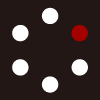
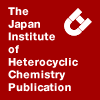
HETEROCYCLES
An International Journal for Reviews and Communications in Heterocyclic ChemistryWeb Edition ISSN: 1881-0942
Published online by The Japan Institute of Heterocyclic Chemistry
e-Journal
Full Text HTML
Received, 30th July, 2011, Accepted, 14th October, 2011, Published online, 24th October, 2011.
DOI: 10.3987/COM-11-S(P)89
■ Reactions of an Overcrowded Silylene with Pyridines: Formation of a Novel 2H-1,2-Azasilepine and Its Further Cycloaddition
Yoshiyuki Mizuhata, Takahiro Sato, and Norihiro Tokitoh*
Institute for Chemical Research, Kyoto University, Uji, Kyoto 611-0011, Japan
Abstract
The reactions of an overcrowded silylene [Tbt(Mes)Si:] with two types of pyridines were performed. In the case using pyridine, a unique 2:2 adduct bearing five, six, and seven-membered rings was obtained. On the other hand, the reaction with N,N-dimethyl-4-aminopyridine (DMAP) afforded the corresponding 1:1 adduct having a 2H-1,2-azasilepine skeleton.Silylenes (>Si:) are heavier congeners of carbene (>C:), that is, divalent silicon species without any electronic charge. They are usually postulated as reactive intermediates and the examples were quite limited for isolable and/or stable silylenes.1
The reactivity of silylenes has been widely investigated, and various kinds of cycloaddition and insertion reactions have been reported.1 Among them, we have already reported the synthesis of the extremely hindered disilene 1 and revealed its thermal dissociation into the corresponding silylene 2 for the first time (Scheme 1).2,3 Silylene 2 was found to react even with inert arenes, e.g., benzene, naphthalene, giving the corresponding 2:1 adducts.3 In the case of benzene, [1+2] cycloadduct was generated initially, and then the ring expansion giving silepin 3 and the successive addition of another silylene occurred to afford 4 (Scheme 2). Similar reactivity in photochemical conditions was reported by Kira and his co-workers, and the corresponding silepins were isolated.4 In the course of our research on the synthetic utility of silylene 2, we found here the unique reactivity of 2 towards pyridines.
The reaction of silylene 2 generated from disilene 1 with pyridine afforded the 2:2 adduct 5 in 30% yield (Scheme 3).5 Interestingly, expected 2:1 adduct like 4 was not generated, but another pyridine molecule got involved in the skeleton of 5. Although various stereoisomers are considerable for the structure of 5, only one pair of the enantiomers was generated in this reaction as judged by the 1H NMR spectrum of the crude mixture. The structure of 5 was reasonably supported by the 1H and 13C NMR and mass spectra, and finally determined by X-ray crystallographic analysis.6 The whole and core structures of 5 are shown in Figure 1. The reactions of diamino-substituted silylene 6 with pyridine or quinoline were performed by Lappert and his co-workers (Scheme 3).7 In contrast to our results, 2:1 adducts 7 having a disilane moiety were obtained, and no C–N bond cleavage occurred.
Possible reaction pathways for the formation of 5 are summarized in Scheme 4. Taking account into consideration of the cleavage of not C–C but C–N bond on a pyridine molecule, silylene-pyridine complex 8 is considered to be generated initially. Its lone pair on the silicon atom could attack the ortho-carbon of the pyridine ring giving [1+2] adduct 9 and then ring-expanded compound, 2H-1,2-azasilepine 10. As for the base-stabilized silylene such as compound 8, we have already reported the silylene–isocyanide complexes as thermally stable compounds by the reaction of 2 with isocyanides.8 In addition, since silylene 2 can react with pivalonitlile to afford the corresponding [1+2] adduct, that is, SiNC three-membered ring compound, 2H-1,2-azasilirene,9 it is possible to form intermediate
9 bearing a three-membered ring similarly to the case of the reaction of 2 with benzene. Then, silylene 2 reacted with the less hindered C=C bond of 10 to afford 11. Although the nitrogen atom of azasilepine 10 can interact with 2 and give the corresponding SiNC three-membered ring, steric and electronic (decrease of nucleophilicity due to the neighboring silicon atom) demands prevented such kind of reactivity. At the final stage, the nitrogen atom of a pyridine molecule attacked to the silicon atom of 11 resulting in the opening of the silacyclopropane ring and the formation of C–C bond. Release of the ring strain of the three-membered ring in 11 and the relative stability of the resulting carbanion are presumably the driving force of the ring-opening reaction followed by the second addition of pyridine molecule. On the other hand, (E)-4 having a skeleton similar to that of 11 did not react with pyridine under similar conditions (10 equiv. of pyridine at 70 ºC in THF for 10 h), suggesting that the ring strain of the silacyclopropane unit in 11 is not the dominant factor for the formation of final product 5 but the stabilization
of the ring-opened carbainon 12 by its imine unit is of great importance for the ring opening reaction and the regioselective ring annelation giving 5.
In order to investigate the initial step, the reaction of 2 with DMAP (N,N-dimethyl-4-aminopyridine) was performed. Steric hindrance around the 5-position of the silepin skeleton and the electron-withdrawing character of NMe2 may suppress the second addition of silylene 2. As expected, 1:1 adduct 13 having 2H-1,2-azasilepine skeleton was isolated as a stable product in 29% yield (Scheme 5).10 As far as we know, this is the first example of a 2H-1,2-azasilepine skeleton. Compound 13 was fully characterized by the 1H and 13C NMR and mass spectra, and the molecular structure was finally determined by X-ray crystallographic analysis.11 The whole and core structures of 13 are shown in Figure 2 together with the selected bond lengths. The NMe2 moiety and C1-4 atoms are almost in same plane, and N1, C5, and Si1 atoms are deviated from that plane.
In summary, we have found the unique reactivity of an overcrowded silylene 2 with pyridines giving the first examples of 2H-1,2-azasilepine derivatives. These results indicated that a silylene can activate not only the C=C bond but also the C=N bond in an aromatic ring. Since silyl substituents are known to be removable under appropriate conditions, activation by silylenes has a potential leading to a novel method for the chemical conversion of inactive bonds.
ACKNOWLEDGEMENTS
This work was supported by Grants-in-Aid for Creative Scientific Research (No. 17GS0207), Scientific Research (B) (No. 22350017), Scientific Research on Innovative Areas (No. 23108707, “π-Space”), Young Scientist (B) (No. 21750042), and the Global COE Program (B09, “Integrated Materials Science”) from the Ministry of Education, Culture, Sports, Science and Technology, of Japan. Y.M. thanks Mitsubishi Chemical Corp., the Society of Synthetic Organic Chemistry, Japan, and Kinki Invention Center, for financial support. T.S. is grateful for JSPS fellowship for young scientists.
References
1. For recent reviews, see: Y. Mizuhata, T. Sasamori, and N. Tokitoh, Chem. Rev., 2009, 109, 3479; CrossRef M. Kira, T. Iwamoto, and S. Ishida, Bull. Chem. Soc. Jpn., 2007, 80, 258; CrossRef M. Kira, J. Organomet. Chem., 2004, 689, 4475;; CrossRef M. Kira, S. Ishida, and T. Iwamoto, Chem. Rec., 2004, 4, 243; CrossRef M. Weidenbruch, Organometallics, 2003, 22, 4348; CrossRef M. Weidenbruch, J. Organomet. Chem., 2002, 646, 39; CrossRef N. Tokitoh and R. Okazaki, Coord. Chem. Rev., 2000, 210, 251. CrossRef
2. N. Tokitoh, H. Suzuki, R. Okazaki, and K. Ogawa, J. Am. Chem. Soc., 1993, 115, 10428; CrossRef H. Suzuki, N. Tokitoh, R. Okazaki, J. Harada, K. Ogawa, S. Tomoda, and M. Goto, Organometallics, 1995, 14, 1016. CrossRef
3. H. Suzuki, N. Tokitoh, and R. Okazaki, J. Am. Chem. Soc., 1994, 116, 11572; CrossRef H. Suzuki, N. Tokitoh, and R. Okazaki, Bull. Chem. Soc. Jpn., 1995, 68, 2471. CrossRef
4. M. Kira, S. Ishida, T. Iwamoto, and C. Kabuto, J. Am. Chem. Soc., 2002, 124, 3830. CrossRef
5. Experimental procedure and chemical data: The mixture of (Z)-1 (111 mg, 0.0793 mmol) and pyridine (62.8 mg, 0.793 mmol) was stirred in THF at 70 °C for 10 h and the solvent was removed under reduced pressure. Hexane was added to the residue and the solution was filtered. The filtrate was evaporated and recrystallized from hexane/THF to afford 5 (37.5 mg, 0.0241 mmol, 30%) as colorless crystals. 5: mp 241 °C (dec.); 1H NMR (800 MHz, C6D6, 343 K): δ 0.04 (s, 18H), 0.17 (s, 36H), 0.19 (s, 18H), 0.29 (s, 36H), 1.48 (s, 1H), 1.49 (s, 1H), 2.07 (s, 3H), 2.12 (s, 3H), 2.22 (brs, 2H), 2.51 (s, 12H), 2.61 (brs, 2H), 3.18 (ddd, J = 5.4, 3.3, 2.4 Hz, 1H), 3.96 (ddd, J = 10.7, 5.4, 5.1 Hz, 1H), 4.29 (ddd, J = 9.8, 1.4, 0.6 Hz, 1H), 4.58 (ddd, J = 7.2, 5.4, 0.6 Hz, 1H), 4.78 (ddd, J = 10.7, 1.8, 1.4 Hz, 1H), 5.38 (ddd, J = 9.8, 5.4, 1.8 Hz, 1H), 5.81 (dd, J = 16.1, 2.4 Hz, 1H), 5.83 (dd, J = 16.1, 3.3 Hz, 1H), 6.38 (d, J = 7.2 Hz, 1H), 6.60 (brs, 4H), 6.70 (brs, 2H), 6.82 (brs, 2H), 9.32 (d, J = 5.1 Hz, 1H); 13C NMR (200 MHz, C6D6, 343 K): δ 1.19 (q), 1.21 (q), 1.24 (q), 1.26 (q), 2.2 (q), 3.2 (q), 20.8 (q), 20.9 (q), 26.1 (q × 2), 27.8 (d), 27.9 (q × 2), 29.6 (d), 30.1 (d), 31.1 (d), 31.4 (d), 34.1 (d), 58.2 (d), 59.4 (d), 97.5 (d), 115.3 (d), 123.1 (d), 123.9 (d), 128.2 (s), 128.5 (d × 2), 129.4 (s), 129.9 (d × 2), 130.4 (s), 131.2 (d), 133.5 (d), 134.1 (s), 134.9 (s), 136.1 (d), 137.0 (d), 138.6 (d), 138.8 (d), 139.6 (s × 2), 139.7 (s × 2), 144.2 (s), 144.3 (s), 145.5 (s), 147.1 (d), 153.8 (s × 4), 171.7 (d); 29Si NMR (160 MHz, C6D6, 343 K): δ –28.4, –3.0, 1.9, 2.1, 2.5, 2.7; HRMS (APPI-TOF) m/z calcd for C82H151N2Si14 1557.8658 ([M+H]+). Found: 1557.8665 ([M+H]+).
6. X-Ray crystallographic data for [5⋅hexane]: C82H150N2Si14⋅C6H14, M = 1643.47; triclinic; space group P-1 (no. 2); a = 13.5983(9), b = 13.5983(9), c = 20.7806(10) Å; α = 66.2556(19), β = 88.173(3), γ = 84.602(6)°; V = 5036.2(5) Å3; Z = 2; Dcalcd = 1.084 g/cm3; µ = 0.218 mm–1; 2θmax = 50°; T = 103 K; R1 [I > 2σ(I)] = 0.0542; wR2 (all data) = 0.1422; GOF = 1.034 for 17618 reflections and 981 parameters.
7. B. Gehrhus and M. F. Lappert, J. Organomet. Chem., 2001, 617-618, 209. CrossRef
8. N. Takeda, H. Suzuki, N. Tokitoh, R. Okazaki, and S. Nagase, J. Am. Chem. Soc., 1997, 119, 1456. CrossRef
9. N. Tokitoh, H. Suzuki, N. Takeda, T. Kajiwara, T. Sasamori, and R. Okazaki, Silicon Chem., 2002, 1, 313. CrossRef
10. Experimental procedure and chemical data: The mixture of (Z)-1 (90.2 mg, 0.0645 mmol) and N,N-dimethyl-4-aminopyridine (39.3 mg, 0.322 mmol) was stirred in THF at 70 °C for 10 h, and the solvent was removed under reduced pressure. Hexane was added to the residue and the solution was filtered. The filtrate was evaporated and recrystallized from hexane/THF to afford 13 (31.2 mg, 0.0380 mmol, 29% yield) as pale yellow crystals. 13: mp 211 °C (dec.); 1H NMR (300 MHz, C6D6, 298 K): δ 0.12 (s, 9H), 0.16 (s, 9H), 0.25 (s, 18H), 0.37 (s, 9H), 0.40 (s, 9H), 1.55 (s, 1H), 2.02 (s, 3H), 2.12 (s, 6H), 2.62 (s, 6H), 2.90 (s, 1H), 3.18 (s, 1H), 4.82 (d, J = 4.4 Hz, 1H), 6.52 (s, 2H), 6.60 (s, 1H), 6.66 (s, 2H), 6.73 (s, 1H), 8.53 (d, J = 4.4 Hz, 1H); 13C NMR (75 MHz, C6D6, 298 K): δ 1.16 (q), 1.20 (q), 1.5 (q), 1.9 (q), 2.2 (q), 20.9 (q), 27.1 (q × 2), 27.9 (d × 2), 30.8 (d), 39.5 (q × 2), 105.6 (d), 123.5 (d), 128.6 (d), 129.3 (d × 2), 133.6 (s), 136.5 (d), 136.7 (d), 138.8 (s), 144.2 (s), 144.3 (s), 144.5 (s × 2), 153.1 (s), 153.6, (s) 155.6 (s), 162.7 (d); 29Si NMR (60 MHz, C6D6, 298 K): δ –20.8, 2.1, 2.4, 2.9. HRMS (APPI-TOF) m/z calcd for C43H81N2Si7 821.4779 ([M+H]+). Found: 821.4774 ([M+H]+).
11. X-Ray crystallographic data for 13: C43H80N2Si7, M = 821.72; monoclinic; space group C2/c (no. 15); a = 21.3564(3), b = 25.7153(4), c = 19.2493(3) Å; β = 107.7834(8)°; V = 10066.3(3) Å3; Z = 8; Dcalcd = 1.084 g/cm3; µ = 0.219 mm–1; 2θmax = 50°; T = 103 K; R1 [I > 2σ(I)] = 0.0685; wR2 (all data) = 0.1887; GOF = 1.021 for 8867 reflections and 614 parameters.