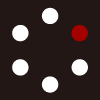
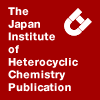
HETEROCYCLES
An International Journal for Reviews and Communications in Heterocyclic ChemistryWeb Edition ISSN: 1881-0942
Published online by The Japan Institute of Heterocyclic Chemistry
e-Journal
Full Text HTML
Received, 25th July, 2011, Accepted, 26th September, 2011, Published online, 27th September, 2011.
DOI: 10.3987/COM-11-S(P)86
■ [3+2] Cycloaddition of Seleno- and Thioaldehydes with Cyclic Azomethine Imines
Hajime Maeda, Yusuke Takamizawa, and Masahito Segi*
Chemistry Course, College of Science and Engineering, School of Chemistry, Kanazawa University, Kakuma, Kanazawa, Ishikawa 920-1192, Japan
Abstract
Reaction of seleno- and thioaldehydes with cyclic azomethine imines in refluxing toluene gave [3+2] cycloadducts as a diastereomeric pure form in high yields. Thermal cycloreversion of the [3+2] cycloadducts regioselectively occurred to regenerate seleno- and thioaldehydes which can be trapped in situ by 2,3-dimethyl-1,3-butadiene.Selenocarbonyl compounds, for example, selenoketones, selenoaldehydes, and selenoamides, often exhibit unique reactivities that can not be seen in chemistry of the corresponding carbonyl compounds. Especially, cycloaddition reactions involving selenocarbonyl compounds have attracted much attention due to their high synthetic potential for construction of selenium-containing heterocyclic compounds.1-6 As for the synthetic route of the selenocarbonyl compounds, we have developed a convenient direct conversion of carbonyl compounds to the corresponding selenocarbonyl compounds by reaction with bis(dimethylaluminum) selenide in high yields.7-20 Among several selenocarbonyl compounds, selenoaldehydes (1) can be hardly isolated in general because they are extremely unstable compounds in air, but they can be trapped by anthracene in situ to give [4+2] cycloadducts 2 as stable and isolable
compounds.16-19 We have also developed that thermal retro hetero-Diels Alder reaction of 2 at approximately 110 °C restores 1 under neutral conditions, and that they react with linear 1,3-dipole molecules such as nitrile oxides and nitrile imines to give selenium-containing five-membered ring products, 1,4,2-oxaselenazoles and 1,3,4-selenadiazoles, respectively.21 Our continuing interest on the synthesis of selenium-containing heterocycles let us examine reactions employing some nonlinear 1,3-dipoles, but good results have not been obtained. In this study, we found that by using cyclic azomethine imines, which are representative rigid nonlinear 1,3-dipoles that often used to synthesis of diaza-heterocycles by reaction with various unsaturated compounds,22-32 high-yield synthesis of novel selenium-containing bicyclic heterocycles could be achieved by reaction with selenoaldehydes 1 generated thermally in situ from 2. Moreover, we also clarified that the reaction could be applied to synthesis of sulfur-containing analogues by the reaction using thioaldehydes.
Cyclic azomethine imines used in this study, 2-arylmethylidene-5-oxopyrazolidin-2-ium-1-ides (3), are known to be stable compounds that can be easily prepared by reaction of pyrazolin-3-one with benzaldehyde derivatives as a stereoisomeric pure form (Z form).27 A toluene solution containing Diels-Alder adduct of selenobenzaldehyde (2a) and (Z)-2-benzylidene-5-oxopyrazolidin-2-ium-1-ide (3a) was refluxed for 3 h under argon atmosphere (Table 1, entry 1). Purification by silica gel column chromatography gave 1,3-diphenyldihydro-1H-pyrazolo[1,2-c][1,3,4]selenadiazol-5(3H)-one (4a) in 19% isolated yield as a single stereoisomer, trans form (vide infra). Shortening of the reaction time resulted in increase of the yields (entries 2 and 3), and the best yield was obtained when the reaction was carried out at 110 °C for 10 min (entry 4).33 Both elevated reaction temperature (150 °C) and lowered one (90 °C) did not improve the yield (entries 5 and 6). p-Methoxy- and p-cyano-substituted selenobenzaldehydes (1b-c) generated in situ also reacted with 3a to give the corresponding heterocycles 4b-c in high yields (entries 7 and 8). Selenobutanal (1d), an aliphatic selenoaldehyde, could also be used in the [3+2] cycloaddition when it was reacted with 3a at 160 °C (for requirement of generation of aliphatic selenoaldehydes17), giving 4d in 69% yield as a 91 : 9 diastereomeric mixture (entry 9). Cyclic azomethine imines 3b-f having p-methylphenyl, p-methoxyphenyl, p-trifluoromethylphenyl, 1-naphthyl, and tert-butyl groups could also be used to give [3+2] cycloaddition products 4e-i in 62-88% yields (entries 10-14). Combination of double electron-withdrawing aryl groups in 2 and 3 made the yield increase up to 95% (entry 15). Similarly, thiobenzaldehydes 5 generated by thermal reaction from analogous precursors 6a-d reacted with 3a to give the corresponding sulfur analogues 7a-d in high yields (entries 16-19).
All products listed in Table 1 were obtained as a single stereoisomer with the exception of 4d and 7d which contained small amounts of another diastereomers. On the contrary, when thione derivative 8 was
allowed to react with 2a, the product 9 was produced in 52 : 48 diastereomer ratio (Scheme 2). NOE irradiation experiments indicated that 5.7% enhancement of integration of NMR signals between two benzylic hydrogens (H1 and H2) of 9 was observed only in one isomer and the enhancement was not observed from another isomer, so each benzylic hydrogens can be assigned to cis and trans isomers, respectively. Since NOE irradiation of 4a under the same conditions did not show any enhancement, we determined the stereochemistry of 4a as trans isomer. The trans configuration is also expected to other products 4b-j and 7a-d from similarity of their 1H NMR spectra with that of 4a.
From these results, we propose a possible mechanism for the [3+2] cycloaddition as shown in Scheme 3. Excellent regioselectivity can be explained by interaction of LUMO of 1 and HOMO of 3, whose orbital coefficients spread on selenocarbonyl carbon of 1 and nitrogen at 1-position of 3 larger than the selenium and benzylidene carbon, respectively.28, 34-36 As for the excellent stereoselectivity giving trans isomers, we would like to propose effective orbital interaction between aryl group of 1 and π orbitals including carbonyl group of 3. Hence, when the two addends approach each other, TS I where aryl group of 1 locates on endo-position toward 3 may be favored rather than TS II where the aryl group locates on exo-position. The large difference of the cis / trans ratio between the reactions using 3a and 8 may be due to the weakened orbital interaction between 1 and 8.
From the entries 1-4 in Table 1, the time profile suggests that thermal decomposition of the product 4 might be involved. Indeed, when reaction of 2b with 3a was carried out at 110 °C for 1 h, a diphenyl
derivative 4a was obtained in a minor extent with the formation of main product 4b, in spite of that unsubstituted selenobenzaldehyde should not be formed from thermolysis of 2b (Scheme 4). These results prompted us to investigate thermal cycloreversion of 4 at 110 °C to regenerate selenoaldehydes 1 (Table 2). When 4b was allowed to treat with excess amount of 2,3-dimethyl-1,3-butadiene at 110 °C for 3 h, Diels-Alder adducts 10a and 10b, which indicate generation of p-methoxyselenobenzaldehyde and unsubstituted selenobenzaldehyde, were obtained in 82% and 3% isolated yields, respectively (entry 1). Reaction of some other derivatives 4c, 4e, and 4g, or thio derivatives 7b-c, also resulted in major production of 10 or 11 and minor production of 10’ or 11’ (entries 2-6).
From these results, the regioselective cycloreversion of 4 and 7 giving seleno- and thioaldehydes 1 and 5, respectively, is rationalized as depicted in Scheme 5. Thermal bond cleavage at position a, i.e. the retro reaction of the cycloaddition, gives the starting materials 1 and 3, whereas bond cleavage at position b produces 1’ and 1,3-dipole species 3’. As expected from structures 3 and 3’, 3’ seems to be thermodynamically more unstable than 3 because 3’ has cationic nitrogen atom on α-position of
electron-withdrawing carbonyl group. This assumption is not inconsistent with the results that generation of 1 is a major pathway and that of 1’ is a minor pathway. Existence of route b in Scheme 5 is also supported from the experimental results in the reaction of 2b with 3a shown in Scheme 4. As a reason for decreasing the yield of 4 by longer reaction time of [3+2] cycloaddition at 110 °C (entries 1-4 in Table 1), the [3+2] cycloaddition proceeds in an equilibrium with regioselective cycloreversion, so the reproduced selenoaldehyde gradually decomposes during the reaction.
In conclusion, we have developed a novel and stereoselective [3+2] cycloaddition reaction between seleno- and thioaldehydes generated in situ with cyclic azomethine imines to give novel selenium-containing bicyclic heterocycles in high yields. Trans configuration of 1,3-diaryl groups of products was determined by NOE experiments of thione derivatives. Regio- and setereoselectivity can be explained by orbital coefficients of two addends and orbital overlapping at transition states, respectively. Cycloreversion giving selenoaldehydes and cyclic azomethine imines competes with the [3+2] cycloaddition, and it occurs at two types of bond cleavage patterns, in which retro-type reaction is a major pathway.
ACKNOWLEDGEMENTS
This work was partially supported by Grant-in-Aids for Scientific Research (C) from the Ministry of Education, Culture, Sports, Science and Technology (MEXT) of Japan.
References
1. M. Renson, ‘The Chemistry of Organic Selenium and Tellurium Compounds’, Vol. 1, ed. by S. Patai and Z. Rappoport, John Wiley & Sons, New York, 1986, pp. 399-516.
2. A. R. Katritzki, C. W. Rees, and E. F. V. Scriven, ‘Comprehensive Heterocyclic Chemistry II. A Review of the Literature 1982-1995’, Vol. 1-11, Elsevier Science, Oxford, 1996.
3. V. P. Litvinov and V. D. Dyachenko, Russ. Chem. Rev., 1997, 66, 923. CrossRef
4. D. R. Garud, M. Koketsu, and H. Ishihara, Molecules, 2007, 12, 504. CrossRef
5. H. Heimgartner, Y. Zhou, P. K. Atanassov, and G. L. Sommen, Phosphorus, Sulfur, Silicon, Rel. Elem., 2008, 183, 840.
6. A. R. Katritzki, C. A. Ramsden, E. F. V. Scriven, and R. J. K. Taylor, ‘Comprehensive Heterocyclic Chemistry III. A Review of the Literature 1995-2007’, Vol. 1-15, Elsevier Science, Oxford, 2008.
7. M. Segi, T. Koyama, T. Nakajima, S. Suga, S. Murai, and N. Sonoda, Tetrahedron Lett., 1989, 30, 2095. CrossRef
8. M. Segi and S. Suga, J. Synth. Org. Chem., Jpn., 1990, 48, 1064. CrossRef
9. M. Segi, A. Kojima, T. Nakajima, and S. Suga, Synlett, 1991, 105. CrossRef
10. G. M. Li, M. Segi, and T. Nakajima, Tetrahedron Lett., 1992, 33, 3515. CrossRef
11. M. Segi, T. Takahashi, H. Ichinose, G. M. Li, and T. Nakajima, Tetrahedron Lett., 1992, 33, 7865. CrossRef
12. G. M. Li, T. Kamogawa, M. Segi, and T. Nakajima, Chem. Express, 1993, 8, 53.
13. M. Segi and T. Nakajima, J. Synth. Org. Chem., Jpn., 1995, 53, 678. CrossRef
14. G. M. Li, R. A. Zingaro, M. Segi, J. H. Reibenspies, and T. Nakajima, Organometallics, 1997, 16, 756. CrossRef
15. G. M. Li, S. Niu, M. Segi, R. A. Zingaro, H. Yamamoto, K. Watanabe, T. Nakajima, and M. B. Hall, J. Org. Chem., 1999, 64, 1565. CrossRef
16. A. Zhou, M. Segi, and T. Nakajima, Tetrahedron Lett., 2003, 44, 1179. CrossRef
17. M. Segi, J. Synth. Org. Chem., Jpn., 2003, 61, 661. CrossRef
18. M. Segi, A. Zhou, and M. Honda, Phosphorus, Sulfur, Silicon, Rel. Elem., 2005, 180, 1045.
19. M. Segi, K. Kawaai, M. Honda, and S. Fujinami, Tetrahedron Lett., 2007, 48, 3349. CrossRef
20. H. Maeda, M. Takashima, K. Sakata, T. Watanabe, M. Honda, and M. Segi, Tetrahedron Lett., 2011, 52, 415. CrossRef
21. M. Segi, K. Tanno, M. Kojima, M. Honda, and T. Nakajima, Tetrahedron Lett., 2007, 48, 2303. CrossRef
22. R. Grashey, ‘1,3-Dipolar Cycloaddition Chemistry’, Vol. 1, ed. by A. Padwa, John Wiley & Sons, New York, 1984, pp. 733-817.
23. R. Grigg, J. Kemp, and N. Thompson, Tetrahedron Lett., 1978, 19, 2827. CrossRef
24. R. Huisgen, R. Fleischmann, and A. Eckell, Chem. Ber., 1977, 110, 500. CrossRef
25. K.-I. Washizuka, K. Nagai, S. Minakata, I. Ryu, and M. Komatsu, Tetrahedron Lett., 2000, 41, 691. CrossRef
26. C. Turk, J. Svete, B. Stanovnik, L. Golic, S. Golic-Grdadolnik, A. Golobic, and L. Selic, Helv. Chim. Acta, 2001, 84, 146. CrossRef
27. R. Shintani and G. C. Fu, J. Am. Chem. Soc., 2003, 125, 10778. CrossRef
28. C. W. G. Fishwick, R. Grigg, V. Sridharan, and J. Virica, Tetrahedron, 2003, 59, 4451. CrossRef
29. H. Suga, A. Funyu, and A. Kakehi, Org. Lett., 2007, 9, 97. CrossRef
30. A. Chan and K. A. Scheidt, J. Am. Chem. Soc., 2007, 129, 5334. CrossRef
31. J. G. Schantl, Adv. Heterocycl. Chem., 2010, 99, 185. CrossRef
32. S. Chassaing, A. Alix, T. Boningari, K. S. S. Sido, M. Keller, P. Kuhn, B. Louis, J. Sommer, and P. Pale, Synthesis, 2010, 1557. CrossRef
33. Typical procedure for [3+2] cycloaddition: To an argon-purged, two-necked, 20 mL round-bottom flask equipped with a reflux condenser and a magnetic stirrer, toluene (6 mL), compound 2a (174 mg, 0.5 mmol), and cyclic azomethine imine (3a, 261 mg, 1.5 mmol) were placed. The solution was heated to 110 °C by an oil bath and stirred for 10 min. After cooling to 0 °C by an ice bath, saturated NH4Cl aqueous solution was added. The organic layer was extracted, dried over MgSO4, and evaporated. Column chromatography on silica gel (eluent; hexane : ethyl acetate = 2 : 1) gave pure product, trans-1,3-diphenyldihydro-1H-pyrazolo[1,2-c][1,3,4]selenadiazol-5(3H)-one (4a, 130 mg, 76% yield). Spectral data for 4a: 1H NMR (270 MHz, CDCl3): δ 7.61 – 7.23 (m, 10H), 6.74 (s, 1H), 5.52 (s, 1H), 3.60 (ddd, J = 12.7, 10.7, 8.8 Hz, 1H), 3.16 – 3.07 (m, 1H), 2.91 (ddd, J = 9.0, 10.7, 17.6 Hz, 1H), 2.49 (ddd, J = 17.6, 8.8, 2.0 Hz, 1H); 13C NMR (68 MHz, CDCl3): δ 173.1, 141.7, 135.5, 128.9, 128.7, 128.5, 127.7, 126.5, 73.5, 53.2, 44.7, 28.8; 77Se NMR (76 MHz, CDCl3): δ 692.66; MS (EI), m/e (relative intensity): 344 (M+, 98.0), 260 (12.6), 175 (100), 170 (98.3), 105 (54.5), 84 (33.5); HRMS (EI) Calcd for C17H16N2OSe: 344.0428; Found: 344.0430.
34. E. Vedejs, D. A. Perry, and R. G. Wilde, J. Am. Chem. Soc., 1986, 108, 2985. CrossRef
35. P. T. Meinke, G. A. Krafft, and J. T. Spencer, Tetrahedron Lett., 1987, 28, 3887. CrossRef
36. K. Urbaniak, R. Szymanski, J. Romanski, G. Mloston, M. Domagala, A. Linden, and H. Heimgartner, Helv. Chim. Acta, 2004, 87, 496. CrossRef