, dd, J5’,6’a = 2.0, J6’a,6’b = 12.5 Hz, H-6’a), 4.31 (1H, d, J1,2 = 7.0 Hz, H-1), 4.90 (1H, d, J1’,2’ = 8.0 Hz, H-1’), 5.00 (1H, dd, J1’,2’ = 8.0, J2’,3’ = 9.5 Hz, H-2’), 5.18 (1H, dd, J3’,4’ = 9.5, J4’,5’ = 10.0 Hz, H-4’), 5.35 (1H, t, J2’,3’ = J3’,4’ = 9.5 Hz, H-3’). 13C-NMR (100 MHz, CDCl3) δ: 61.58 (C-6’), 62.61 (C-6), 67.48 (C-4’), 69.14 (C-4), 72.14 (C-3’), 72.17 (C-5’), 72.56 (C-2’), 72.59 (C-3), 73.90 (C-5), 79.70 (C-2), 101.08 (C-1’), 103.04 (C-1).
2,3,4,6-Tetra-O-pivaloyl-α-D-galactopyranosyl bromide (7): To a stirred solution of penta-O- pivaloyl-β-D-galactopyranose (13.5 g, 22.5 mmol) was added dropwise 30% hydrogen bromide in AcOH (25 mL) under ice-cooling. The resulting mixture was stirred at ambient temperature for 1 day, and then evaporated in vacuo azeotropically with toluene (3 x 250 mL). The residue was dissolved in Et2O (300 mL) followed by washing with 5% NaHCO3 aq (250 mL) and water (2 x 250 mL). The organic phase was dried (Na2SO4) and evaporated to give the residue, which was crystallized from Et2O-hexane to afford 10.3 g (78% yield) of 7 as colorless crystals. Mp 56~58 °C. [α]D24.2 +143.6 (c 1.00, CHCl3). MS (FAB) m/z: 579, 581 [M+H]+, 601, 603 [M+Na]+. Anal. Calcd for C26H43O9Br: C 53.89, H 7.48, Br 13.79. Found: C 53.80, H 7.43, Br 13.70. 1H-NMR (300 MHz, CDCl3) δ: 4.06 (1H, dd, J5,6a = 7.0, J6a,6b = 11.0 Hz, H-6a), 4.14 (1H, dd, J5,6b = 7.0 Hz, J6a,6b = 11.0 Hz, H-6b), 4.52 (1H, br dt, J4,5 = 1.0, J5,6a = J5,6b = 7.0 Hz, H-5), 5.03 (1H, dd, J1,2 = 4.0, J2,3 = 10.5 Hz, H-2), 5.50 (1H, dd, J2,3 = 10.5, J3,4 = 3.0 Hz, H-3), 5.54 (1H, dd, J3,4 = 3.0, J4,5 = 1.0 Hz, H-4), 6.69 (1H, d, J1,2 = 4.0 Hz, H-1). 13C-NMR (75 MHz, CDCl3) δ: 60.51 (C-6), 66.60 (C-2), 67.82 (C-4), 68.10 (C-3), 71.55 (C-5), 88.37 (C-1).
2,3,4,6-Tetra-O-pivaloyl-α-D-mannopyranosyl bromide (8): Prepared similarly as described above for galactosyl bromide 7.
Colorless crystals. Mp 113~115 °C. [α]D23.4 +75.9 (c 1.00, CHCl3). MS (FAB) m/z: 579, 581 [M+H]+, 601, 603 [M+Na]+. 1H-NMR (300 MHz, CDCl3) δ: 4.15 (1H, dd, J5,6a = 4.0, J6a,6b = 11.0 Hz, H-6a), 4.25 (1H, ddd, J5,6a = J5,6b = 4.0, J6a,6b = 11.0 Hz, H-5), 4.27 (1H, dd, J5,6b = 4.0 Hz, J6a,6b = 11.0 Hz, H-6b), 5.45 (1H, dd, J1,2 = 1.5, J2,3 = 3.5 Hz, H-2), 5.57 (1H, t, J3,4 = J4,5 = 10.0 Hz, H-4), 5.75 (1H, dd, J2,3 = 3.5, J3,4 = 10.0 Hz, H-3), 6.26 (1H, d, J1,2 = 1.5 Hz, H-1). 13C-NMR (75 MHz, CDCl3) δ: 60.95 (C-6), 64.30 (C-4), 68.32 (C-3), 72.06 (C-2), 73.18 (C-5), 83.73 (JC-1,H-1 = 185.1 Hz, C-1).
Methyl O-2,3,4,6-tetra-O-pivaloyl-β-D-galactopyranosyl-(1→3)-β-D-galactopyranoside (9):
Colorless syrup. [α]D22.1 –0.72 (c 1.00, CHCl3). HRMS (FAB) m/z: Calcd for C33H56O15Na [M+Na]+ 715.3511; found 715.3503. 1H-NMR (400 MHz, CDCl3) δ: 3.54 (1H, ddd, J4,5 = 1.0, J5,6a = 4.5, J5,6b = 6.5 Hz, H-5), 3.61 (1H, dd, J2,3 = 10.5, J3,4 = 3.5 Hz, H-3), 3.76 (1H, dd, J1,2 = 8.0, J2,3 = 9.5 Hz, H-2), 3.80 (1H, dd, J5,6a = 4.5, J6a,6b = 11.5 Hz, H-6a), 3.98 (1H, dd, J5,6b = 6.5, J6a,6b = 11.5 Hz, H-6b), 4.01 (1H, ddd, J4’,5’ = 1.0, J5’,6’a = 5.5, J5’,6’b = 6.0 Hz, H-5’), 4.02 (1H, dd, J5’,6’b = 6.0, J6’a,6’b = 9.5 Hz, H-6’b), 4.02 (1H, dd, J3,4 = 3.5, J4,5 = 1.0 Hz, H-4), 4.12 (1H, dd, J5’,6’a = 5.5, J6’a,6’b = 9.5 Hz, H-6’a), 4.16 (1H, d, J1,2 = 8.0 Hz, H-1), 4.88 (1H, d, J1’,2’ = 8.0 Hz, H-1’), 5.14 (1H, dd, J2’,3’ = 10.5, J3’,4’ = 3.5 Hz, H-3’), 5.22 (1H, dd, J1’,2’ = 8.0, J2’,3’ = 10.5 Hz, H-2’), 5.40 (1H, dd, J3’,4’ = 3.5, J4’,5’ = 1.0 Hz, H-4’). 13C-NMR (75 MHz, CDCl3) δ: 61.46 (C-6’), 62.41 (C-6), 66.67 (C-4’), 68.63 (C-4), 68.91 (C-2’), 70.48 (C-3’), 70.63 (C-2), 71.40 (C-5’), 74.12 (C-5), 81.34 (C-3), 101.32 (C-1’), 103.76 (C-1).
Methyl O-2,3,4,6-tetra-O-pivaloyl-β-D-galactopyranosyl-(1→2)-β-D-galactopyranoside (10):
Colorless syrup. [α]D22.1 –4.44 (c 0.50, CHCl3). HRMS (FAB) m/z: Calcd for C33H56O15Na [M+Na]+ 715.3511; found 715.3544. 1H-NMR (400 MHz, CDCl3) δ: 3.52 (1H, ddd, J4,5 = 1.0, J5,6a = 4.5, J5,6b = 6.0 Hz, H-5), 3.59 (1H, dd, J1,2 = 7.5, J2,3 = 9.0 Hz, H-2), 3.68 (1H, dd, J2,3 = 9.0, J3,4 = 3.5 Hz, H-3), 3.84 (1H, dd, J5,6a = 4.5, J6a,6b = 12.0 Hz, H-6a), 3.96 (1H, dd, J5,6b = 6.0, J6a,6b = 12.0 Hz, H-6b), 3.99 (1H, dd, J3,4 = 3.5, J4,5 = 1.0 Hz, H-4), 4.00 (1H, dd, J5’,6’a = 8.5, J6’a,6’b = 15.0 Hz, H-6’a), 4.01 (1H, ddd, J4’,5’ = 1.0, J5’,6’a = 8.5, J5’,6’b = 10.0 Hz, H-5’), 4.21 (1H, dd, J5’,6’b = 10.0, J6’a,6’b = 15.0 Hz, H-6’b), 4.32 (1H, d, J1,2 = 7.5 Hz, H-1), 4.87 (1H, d, J1’,2’ = 7.5 Hz, H-1’), 5.13 (1H, dd, J1’,2’ = 7.5, J2’,3’ = 10.5 Hz, H-2’), 5.18 (1H, dd, J2’,3’ = 10.5, J3’,4’ = 3.0 Hz, H-3’), 5.42 (1H, dd, J3’,4’ = 3.0, J4’,5’ = 1.0 Hz, H-4’). 13C-NMR (75 MHz, CDCl3) δ: 60.68 (C-6’), 62.51 (C-6), 66.21 (C-4’), 68.95 (C-4), 70.39 (C-2’), 70.65 (C-5’), 70.12 (C-3’), 72.28 (C-3), 74.01 (C-5), 80.24 (C-2), 101.55 (C-1’), 103.39 (C-1).
Methyl O-2,3,4,6-tetra-O-pivaloyl-α-D-mannopyranosyl-(1→3)-β-D-galactopyranoside (11):
Colorless syrup. [α]D19.1 +33.4 (c 1.00, CHCl3). HRMS (FAB) m/z: Calcd for C33H56O15Na [M+Na]+ 715.3511; found 715.3517. 1H-NMR (300 MHz, CDCl3) δ: 3.48 (1H, ddd, J4,5 = 1.0, J5,6a = 5.0, J5,6b = 6.0 Hz, H-5), 3.71 (1H, dd, J2,3 = 9.5, J3,4 = 3.0 Hz, H-3), 3.82 (1H, dd, J1,2 = 7.5, J2,3 = 9.5 Hz, H-2), 3.84 (1H, dd, J5,6a = 5.0, J6a,6b = 11.5 Hz, H-6a), 3.94 (1H, dd, J5,6b = 6.0, J6a,6b = 11.5 Hz, H-6b), 4.07 (1H, dd, J3,4 = 3.0, J4,5 = 1.0 Hz, H-4), 4.14 (1H, dd, J5’,6’a = 2.5, J6’a,6’b = 10.5 Hz, H-6’a), 4.15 (1H, dd, J5’,6’b = 2.5, J6’a,6’b = 10.5 Hz, H-6’b), 4.16 (1H, d, J1,2 = 7.5 Hz, H-1), 4.54 (1H, dt, J4’,5’ = 10.0, J5’,6’a = J5’,6’b = 2.5 Hz, H-5’), 4.95 (1H, d, J1’,2’ = 1.5 Hz, H-1’), 5.30 (1H, dd, J1’,2’ = 1.5, J2’,3’ = 3.0 Hz, H-2’), 5.42 (1H, dd, J2’,3’ = 3.0, J3’,4’ = 10.0 Hz, H-3’), 5.52 (1H, t, J3’,4’ = J4’,5’ = 10.0 Hz, H-4’). 13C-NMR (75 MHz, CDCl3) δ: 61.80 (C-6’), 62.07 (C-6), 64.85 (C-4’), 65.98 (C-4), 68.95 (C-5’), 69.56 (C-2, 3’), 69.66 (C-2’), 74.14 (C-5), 77.66 (C-3), 94.87 (JC-1,H-1 = 171.0 Hz, C-1’), 104.56 (C-1).
Methyl O-2,3,4,6-tetra-O-pivaloyl-α-D-mannopyranosyl-(1→2)-β-D-galactopyranoside (12):
Colorless syrup. [α]D22.3 +35.8 (c 0.65, CHCl3). HRMS (FAB) m/z: Calcd for C33H56O15Na [M+Na]+ 715.3511; found 715.3526. 1H-NMR (300 MHz, CDCl3) δ: 3.50 (1H, ddd, J4,5 = 1.0, J5,6a = 4.5, J5,6b = 5.0 Hz, H-5), 3.60 (1H, dd, J2,3 = 10.0, J3,4 = 3.0 Hz, H-3), 3.62 (1H, dd, J1,2 = 7.0, J2,3 = 10.0 Hz, H-2), 3.92 (1H, dd, J5,6a = 4.5, J6a,6b = 12.0 Hz, H-6a), 3.96 (1H, dd, J5,6b = 5.0, J6a,6b = 12.0 Hz, H-6b), 4.03 (1H, dd, J3,4 = 3.0, J4,5 = 1.0 Hz, H-4), 4.13 (1H, dd, J5’,6’a = 2.5, J6’a,6’b = 12.5 Hz, H-6’a), 4.16 (1H, dd, J5’,6’b = 3.0, J6’a,6’b = 12.5 Hz, H-6’b), 4.26 (1H, d, J1,2 = 7.0 Hz, H-1), 4.51 (1H, ddd, J4’,5’ = 10., J5’,6’a = 2.5, J5’,6’b = 3.0 Hz, H-5’), 5.09 (1H, d, J1’,2’ = 2.0 Hz, H-1’), 5.28 (1H, dd, J1’,2’ = 2.0, J2’,3’ = 3.5 Hz, H-2’), 5.39 (1H, dd, J2’,3’ = 3.5, J3’,4’ = 10.5 Hz, H-3’), 5.51 (1H, t, J3’,4’ = J4’,5’ = 10.5 Hz, H-4’). 13C-NMR (75 MHz, CDCl3) δ: 62.14 (C-6’), 62.78 (C-6), 65.16 (C-4’), 68.97 (C-5’), 69.44 (C-2’), 69.50 (C-3’), 70.23 (C-4), 72.39 (C-3), 73.52 (C-5), 78.30 (C-2), 98.31 (JC-1,H-1 = 174.7 Hz, C-1’), 104.33 (C-1).
Methyl O-2,3,4,6-tetra-O-pivaloyl-β-D-glucopyranosyl-(1→2)-α-D-galactopyranoside (14):
Colorless syrup. [α]D21.5 +54.9 (c 0.84, CHCl3). HRMS (FAB) m/z: Calcd for C33H56O15Na [M+Na]+ 715.3511; found 715.3500. 1H-NMR (300 MHz, CDCl3) δ: 3.74 (1H, ddd, J4’,5’ = 10.0, J5’,6’a = 1.5, J5’,6’b = 5.5 Hz, H-5’), 3.82 (1H, ddd, J4,5 = 1.5, J5,6a = 4.0, J5,6b = 6.0 Hz, H-5), 3.83 (1H, dd, J5,6a = 4.0, J6a,6b = 12.5 Hz, H-6a), 3.88 (1H, dd, J1,2 = 3.5, J2,3 = 9.5 Hz, H-2), 3.92 (1H, dd, J5,6b = 6.0, J6a,6b = 12.5 Hz, H-6b), 3.99 (1H, dd, J2,3 = 9.5, J3,4 = 3.5 Hz, H-3), 4.01 (1H, dd, J5’,6’b = 5.5, J6’a,6’b = 12.0 Hz, H-6’b), 4.09 (1H, dd, J3,4 = 3.5, J4,5 = 1.5 Hz, H-4), 4.28 (1H, dd, J5’,6’a = 1.5, J6’a,6’b = 12.0 Hz, H-6’a), 4.83 (1H, d, J1’,2’ = 8.0 Hz, H-1’), 4.91 (1H, d, J1,2 = 3.5 Hz, H-1), 5.09 (1H, dd, J1’,2’ = 8.0, J2’,3’ = 9.5 Hz, H-2’), 5.12 (1H, dd, J3’,4’ = 9.5, J4’,5’ = 10.0 Hz, H-4’), 5.34 (1H, t, J2’,3’ = J3’,4’ = 9.5 Hz, H-3’). 13C-NMR (75 MHz, CDCl3) δ: 61.78 (C-6’), 63.15 (C-6), 67.58 (C-4’), 67.95 (C-3), 68.93 (C-5), 70.72 (C-4), 71.57 (C-2’), 72.14 (C-3’), 72.55 (C-5’), 78.01 (C-2), 99.44 (C-1), 101.49 (C-1’).
Methyl O-2,3,4,6-tetra-O-pivaloyl-β-D-glucopyranosyl-(1→3)-α-D-galactopyranoside (15):
Colorless syrup. [α]D24.4 +63.7 (c 1.0, CHCl3). HRMS (FAB) m/z: Calcd for C33H56O15Na [M+Na]+ 715.3511; found 715.3523. 1H-NMR (300 MHz, CDCl3) δ: 3.72 (1H, ddd, J4’,5’ = 10.0, J5’,6’a = 2.0, J5’,6’b = 5.5 Hz, H-5’), 3.77 (1H, ddd, J4,5 = 1.0, J5,6a = 4.0, J5,6b = 7.0 Hz, H-5), 3.78 (1H, dd, J5,6a = 4.0, J6a,6b = 12.5 Hz, H-6a), 3.78 (1H, dd, J2,3 = 10.0, J3,4 = 3.5 Hz, H-3), 3.92 (1H, dd, J5,6b = 7.0, J6a,6b = 12.5 Hz, H-6b), 3.94 (1H, dd, J1,2 = 4.0, J2,3 = 10.0 Hz, H-2), 3.95 (1H, dd, J5’,6’b = 5.5, J6’a,6’b = 12.0 Hz, H-6’b), 4.07 (1H, dd, J3,4 = 3.5, J4,5 = 1.0 Hz, H-4), 4.30 (1H, dd, J5’,6’a = 2.0, J6’a,6’b = 12.0 Hz, H-6’a), 4.81 (1H, d, J1,2 = 4.0 Hz, H-1), 4.90 (1H, d, J1’,2’ = 8.0 Hz, H-1’), 5.02 (1H, dd, J1’,2’ = 8.0, J2’,3’ = 9.5 Hz, H-2’), 5.08 (1H, dd, J3’,4’ = 9.5, J4’,5’ = 10.0 Hz, H-4’), 5.34 (1H, t, J2’,3’ = J3’,4’ = 9.5 Hz, H-3’). 13C-NMR (75 MHz, CDCl3) δ: 61.67 (C-6’), 62.75 (C-6), 67.82 (C-4’), 68.19 (C-2), 69.23 (C-5), 69.80 (C-4), 71.45 (C-2’), 71.69 (C-3’), 72.41 (C-5’), 78.99 (C-3), 99.42 (C-1), 101.12 (C-1’).
Methyl O-2,3,4,6-tetra-O-pivaloyl-β-D-glucopyranosyl-(1→2)-β-D-glucopyranoside (17):
Colorless syrup: [α]D21.9 +3.73 (c 1.00, CHCl3). HRMS (FAB) m/z: Calcd for C33H56O15Na [M+Na]+ 715.3511; found 715.3514. 1H-NMR (400 MHz, CDCl3) δ: 3.26 (1H, dd, J1,2 = 8.0, J2,3 = 9.0 Hz, H-2), 3.34 (1H, ddd, J4,5 = 9.0, J5,6a = 3.5, J5,6b = 4.5 Hz, H-5), 3.52 (1H, t, J3,4 = J4,5 = 9.0 Hz, H-4), 3.56 (1H, t, J2,3 = J3,4 = 9.0 Hz, H-3), 3.73 (1H, ddd, J4’,5’ = 10.0, J5’,6’a = 1.5, J5’,6’b = 3.5 Hz, H-5’), 3.80 (1H, dd, J5,6b = 4.5, J6a,6b = 12.0 Hz, H-6b), 3.90 (1H, dd, J5,6a = 3.5, J6a,6b = 12.0 Hz, H-6a), 4.09 (1H, dd, J5’,6’b = 3.5, J6’a,6’b = 12.5 Hz, H-6’b), 4.25 (1H, dd, J5’,6’a = 1.5, J6’a,6’b = 12.5 Hz, H-6’a), 4.36 (1H, d, J1,2 = 8.0 Hz, H-1), 4.85 (1H, d, J1’,2’ = 8.0 Hz, H-1’), 5.00(1H, dd, J1’,2’ = 8.0, J2’,3’ = 9.5 Hz, H-2’), 5.19 (1H, dd, J3’,4’ = 9.5, J4’,5’ = 10.0 Hz, H-4’), 5.34 (1H, t, J2’,3’ = J3’,4’ = 9.5 Hz, H-3’). 13C-NMR (100 MHz, CDCl3) δ: 61.50 (C-6’), 62.32 (C-6), 67.46 (C-4’), 69.96 (C-4), 72.14 (C-3’), 72.18 (C-5’), 72.42 (C-2’), 74.99 (C-5), 75.51 (C-3), 81.52 (C-2), 100.91 (C-1’), 102.93 (C-1).
Methyl O-2,3,4,6-tetra-O-pivaloyl-β-D-glucopyranosyl-(1→3)-β-D-glucopyranoside (18):
Colorless syrup: [α]D19.7 –2.94 (c 1.00, CHCl3). HRMS (FAB) m/z: Calcd for C33H56O15Na [M+Na]+ 715.3511; found 715.3540. 1H-NMR (400 MHz, CDCl3) δ: 3.33 (1H, ddd, J4,5 = 9.0, J5,6a = 3.5, J5,6b = 5.0 Hz, H-5), 3.36 (1H, dd, J1,2 = 8.0, J2,3 = 9.0 Hz, H-2), 3.45 (1H, t, J2,3 = J3,4 = 9.0 Hz, H-3), 3.49 (1H, t, J3,4 = J4,5 = 9.0 Hz, H-4), 3.76 (1H, dd, J5,6b = 5.0, J6a,6b = 12.0 Hz, H-6b), 3.79 (1H, ddd, J4’,5’ = 10.0, J5’,6’a = 2.0, J5’,6’b = 6.0 Hz, H-5’), 3.92 (1H, dd, J5,6a = 2.0, J6a,6b = 12.0 Hz, H-6a), 3.99 (1H, dd, J5’,6’b = 6.0, J6’a,6’b = 12.0 Hz, H-6’b), 4.18 (1H, d, J1,2 = 8.0 Hz, H-1), 4.25 (1H, dd, J5’,6’a = 2.0, J6’a,6’b = 12.0 Hz, H-6’a), 4.79 (1H, d, J1’,2’ = 8.0 Hz, H-1’), 5.05 (1H, dd, J1’,2’ = 8.0, J2’,3’ = 9.5 Hz, H-2’), 5.09 (1H, dd, J3’,4’ = 9.5, J4’,5’ = 10.0 Hz, H-4’), 5.35 (1H, t, J2’,3’ = J3’,4’ = 9.5 Hz, H-3’). 13C-NMR (100 MHz, CDCl3) δ: 61.78 (C-6’), 62.83 (C-6), 67.86 (C-4’), 69.32 (C-4), 71.36 (C-2’), 71.92 (C-3’), 72.43 (C-5’), 72.96 (C-2), 75.49 (C-5), 85.99 (C-3), 101.38 (C-1’), 103.62 (C-1).
Methyl O-2,3,4,6-tetra-O-pivaloyl-β-D-glucopyranosyl-(1→2)-α-D-glucopyranoside (20):
Colorless syrup. [α]D22.5 +54.7 (c 1.00, CHCl3). HRMS (FAB) m/z: Calcd for C33H56O15Na [M+Na]+ 715.3511; found 715.3521. 1H-NMR (300 MHz, CDCl3) δ: 3.45 (1H, dd, J1,2 = 3.5, J2,3 = 10.0 Hz, H-2), 3.53 (1H, dd, J3,4 = 9.0, J4,5 = 9.5 Hz, H-4), 3.63 (1H, dt, J4,5 = 9.5, J5,6a = J5,6b = 3.5 Hz, H-5), 3.74 (1H, ddd, J4’,5’ = 10.0, J5’,6’a = 1.5, J5’,6’b = 5.5 Hz, H-5’), 3.79 (1H, dd, J5,6a = 3.5, J6a,6b = 11.5 Hz, H-6a), 3.84 (1H, dd, J5,6b = 3.5, J6a,6b = 11.5 Hz, H-6b), 3.90 (1H, dd, J2,3 = 10.0, J3,4 = 9.0 Hz, H-3), 3.99 (1H, dd, J5’,6’b = 5.5, J6’a,6’b = 12.5 Hz, H-6’b), 4.30 (1H, dd, J5’,6’b = 5.5 Hz, J6’a,6’b = 12.5 Hz, H-6’a), 4.81 (1H, d, J1,2 = 3.5 Hz, H-1), 4.85 (1H, d, J1’,2’ = 8.0 Hz, H-1’), 5.08 (1H, dd, J1’,2’ = 8.0 Hz, J2’,3’ = 9.5 Hz, H-2’), 5.11 (1H, dd, J3’,4’ = 9.5 Hz, J4’,5’ = 10.0 Hz, H-4’), 5.33 (1H, t, J2’,3’ = J3’,4’ = 9.5 Hz, H-3’). 13C-NMR (75 MHz, CDCl3) δ: 61.77 (C-6’), 62.22 (C-6), 67.62 (C-4’), 70.55 (C-5), 70.70 (C-4), 71.57 (C-2’), 71.78 (C-3), 72.13 (C-3’), 72.48 (C-5’), 80.48 (C-2), 99.30 (C-1), 101.24 (C-1’).
Methyl O-2,3,4,6-tetra-O-pivaloyl-β-D-glucopyranosyl-(1→3)-α-D-glucopyranoside (21):
Colorless syrup. [α]D23.0 +62.2 (c 1.00, CHCl3). HRMS (FAB) m/z: Calcd for C33H56O15Na [M+Na]+ 715.3511; found 715.3506. 1H-NMR (300 MHz, CDCl3) δ: 3.44 (1H, dd, J3,4 = 8.0, J4,5 = 9.5 Hz, H-4), 3.54 (1H, dd, J1,2 = 4.0, J2,3 = 9.5 Hz, H-2), 3.58 (1H, ddd, J4,5 = 9.5, J5,6a = 3.5, J5,6b = 5.0 Hz, H-5), 3.59 (1H, dd, J3,4 = 8.0, J4,5 = 9.5 Hz, H-3), 3.75 (1H, dd, J5,6b = 5.0, J6a,6b = 11.5 Hz, H-6b), 3.77 (1H, ddd, J4’,5’ = 10.0, J5’,6’a = 2.0, J5’,6’b = 6.0 Hz, H-5’), 3.86 (1H, dd, J5,6a = 3.5, J6a,6b = 11.5 Hz, H-6a), 3.99 (1H, dd, J5’,6’b = 6.0, J6’a,6’b = 12.5 Hz, H-6’b), 4.24 (1H, dd, J5’,6’a = 2.0, J6’a,6’b = 12.5 Hz, H-6’a), 4.73 (1H, d, J1,2 = 4.0 Hz, H-1), 4.76 (1H, d, J1’,2’ = 8.0 Hz, H-1’), 5.05 (1H, dd, J1’,2’ = 8.0, J2’,3’ = 9.5 Hz, H-2’), 5.09 (1H, dd, J3’,4’ = 9.5, J4’,5’ = 10.0 Hz, H-4’), 5.34 (1H, t, J2’,3’ = J3’,4’ = 9.5 Hz, H-3’). 13C-NMR (75 MHz, CDCl3) δ: 61.72 (C-6’), 62.74 (C-6), 67.85 (C-4’), 69.14 (C-4), 71.05 (C-2), 71.12 (C-5), 71.39 (C-2’), 71.94 (C-3’), 72.38 (C-5’), 85.23 (C-3), 99.05 (C-1), 101.54 (C-1’).
Methyl O-2,3,4,6-tetra-O-pivaloyl-β-D-glucopyranosyl-(1→2)-α-L-fucopyranoside (23):
Colorless crystals. Mp 210~212 °C. [α]D22.5 –38.6 (c 0.97, CHCl3). HRMS (FAB) m/z: Calcd for C33H56O14Na [M+Na]+ 699.3568; found 699.3560. 1H-NMR (300 MHz, CDCl3) δ: 1.28 (3H, d, J5,6 = 6.0 Hz, H-6), 3.78 (1H, ddd, J4’,5’ = 10.0, J5’,6’a = 2.0, J5’,6’b = 6.0 Hz, H-5’), 3.79 (1H, dd, J1,2 = 4.0, J2,3 = 9.5 Hz, H-2), 3.82 (1H, dd, J3,4 = 3.5, J4,5 = 1.0 Hz, H-4), 3.90 (1H, dq, J4,5 = 1.0, J5,6 = 6.0 Hz, H-5), 3.94 (1H, dd, J2,3 = 9.5, J3,4 = 3.5 Hz, H-3), 3.95 (1H, dd, J5’,6’b = 6.0, J6’a,6’b = 12.5 Hz, H-6’b), 4.33 (1H, dd, J5’,6’a = 2.0, J6’a,6’b = 12.5 Hz, H-6’a), 4.60 (1H, d, J1’,2’ = 8.0 Hz, H-1’), 4.66 (1H, d, J1,2 = 4.0 Hz, H-1), 5.06 (1H, dd, J1’,2’ = 8.0, J2’,3’ = 9.5 Hz, H-2’), 5.10 (1H, dd, J3’,4’ = 9.5, J4’,5’ = 10.0 Hz, H-4’), 5.32 (1H, t, J2’,3’ = J3’,4’ = 9.5 Hz, H-3’). 13C-NMR (75 MHz, CDCl3) δ: 15.93 (C-6), 61.49 (C-6’), 65.11 (C-5), 67.46 (C-4’), 68.20 (C-3), 71.07 (C-2’), 71.19 (C-4), 71.90 (C-3’), 72.75 (C-5’), 80.35 (C-2), 97.99 (C-1), 101.54 (C-1’).
Methyl O-2,3,4,6-tetra-O-pivaloyl-β-D-glucopyranosyl-(1→4)-α-L-rhamnopyranoside (25):
Colorless syrup. [α]D22.0 –28.3 (c 1.00, CHCl3). HRMS (FAB) m/z: Calcd for C33H56O14Na [M+Na]+ 699.3562; found 699.3571 [M+Na]+. 1H-NMR (300 MHz, CDCl3) δ: 1.27 (3H, d, J5,6 = 6.0 Hz, H-6), 3.50 (1H, dd, J3,4 = 9.0 J4,5 = 9.5 Hz, H-4), 3.61 (1H, dq, J4,5 = 9.5, J5,6 = 6.0 Hz, H-5), 3.70 (1H, ddd, J4’,5’ = 10.0, J5’,6’a = 2.0, J5’,6’b = 5.0 Hz, H-5’), 3.76 (1H, dd, J2,3 = 3.5, J3,4 = 9.0 Hz, H-3), 3.85 (1H, dd, J1,2 = 1.5, J2,3 = 3.5 Hz, H-2), 3.98 (1H, dd, J5’,6’b = 5.0, J6’a,6’b = 12.0 Hz, H-6’b), 4.25 (1H, dd, J5’,6’a = 2.0, J6’a,6’b = 12.0 Hz, H-6’a), 4.63 (1H, d, J1,2 = 1.5 Hz, H-1), 4.95-5.02 (2H, m, J1’,2’ = 7.0 Hz, H-1’, 2’), 5.12 (1H, dd, J3’,4’ = 9.5, J4’,5’ = 10.0 Hz, H-4’), 5.32 (1H, t, J2’,3’ = J3’,4’ = 9.5 Hz, H-3’). 13C-NMR (75 MHz, CDCl3) δ: 17.57 (C-6), 61.71 (C-6’), 66.02 (C-5), 67.98 (C-4’), 71.17 (C-2), 71.39 (C-3), 71.83 (C-2’), 71.98 (C-5’), 72.30 (C-3’), 79.32 (C-4), 100.25 (C-1), 100.59 (C-1’).
ACKNOWLEDGEMENTS
We are indebted to Mses. Michiko Sato, Akiko Nakagawa, and Chikako Sakabe at the Analytical Center of the School of Pharmacy, Kitasato University for the NMR and MS measurements. Financial support from the Ministry of Education, Culture, Sports, Science, and Technology (Grant-in-Aid for Scientific Research No. 19590010) is gratefully acknowledged.
References
1. a) P. Stallforth, B. Lepenies, A. Adibekian, and P. H. Seeberger, J. Med. Chem., 2009, 52, 5561; CrossRef b) D. P. Galonic and D. Y. Gin, Nature, 2007, 446, 1000; CrossRef c) B. O. Fraser-Reid, K. Tatsuta, and T. Thiem, eds., Glycoscience Chemistry and Chemical Biology, 2nd Ed., Springer: Berlin, 2008.
2. a) A. V. Demchenko, Handbook of Chemical Glycosylation; Wiley-VCH: Weinheim, 2008; CrossRef b) D. Crich and M. Smith, J. Am. Chem. Soc., 2001, 123, 9015; CrossRef c) B. Ernst, W. Hart, and P. Sinay, Carbohydrate in Chemistry and Biochemistry; Wiley-VCH: Weinheim, 2008; d) K. Toshima and K. Tatsuta, Chem. Rev., 1993, 93, 1503; CrossRef e) P. Sinay, Pure Appl. Chem., 1991, 63, 519; CrossRef f) H. Kunz, Angew. Chem., Int. Ed. Engl., 1987, 26, 294; CrossRef g) R. R. Schmidt, Angew. Chem., Int. Ed. Engl., 1986, 25, 212; CrossRef h) H. Paulsen, Angew. Chem., Int. Ed. Engl.,1982, 21, 155. CrossRef
3. a) C.-C. Wang, J.-C. Lee, S.-Y. Luo, S. S. Kulkarni, Y.-W. Huang, C.-C. Lee, K.-L. Chang, and S.-C. Hung, Nature, 2007, 446, 896; CrossRef b) T. Kawabata and T. Furuta, Chem. Lett., 2009, 38, 640; CrossRef c) Y. Demizu, Y. Kubo, H. Miyoshi, T. Maki, Y. Matsumura, N. Moriyama, and O. Onomura, Org. Lett., 2008, 10, 5075; CrossRef d) T. Maki, N. Ushijima, Y. Matsumura, and O. Onomura, Tetrahedron Lett., 2009, 50, 1466; CrossRef e) D. Lee and M. S. Taylor, J. Am. Chem. Soc., 2011, 133, 3724; CrossRef f) L. Chan and M. S. Taylor, Org. Lett., 2011, 13, 3090. CrossRef
4. P. J. Garegg, J. Maloisel, and S. Oscarson, Synthesis, 1995, 409. CrossRef
5. R. K. P. Kartha, M. Kiso, A. Hasegawa, and H. L. Jennings, J. Chem. Soc., Perkin 1, 1995, 3023. CrossRef
6. E. Kaji and N. Harita, Tetrahedron Lett., 2000, 41, 53. CrossRef
7. E. Kaji, K. Shibayama, and K. In, Tetrahedron Lett., 2003, 44, 4881. CrossRef
8. a) K. Oshima and Y. Aoyama, J. Am. Chem. Soc., 1999, 121, 2315; CrossRef b) K. Oshima, T. Yamauchi, M. Shimomura, and Y. Aoyama, Bull. Chem. Soc. Jpn., 2002, 75, 1319; CrossRef c) Recently Taylor et al., reported a new method for catalytic regioselective activation of glycosyl acceptors by a diarylborinic acid derivative: C. Gouliaras, D. Lee, L. Chan, and M. S. Taylor, dx.doi.org/10.1021/ja2062715|J.Am.Chem.Soc. CrossRef
9. A part of this work has been published as a preliminary communication: E. Kaji, T. Nishino, K. Ishige, Y. Ohya, and Y. Shirai, Tetrahedron Lett., 2010, 51, 1570. CrossRef
10. a) K.-W. Yu, H. Kiyohara, T. Matsumoto, H.-C. Yang, and H. Yamada, Planta Medica, 1998, 64, 714; CrossRef b) A. E. Clarke, R. L. Anderson, and B. A. Stone, Phytochemistry, 1979, 18, 521; CrossRef c) I. Taguchi, H. Kiyohara, T. Matsumoto, and H. Yamada, Carbohydr. Res., 2004, 339, 763. CrossRef
11. H. Kunz and A. Harreus, Liebigs Ann. Chem., 1982, 41. CrossRef
12. C. A. A. van Boeckel, T.Beetz, A. C. Kock-van Dalen, and H. van Bekkum, Recl. Trav. Chim. Pays-Bas, 1987, 106, 596; CrossRef Ag(I) on silica alumina has been found to be an efficient promoter for Koenigs-Knorr type glycosylation using glycosyl bromide as a donor, comparing with other silver catalysts as Ag2CO3 and AgOTf, etc., as described in Ref. 6 and E. Kaji, F. W. Lichtenthaler, Y. Osa, K. Takahashi, and S. Zen, Bull. Chem. Soc. Jpn., 1995, 68, 2401 and the preceding papers cited therin.