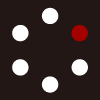
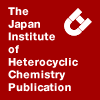
HETEROCYCLES
An International Journal for Reviews and Communications in Heterocyclic ChemistryWeb Edition ISSN: 1881-0942
Published online by The Japan Institute of Heterocyclic Chemistry
e-Journal
Full Text HTML
Received, 30th June, 2011, Accepted, 10th August, 2011, Published online, 15th August, 2011.
DOI: 10.3987/COM-11-S(P)66
■ Catalytic Asymmetric Amination of Oxindoles under Dinuclear Nickel Schiff Base Catalysis
Shinsuke Mouri, Zhihua Chen, Shigeki Matsunaga,* and Masakatsu Shibasaki*
Graduate School of Pharmaceutical Sciences, The University of Tokyo, 7-3-1 Hongo, Bunkyo-ku, Tokyo 113-0033, Japan
Abstract
Catalytic asymmetric synthesis of 3-aminooxindoles with a tetrasubstituted carbon stereocenter is described. 1 mol% of homobimetallic (R)-Ni2-Schiff base complex 1 catalyzed asymmetric amination of 3-alkyl-substituted oxindoles with azodicarboxylates to give products in 89-99% yield and 87-99% ee. For 3-aryl-substituted oxindoles, 10 mol% of (R)-Ni2-Schiff base complex was required to obtain products in 66-98% ee. Postulated catalytic cycle as well as transformation of the products are also described.INTRODUCTION
Oxindoles bearing a tetrasubstituted carbon stereocenter at the 3-position constitute an important structural motif in natural products and biologically active compounds.1 Among them, oxindoles with heteroatoms at the stereogenic center are useful in medicinal chemistry.2 Various methods for catalytic asymmetric synthesis of 3-fluorooxindoles,3 3-chlorooxindoles,4 and 3-hydroxyoxindoles,5,6 as well as their applications to the synthesis of biologically active compounds, have been reported. 3-Aminooxindoles are especially useful units found in therapeutic agents, such as NITD609 for the treatment of malaria, AG-041R, a gastrin/CCK-B receptor agonist, and SSR-149415 for the treatment of stress-related disorders.2 Therefore, development of enantioselective synthetic methods for the structurally diverse sets of chiral 3-aminooxindoles is highly desirable. Several diastereoselective approaches have been developed for synthesizing chiral 3-aminooxindoles using chiral auxiliaries.7 Catalytic asymmetric methods for 3-aminooxindoles, such as Pd-catalyzed asymmetric α-arylation8 and Rh-catalyzed asymmetric intramolecular aza-spiroannulation,9 however, have been limited until very recently. Because catalytic asymmetric benzylic amination of oxindoles provides straightforward access to 3-aminooxindoles, several groups including us have been intensively studied on this topic since 2009.10-12 Both organocatalytic10 and metal-catalyzed amination reactions11,12 have been reported to afford products in high enantioselectivity. We herein describe full substrate scope and limitations of our works on this issue under bimetallic Schiff base 1 catalysis (Figure 1).
RESULTS AND DISCUSSION
As a part of our ongoing research on bimetallic Schiff base catalysis,13-16 we recently developed a homodinuclear Mn2-Schiff base 1 complex and Ni2-Schiff base 1 complex (Figure 1) for the catalytic asymmetric 1,4-addition of 3-substituted-oxindoles to nitroalkenes14 and nitroethylene.15 Therefore, we began our optimization studies using homodinuclear transition metal-1 complexes for reactions of N-Boc 3-methyl-substituted oxindole 3a and azodicarboxylate 4 (Table 1). To select a suitable catalyst for the reaction of oxindole 3a, we screened several metals (entries 1-6), and a homodinuclear Ni2-1 complex gave the best enantioselectivity (entry 6, 97% ee). Toluene was the best solvent among those screened, and 5a was obtained in 99% ee (entry 7). Other solvents, i.e. THF (51% ee), Et2O (61% ee), CH3CN (20% ee), DMSO (1% ee), CHCl3 (75% ee), hexane (30% ee), gave less satisfactory results in terms of enantioselectivity. Catalyst loading was successfully reduced to 1 mol% at 50 °C, still giving 5a in 96% ee after 12 h (entry 8). Furthermore, the amount of 4 was also successfully reduced to 1.2 equiv while maintaining good reactivity and enantioselectivity (entry 9, 99% yield, 99% ee). To check the utility of the bimetallic Ni complex, control experiments were performed in entries 10-14. In entries 10-12, monometallic Ni-salen 2a, 2b, and 2c complexes (Figure 1) prepared from a same chiral source [(R)-1,1’-binaphthyl-2,2’-diamine] were used. The Ni-salen 2a complex with tert-Bu substituents resulted in poor enantioselectivity (entry 10, 13% ee). On the other hand, sterically less hindered monometallic (R)-Ni-2b and (R)-Ni-2c complexes smoothly promoted the reaction, and unexpected reversal of enantiofacial selectivity was observed in comparison with bimetallic (R)-Ni2-1 (entry 9 vs entries 11-12). The enantioselectivity was, however, lower than bimetallic (R)-Ni2-1 [entry 9, (R)-5a, 99% ee vs entries 11-12, (S)-5a, 93-94% ee]. On the other hand, heterobimetallic Pd/Ni/1 and Cu/Ni/1 complexes required 10 mol % catalyst loading for good reactivity, and gave 5a in poor enantioselectivity (entries 13-14).
The substrate scope of the reaction under optimized reaction conditions with homodinuclear (R)-Ni2-1 is summarized in Table 2. The amination of various 3-alkyl-substituted oxindoles 3 with 4 was promoted by 1 mol % of the Ni2-1 catalyst at 50 °C. 3-Methyl, allyl, (E)-cinnamyl, and benzyl substituted oxindoles 3a-3d gave products in high enantioselectivity (entries 1-4, 99-91% ee). 5- or 6-Substituted oxindoles were also applicable. 5-MeO-, 5-F-, 5-Cl-, and 6-Cl-oxindoles (3e-3i) gave products in 99-94% ee (entries 5-9). It is noteworthy that ester and nitrile groups were compatible under the present conditions, and products 5j and 5k were obtained in 96 and 87% ee, respectively (entries 10-11). For 3-aryl-substituted oxindoles, the optimized conditions for 3-alkyl-substituted oxindoles gave poor enantioselectivity (<30% ee) even with 10 mol % catalyst loading, possibly due to background racemic reaction. After re-optimization of the conditions, the best results were obtained in CHCl3 in the presence of molecular sieves 5Å at 30 °C. The role of molecular sieves was not clear, but the addition of molecular sieves had beneficial effects for good reproducibility. The results are summarized in entries 12-19, and moderate to high enantioselectivity, 66-98% ee, was obtained.
We assume that the observed enantiofacial selectivity switch between dinuclear Ni2-1 catalyst and mononuclear (R)-Ni-2b and 2c catalysts (Table 1, entry 9 vs entries 11-12) would be caused by difference in the position of a Ni-enolate intermediate. With dinuclear (R)-Ni2-1, a sterically less hindered Ni-aryloxide in the outer O2O2 cavity would function as a Brønsted base to generate the Ni-enolate in the outer cavity, while a Ni-aryloxide in the N2O2 cavity should generate the Ni-enolate in the case of monometallic (R)-Ni-2b/2c. Because heterobimetallic Pd/Ni/1 and Cu/Ni/1 complexes gave poor enantioselectivity (Table 1, entries 13-14), the Ni metal center in the N2O2 inner cavity of homodinuclear (R)-Ni2-1 is also important for high R-selectivity observed in Table 2, possibly as a Lewis acid to control the orientation of azodicarboxylate 4 from sterically hindered inner cavity. On the other hand, with the monometallic (R)-Ni-2b/2c, azodicarboxylate 4 should come from sterically less hindered outer cite, thus giving S-adduct. Postulated catalytic cycle of the reaction under dinuclear nickel catalysis is shown in Figure 2. One of the Ni-O bonds in the outer O2O2 cavity would work as a Brønsted base to generate Ni-enolate in situ. The other Ni in the inner N2O2 cavity functions as a Lewis acid to control the position of 4, similar to conventional metal-salen Lewis acid catalysis. The C-N bond-formation, followed by protonation, affords product and regenerates the Ni2-1 catalyst.
To demonstrate the synthetic utility of the products, we investigated product transformations (Scheme 1). Three Boc moieties in 5a were readily removed with 4 M HCl in 1,4-dioxane/MeOH at room temperature to give 6a. For the N-N bond cleavage in 6a, Rh/C under H2 atmosphere produced the best results to give 3-aminooxindole 7a in 85% yield (in two steps from 5a). For the N-N bond-cleavage of 6a, the use of Rh/C rather than Pd/C or Raney Ni was essential to suppress undesirable de-amination via the C-N bond cleavage at benzylic position. Removal of the Boc groups in 5j and the N-N bond-cleavage also proceeded smoothly under the similar procedure, giving 3-aminooxindole 7j in 83% yield (in two steps). By treating with isocyanate, 8j was readily obtained from 7j (92% yield), which is a known key intermediate for AG-041R synthesis.9
In summary, we developed a highly enantioselective catalytic asymmetric access to 3-aminooxindoles with a tetrasubstituted carbon stereocenter. A homodinuclear Ni2-Schiff base 1 complex was suitable for catalytic asymmetric amination of 3-substituted oxindoles with di-tert-butyl azodicarboxylate. Reversal of enantiofacial selectivity was observed between bimetallic and monometallic Schiff base complexes, which indicated the plausible role of two metals in dinuclear Ni2-Schiff base 1 catalysis.
EXPERIMENTAL
General: Infrared (IR) spectra were recorded on a JASCO FT/IR 410 Fourier transform infrared spectrophotometer. NMR spectra were recorded on JEOL ECX500 spectrometers, operating at 500 MHz for 1H NMR and 125.65 MHz for 13C NMR. Chemical shifts in CDCl3 were reported in the scale relative to tetramethylsilane (0 ppm) for 1H NMR. For 13C NMR, chemical shifts were reported in the scale relative to CHCl3 (77.0 ppm) as an internal reference. Column chromatography was performed with silica gel Merck 60 (230-400 mesh ASTM). Optical rotations were measured on a JASCO P-1010 polarimeter. ESI mass spectra were measured on Waters micromass ZQ (for LRMS) and ESI mass spectra for HRMS were measured on a JEOL JMS-T100LC AccuTOF spectrometer. The enantiomeric excess (ee) was determined by HPLC analysis. HPLC was performed on JASCO HPLC systems consisting of the following: pump, PU-2080 plus; detector, UV-2075 plus, measured at 254 nm; column, DAICEL CHIRALPAK IA, IA-3, IB or IC; mobile phase, hexane-dichloromethane. 5l, 5m, 5n, 5q, 5r, 5s, 7a and 8j are known compounds. Compounds 3 were synthesized following the literature procedure.3,10 The absolute configuration of 5ja was determined after conversion into known compound, by comparing the sign of optical rotation with the literature data reported by Iwabuchi et al.9 The absolute configuration of other products was assigned by analogy.
(R)-Di-tert-butyl 1-(1-(tert-butoxycarbonyl)-3-methyl-2-oxoindolin-3-yl)hydrazine-1,2-dicarboxylate (5a): To a stirred solution of oxindole 3a (49.4 mg, 0.20 mmol) in toluene (2.0 mL) was added Ni2/Schiff base 1 catalyst (1.3 mg, 2.0 µmol). The resulting mixture was stirred at 50 ºC for 30 min. To the solution was added di-tert-butyl azodicarboxylate 4 (55.3 mg, 0.24 mmol) in one portion. The resulting yellow solution was stirred at 50 ºC for 18 h. The reaction mixture was diluted with Et2O, and the precipitate was removed by filtration through a pad of Celite. The filtrate was concentrated under reduced pressure, and the residue was purified by silica gel flash column chromatography (hexane/EtOAc = 9/1) to give the desired product 5a (94.6 mg, 99% yield) as a colorless solid; IR (KBr) ν 3323, 2980, 2934, 1739, 1609, 1480, 1369 cm-1; 1H NMR (CDCl3) δ 1.14 (s, 9H), 1.52 (s, 3H), 1.64 (s, 9H), 6.66 (s, 1H), 7.20 (m, 1H), 7.29 (m, 1H), 7.80 (d, J = 7.5, 7.9 Hz, 1H), 7.90 (d, J = 6.9 Hz, 1H); 13C NMR (CDCl3) δ 24.3, 27.6, 28.1, 28.2, 65.8, 81.5, 83.0, 84.3, 114.6, 123.7, 125.0, 128.4, 132.7, 138.0, 149.2, 152.9, 156.2, 176.3; HRMS (ESI): m/z calculated for C24H35N3O7Na+ [M+Na]+: 500.2372, found: 500.2376, [α]D22.7 -21.0 (c 1.34, CHCl3); HPLC (DAICEL CHIRALPAK IA, hexane/CH2Cl2 = 1/1, flow 0.5 mL/min, at 40 ºC, detection at 254 nm) tR 9.7 min (S) and 10.6, min (R).
(R)-Di-tert-butyl 1-(1-(tert-butoxycarbonyl)-3-allyl-2-oxoindolin-3-yl)hydrazine-1,2-dicarboxylate (5b): colorless solid; IR (KBr) ν 3322, 2981, 2934, 1778, 1761, 1731, 1713 cm-1; 1H NMR (CDCl3) δ 1.15 (s, 9H), 1.53 (s, 9H), 1.64 (s, 9H), 2.67 (dd, J = 6.3, 13.0 Hz, 1H), 2.74 (dd, J = 8.3, 13.0 Hz, 1H), 4.95 (d, J = 10.3 Hz, 1H), 5.00 (d, J = 17.1 Hz, 1H), 5.21 (m, 1H), 6.67 (s, 1H), 7.20 (dd, J = 7.5, 7.5 Hz, 1 H), 7.30 (dd, J = 7.5, 8.0 Hz, 1H), 7.75 (d, J = 8.0 Hz,, 1H), 7.87 (d, J = 7.5 Hz, 1H); 13C NMR (CDCl3) δ 27.6, 28.0, 28.2, 41.5, 68.7, 81.5, 83.0, 84.1, 114.4, 120.7, 124.1, 124.8, 128.6, 129.5, 129.8, 138.9, 149.0, 152.9, 156.2, 175.3; HRMS (ESI): m/z calculated for C26H37N3O7Na+ [M+Na]+: 526.2529, found: 526.2531; [α]D22.5 -9.3 (c 0.60, CHCl3), HPLC (DAICEL CHIRALPAK IA, hexane/CH2Cl2 = 3/7, flow 0.5 mL/min, at 40 ºC, detection at 254 nm) tR 16.7 min (R) and 19.6 min (S).
(R,E)-Di-tert-butyl 1-(1-(tert-butoxycarbonyl)-3-cinnamyl-2-oxoindolin-3-yl)hydrazine-1,2-dicarboxylate (5c): colorless solid; IR (KBr) 3348, 2979, 1792, 1778, 1735, 1709 cm-1; 1H NMR (CDCl3) δ 1.16 (s, 9H), 1.47 (s, 9H), 1.59 (s, 9H), 2.84-2.85 (m, 2H), 5.56-5.59 (m,1H), 6.30 (d, J = 16.0 Hz, 1H), 6.73 (s, 1H), 7.08-7.31 (m, 7H), 7.70 (d, J = 8.1 Hz, 1H), 7.92 (d, J = 7.5 Hz, 1H); 13C NMR (CDCl3) δ 27.9, 28.1, 28.2, 40.5, 69.2, 81.6, 83.1, 84.0, 114.4, 120.9, 124.1, 124.9, 126.2, 127.4, 128.3, 128.7, 129.8, 135.5, 136.9, 138.7, 148.7, 153.0, 156.2, 175.5 ; HRMS (ESI): m/z calculated for C32H41N3O7Na+ [M+Na]+: 602.2842, found: 602.2842; [α]D22.5 -24.4 (c 0.50, CHCl3), HPLC (DAICEL CHIRALPAK IA, hexane/CH2Cl2 = 7/3, flow 0.5 mL/min, at 40 ºC, detection at 254 nm) tR 23.2 min (S) and 29.5 min (R).
(R)-Di-tert-butyl 1-(3-benzyl-1-(tert-butoxycarbonyl)-2-oxoindolin-3-yl)hydrazine-1,2-dicarboxylate (5d): colorless solid; IR (KBr) ν 2980, 2394, 2648, 2360, 2340, 1734 cm-1; 1H NMR (CDCl3) 1.14 (s, 9H), 1.47 (s, 9H), 1.62 (s, 9H), 3.10 (d, J = 12.3 Hz, 1H), 3.40 (d, J = 12.3 Hz, 1H), 6.63-6.66 (m, 2H), 6.77 (s, 1H), 6.95-6.99 (m, 2H), 7.03-7.07 (m, 1H), 7.16-7.23 (m, 2H), 7.35 (d, J = 7.4 Hz, 1H), 8.03 (d, J = 6.9 Hz, 1H); 13C NMR (CDCl3) 27.7, 28.0, 28.3, 43.3, 70.0, 81.6, 83.1, 83.5, 114.1, 124.1, 124.6, 127.0, 127.5, 128.6, 129.6, 130.2, 132.3, 139.0, 148.2, 152.9, 156.4, 175.3; HRMS (ESI): m/z calculated for C25H37N3O7Na+ [M+Na]+: 576.2686, found: 576.2671; [α]D19.0 -2.3 (c 1.5, CHCl3), HPLC (DAICEL CHIRALPAK IA, hexane/CH2Cl2 = 7/3, flow 0.5 mL/min, at 40 ºC, detection at 254 nm) tR 11.6 min (S) and 20.3 min (R).
(R)-Di-tert-butyl 1-(1-(tert-butoxycarbonyl)-5-methoxy-3-methyl-2-oxoindolin-3-yl)hydrazine-1,2-dicarboxylate (5e): colorless solid; IR (KBr) ν 3300, 2980, 2934, 1731, 1601 cm-1; 1H NMR (CDCl3) 1.17 (s, 9H), 1.47 (s, 3H), 1.63 (s, 9H), 3.83 (s, 3H), 6.66 (s, 1H), 6.81 (dd, J = 2.9, 8.6 Hz, 1H), 7.59 (brs, 1H), 7.70 (d, J = 8.6 Hz, 1H;13C NMR (CDCl3) 24.5, 27.7, 28.1, 28.2, 55.7, 66.1, 81.3, 83.0, 84.1, 109.7, 113.5, 115.6, 131.2, 133.6, 149.3, 152.9, 156.1, 157.3, 176.3; HRMS (ESI): m/z calculated for C24H35N3O7Na+ [M+Na]+: 530.2478, found: 530.2480; [α]D22.5 -5.1 (c 3.1, CHCl3), HPLC (DAICEL CHIRALPAK IC, hexane/CH2Cl2 = 1/1, flow 0.5 mL/min, at 40 ºC, detection at 254 nm) tR 50.8 min (S) and 89.5 min (R).
(R)-Di-tert-butyl 1-(1-(tert-butoxycarbonyl)-5-fluoro-3-methyl-2-oxoindolin-3-yl)hydrazine-1,2-dicarboxylate (5f): colorless foam; IR (neat) ν 3323, 2981, 2934, 1782, 1752, 1731, 1705, 1611 cm-1; 1H NMR (CDCl3) 1.18 (s, 9H), 1.47 (s, 3H), 1.60 (s, 9H), 1.64 (s, 9H), 6.61 (s, 1H), 6.99 (ddd, J= 2.9, 8.9, 8.9 Hz, 1H), 7.72 (brs, 1H), 7.79 (dd, J = 4.6, 8.9 Hz, 1H); 13C NMR (CDCl3) 24.3, 27.7, 28.1, 28.2, 65.8, 81.7, 83.2, 84.5, 111.4 (d, 2J C-F = 23.9 Hz), 114.9 (d, 2J C-F = 23.9 Hz), 116.0 (d, 3J C-F = 8.4 Hz), 133.8, 133.9, 149.2, 152.8, 156.2, 160.3 (d, 1J C-F = 245 Hz), 175.9; HRMS (ESI): m/z calculated for C24H34FN3O7Na+ [M+Na]+: 518.2279, found: 518.2276; [α]D22.5 -35.0 (c 0.70, CHCl3) HPLC (Combined use of three comlums DAICEL CHIRALPAK IA, IA-3, and IB, hexane/CH2Cl2 = 3/7, flow 0.5 mL/min, at 40 ºC, detection at 254 nm) tR 53.9 min (S) and 58.2 min (R).
(R,E)-Di-tert-butyl 1-(3-allyl-1-(tert-butoxycarbonyl)-5-fluoro-2-oxoindolin-3-yl)hydrazine-1,2-dicarboxylate (5g): colorless foam; IR (KBr) ν 3324, 2981, 2935, 1735 cm-1; 1H NMR (CDCl3) δ 1.19 (s, 9H), 1.47 (s, 9H), 1.61 (s, 9H), 2.61 (dd, J = 6.9, 13.2 Hz, 1H), 2.73 (dd, J = 8.4, 13.42 Hz, 1H), 4.97 (d, J = 10.3 Hz, 1H), 5.01 (d, J = 17.2 Hz, 1H), 5.17-5.25 (m, 1H), 6.69 (s, 1H), 6.99 (d, J = 2.9, 9.2, 9.2 Hz, 1H), 7.72 (brd, J = 5.8 Hz, 1H), 7.75 (dd, J = 4.6, 9.2 Hz, 1H); 13C NMR (CDCl3) 27.6, 28.0, 28.2, 41.4, 68.7, 81.7, 83.3, 84.3, 111.8 (d, 2J C-F = 25.1 Hz), 115.0 (d, 2J C-F = 22.8 Hz), 115.7 (d, 3J C-F = 8.4 Hz), 121.0, 129.1, 131.7 (d, 3J C-F = 7.2 Hz), 134.8 (d, 4J C-F = 2.4 Hz), 148.9, 152.8, 156.2, 160.2 (d, 1J C-F = 245 Hz), 174.9; HRMS (ESI): m/z calculated for C26H36FN3O7Na+ [M+Na]+: 544.2435, found: 544.2442; [α]D21.0 -12.8 (c 0.70, CHCl3), HPLC (DAICEL CHIRALPAK IA, hexane/CH2Cl2 = 3/7, flow 0.5 mL/min, at 40 ºC, detection at 254 nm) tR 20.9 min (R) and 25.4 min (S).
(R)-Di-tert-butyl 1-(3-allyl-1-(tert-butoxycarbonyl)-5-chloro-2-oxoindolin-3-yl)hydrazine-1,2-dicarboxylate (5h): colorless solid; IR (KBr) ν 3324, 2980, 2934, 1787, 1748, 1733, 1709 cm-1; 1H NMR (CDCl3) δ 1.19 (s, 9H), 1.53 (s, 9H), 1.63 (s, 9H), 2.63 (dd, J = 7.0, 13.4 Hz, 1H), 2.72 (dd, J = 8.0, 13.4 Hz, 1H), 4.97 (brd, J = 9.7 Hz, 1H), 5.01 (brd, J = 17.2 Hz, 1H), 5.16-5.25 (m, 1H), 6.60 (s, 1H), 7.28 (d, J = 9.2 Hz, 1H), 7.72 (d, J = 9.2 Hz, 1H), 7.89 (s, 1H); 13C NMR (CDCl3) δ 27.7, 28.0, 28.1, 41.4, 68.6, 81.7, 83.3, 84.4, 115.7, 121.2, 124.3, 128.7, 129.0, 130.3, 131.6, 137.5, 148.8, 152.8, 156.1, 174.9; HRMS (ESI): m/z calculated for C26H36ClN3O7Na+ [M+Na]+: 560.2140, found: 560.2151; [α]D22.5 -27.3 (c 1.40, CHCl3), HPLC (DAICEL CHIRALPAK IB, hexane/CH2Cl2 = 9/1, flow 0.5 mL/min, at 40 ºC, detection at 254 nm) tR 39.6 min (S) and 51.7 min (R).
(R)-Di-tert-butyl 1-(3-benzyl-1-(tert-butoxycarbonyl)-6-chloro-2-oxoindolin-3-yl)hydrazine-1,2-dicarboxylate (5i): colorless solid; IR (KBr) ν 3325, 2980, 2935, 1787, 1733 1709 cm-1; 1H NMR (CDCl3)1.19 (s, 9H), 1.50 (s, 9H), 1.74 (s, 9H), 3.06 (d, J=12.4 Hz, 1H), 3.37 (d, J=12.4 Hz, 1H), 6.66 (d, J=7.4Hz, 2H), 6.74 (s, 1H), 7.01(dd, J=7.4, 7.6 Hz, 2H), 7.09 (dd, J=7.4, 7.5Hz, 1H), 7.20 (dd, J=1.4, 8.8 Hz, 1H), 7.44(d, J=1.4 Hz, 1H), 7.99 (d, J=8.8 Hz, 1H); 13C NMR (CDCl3) 27.7, 28.0, 28.2, 43.1, 69.6, 81.9, 83.7, 84.1, 114.9, 124.6, 125.1, 127.2, 127.7, 128.1, 130.2, 131.9, 134.2, 139.9, 147.9, 152.8, 156.4, 174.7; HRMS (ESI): m/z calculated for C30H38N3O7ClNa+ [M+Na]+: 610.2296, found: 610.2288; [α]D19.0 -32.3 (c 2.5, CHCl3), HPLC (DAICEL CHIRALPAK IA, hexane/CH2Cl2 = 1/1, flow 0.5 mL/min, at 40 ºC, detection at 254 nm) tR 7.8 min (R) and 10.5 min (S).
(R)-Di-tert-butyl 1-(1-(tert-butoxycarbonyl)-3-(2-methoxy-2-oxoethyl)-2-oxoindolin-3-yl)hydrazine-1,2-dicarboxylate (5j): colorless solid; IR (KBr) ν 3313, 2981, 1715, 1608, 1481, 1372 cm-1; 1H NMR (CDCl3) δ 1.17 (s, 9H), 1.56 (s, 9H), 1.67 (s, 9H), 2.98 (d, J = 14.6 Hz, 1H), 3.18 (d, J = 14.6 Hz, 1H), 3.44 (s, 3H), 6.71 (s, 1H), 7.19 (dd, J = 7.5, 7.5 Hz, 1H), 7.31 (dd, J = 7.5, 8.0 Hz, 1H), 7.80 (d, J = 8.0 Hz, 1H), 7.80 (d, J = 7.5 Hz, 1H); 13C NMR (CDCl3) 27.7, 28.1, 28.2, 42.2, 51.9, 66.2, 88.8, 83.4, 84.1, 114.6, 124.5, 124.7, 128.3, 129.3, 139.7, 149.1, 152.6, 156.1, 168.1, 174.5; HRMS (ESI): m/z calculated for C26H37N3O7Na+ [M+Na]+: 558.2428, found: 558.2432; [α]D22.0 -3.4 (c 1.20, CHCl3), HPLC (DAICEL CHIRALPAK IA, hexane/CH2Cl2 = 3/7, flow 0.5 mL/min, at 40 ºC, detection at 254 nm) tR 22.8 min (S) and 26.5 min (R).
(R)-Di-tert-butyl 1-(1-(tert-butoxycarbonyl)-3-(cyanomethyl)-2-oxoindolin-3-yl)hydrazine-1,2-dicarboxylate (5k): yellow solid; IR (KBr) ν3324, 2981, 2934, 1800, 1778, 1735, 1713, 1608 cm-1; 1H NMR (CDCl3) δ 1.19 (s, 9H), 1.55 (s, 9H), 1.67 (s, 9H), 2.83 (d, J = 16.6 Hz, 1H), 2.94 (d, J = 16.6 Hz, 1H), 6.73 (s, 1H), 7.27 (dd, J = 7.5, 7.5 Hz, 1H), 7.40 (dd, J = 7.5, 8.1 Hz, 1H), 7.85 (d, J = 8.1 Hz, 1H), 8.01 (d, J = 7.5 Hz, 1H); 13C NMR (CDCl3) 26.7, 27.6, 28.0, 28.2, 65.4, 82.5, 83.9, 85.0, 114.4, 115.0, 124.8, 125.4, 127.2, 130.0, 138.6, 148.7, 152.1, 156.1, 172.7; HRMS (ESI): m/z calculated for C25H34N4O7Na+ [M+Na]+: 525.2325, found: 525.2334; [α]D22.5 -67.1 (c 0.80, CHCl3); HPLC (DAICEL CHIRALPAK IA, hexane/CH2Cl2 = 7/3, flow 0.5 mL/min, detection at 254 nm) tR 16.8 min (S) and 34.0 min (R).
(S)-Di-tert-butyl 1-(1-(tert-butoxycarbonyl)-3-(2-methoxyphenyl)-2-oxoindolin-3-yl)hydrazine-1,2-dicarboxylate (5o): colorless solid; IR (KBr) ν 3391, 2979, 2929, 2359, 2320, 1801, 1731 cm-1; 1H NMR (CDCl3) 1.20 (s, 9H), 1.35 (s, 9H), 1.67 (s, 9H), 3.86 (s, 3H), 6.27 (s, 1H), 6.83-6.85 (m, 1H), 6.92-6.96 (m, 2H), 7.14-7.19(m, 2H), 7.24 (m, 2H), 7.45(d, J= 8.1 Hz, 1H) ; 13C NMR (CDCl3) 27.7, 28.0, 28.1, 56.5, 72.8, 80.6, 82.8, 83.8, 113.3, 114.5, 121.1, 124.4, 125.0, 126.0, 128.6, 129.0, 129.5, 130.0, 138.6, 149.3, 153.8, 155.0, 158.0, 173.1; ESI-MS m/z 592.1 [M+Na] calculated for C30H39N3O8Na+ [M+Na]+: 592.2635, found: 592.2616; [α]D22.1 +54.2 (c 3.61, CHCl3); HPLC (DAICEL CHIRALPAK IA, hexane/CH2Cl2 = 1/1, flow 1.0 mL/min, detection at 254 nm) tR 3.9 min (minor) and 4.3 min (major).
(R)-Di-tert-butyl 1-(1-(tert-butoxycarbonyl)-5-methyl-2-oxo-3-phenylindolin-3-yl)hydrazine-1,2-dicarboxylate (5p): colorless solid; IR (KBr) ν 3445, 2977, 2963, 2329, 1733 cm-1; 1H NMR (CDCl3) 1.20 (s, 9H), 1.29 (s, 9H), 1.60 (s, 9H), 2.45 (s, 3H), 6.29 (s, 1H), 7.12-7.14 (m, 1H), 7.27-7.30 (m, 2H), 7.35-7.36 (m, 1H), 7.54-7.55(m, 2H), 7.81 (d, J=8.6 Hz, 1H), 7.98(s, 1H); 13C NMR (CDCl3) 27.7, 28.0, 28.8, 72.7, 80.8, 83.1, 84.1, 114.6, 125.4, 128.0, 128.6, 130.0, 130.1, 133.3, 134.1, 136.4, 149.1, 153.3, 154.7, 174.4; HRMS (ESI): m/z calculated for C30H39N3O7Na+ [M+Na]+: 576.2686, found: 576.2660; [α]D22.1 -79.4 (c 1.04, CHCl3); HPLC (DAICEL CHIRALPAK IA3, hexane/CH2Cl2 = 1/1, flow 0.5 mL/min, detection at 254 nm) tR 8.3 min (major) and 13.6 min (minor).
(R)-3-Amino-3-methyl-1,3-dihydro-indol-2-one (7a): To a 4 M HCl aq. solution in 1,4-dioxane (1.5 mL) at room temperature was added 5a (72 mg, 0.15 mmol). The reaction mixture was stirred for 2 h at room temperature. The reaction mixture was evaporated to give crude 6a, which was used for the next step without purification. To a test tube with the crude 6a was added MeOH (1.5 mL) and Rh/C (36 mg). The reaction was stirred for 5 h at rt under H2 (1 atm), then was filtered through a filter paper and washed with MeOH. The filtrate was concentrated under reduced pressure to give a pale yellow solid. The residue was purified by silica gel flash column chromatography (5% MeOH in AcOEt) to afford 7a (20.7 mg, 0.128 mmol, 85% yield) as a colorless solid; IR (KBr) ν 3139, 1710, 1675, 1620, 1472 cm-1; 1H NMR (CD3OD) δ 1.42 (s, 3H), 6.90 (d, J = 8.0 Hz, 1H), 7.04 (ddd, J = 8.0, 8.0, 1.3 Hz, 1H), 7.22 (ddd, J = 8.0, 8.0, 1.3 Hz, 1H), 7.37 (dd, J = 8.0, 1.3 Hz, 1H); 13C NMR (CD3OD) δ 25.6, 59.1, 111.2, 123.7, 124.5, 129.9, 135.1, 142.1, 184.4; ESI-MS m/z 185 [M+Na]+; [α]D30.3 +10.7 (c 0.60, CH3OH).
(R)-(3-Amino-2-oxo-2,3-dihydro-1H-indol-3-yl)-acetic acid methyl ester (7j): To a solution of 3 M HCl in MeOH/1,4-dioxane = 1:1 (6 mL) at room temperature was added 5j (214 mg, 0.40 mmol). The reaction mixture was stirred for 2 h at room temperature. The reaction mixture was evaporated to give crude 6j without Boc groups as a colorless solid, which was used for the next step without purification. To a test tube with the crude 6j were added MeOH (4 mL) and Rh/C (100 mg). The reaction mixture was stirred for 6 h at room temperature under H2 (1 atm), then filtered through a filter paper and washed with MeOH. The filtrate was concentrated under reduced pressure to give a pale yellow oil. The residue was purified by silica gel flash column chromatography (2.5% MeOH in AcOEt) to afford 7j (73 mg, 0.33 mmol, 83% yield) as a colorless oil; IR (neat) ν 1734, 1623, 1474, 1214, 755 cm-1; 1H NMR (CD3OD) δ 2.92 (d, J = 16.1 Hz, 1H), 2.96 (d, J = 16.1 Hz, 1H), 3.50 (s, 3H), 6.91 (d, J = 8.0 Hz, 1H), 7.02 (dd, J = 8.0, 8.0 Hz, 1H), 7.24 (dd, J = 8.0, 8.0 Hz, 1H), 7.35 (d, J = 8.0 Hz, 1H); 13C NMR (CD3OD) δ 43.0, 59.9, 111.3, 123.5, 124.8, 130.4, 132.6, 143.2, 171.4, 182.3; HRMS (ESI): m/z calculated for C11H12N2O3Na+ [M+Na]+: 243.0746, found: 243.0737; [α]D27.7 +31.0 (c 0.60, CH3OH).
(R)-Methyl 2-(2-oxo-3-(3-p-tolylureido)indolin-3-yl)acetate (8j): p-Tolyl isocyanate (8.0 mg, 0.06 mmol) was added to the mixture of 7j (11.0 mg, 0.05 mmol) in MeCN. The reaction mixture was stirred for 2 h at room temperature. The reaction mixture was evaporated and the residue was purified by silica gel flash column chromatography (AcOEt:hexane = 2:1) to afford 8j (16.3 mg, 0.046 mmol, 92% yield) as a colorless solid; IR (KBr) ν 3338, 1728, 1662, 1604, 1549, 1212, 753 cm-1; 1H NMR (CDCl3) δ 2.26 (s, 3H), 2.60 (d, J = 15.3 Hz, 1H), 2.90 (d, J = 15.3 Hz, 1H), 3.67 (s, 3H), 6.47 (br, 1H), 6.75 (s, 1H), 6.83 (d, J = 7.6 Hz, 1H), 6.99 (dd, J = 7.6, 7.6 Hz, 1H), 7.05 (d, J = 8.3 Hz, 1H), 7.12 (d, J = 8.3 Hz, 1H), 7.18-7.22 (m, 2H), 7.63 (br, 1H); 13C NMR (CDCl3) δ 20.7, 40.5, 52.1, 59.6, 110.7, 120.7, 122.6, 123.0, 129.1, 129.5, 130.1, 133.1, 135.6, 140.6, 154.3, 170.7, 178.0; ESI-MS m/z 376 [M+Na]+; HRMS (ESI): m/z calculated for C24H34ClN3O7Na+ [M+Na]+: 376.1273, found: 376.1263; [α]D30.3 +12.0 (c 0.80, CHCl3).
ACKNOWLEDGEMENTS
This work was supported by Grant-in-aid for Young Scientist (A) from JSPS, Scientific Research on Innovative Areas “Molecular Activation Directed toward Straightforward Synthesis”, the Uehara Memorial Foundation, and the Noguchi Institute. S.M. and S.M. thank prof. Kanai at the University of Tokyo for his fruitful discussion and unlimited access to analytical facilities in his lab. We thank Mr. Mitsunuma and Mr. Furutachi for the supply of starting materials.
References
1. Reviews: A. B. Dounay and L. E. Overman, Chem. Rev., 2003, 103, 2945; CrossRef C. V. Galliford and K. A. Scheidt, Angew. Chem. Int. Ed., 2007, 46, 8748. CrossRef
2. 3-Aminooxindoles in medicinal chemistry: M. Rottmann, C. McNamara, B. K. S. Yeung, M. C. S. Lee, B. Zou, B. Russell, P. Seitz, D. M. Plouffe, N. V. Dharia, J. Tan, S. B. Cohen, K. R. Spencer, G. E. González-Páez, S. B. Lakshminarayana, A. Goh, R. Suwanarusk, T. Jegla, E. K. Schmitt, H.-P. Beck, R. Brun, F. Nosten, L. Renia, V. Dartois, T. H. Keller, D. A. Fidock, E. A. Winzeler, and T. T. Diagana, Science, 2010, 329, 1175; CrossRef M. Ochi, K. Kawasaki, H. Kataoka, and Y. Uchio, Biochem. Biophys. Res. Commun., 2001, 283, 1118; CrossRef K. Bernard, S. Bogliolo, and J. Ehrenfeld, Br. J. Pharmacol., 2005, 144, 1037; CrossRef G. Gilles and S. L. Claudine, Stress, 2003, 6, 199. CrossRef
3. Y. Hamashima, T. Suzuki, H. Takano, Y. Shimura, and M. Sodeoka, J. Am. Chem. Soc., 2005, 127, 10164; CrossRef N. Shibata, J. Kohno, K. Takai, T. Ishimaru, S. Nakamura, T. Toru, and S. Kanemasa, Angew. Chem. Int. Ed., 2005, 44, 4204; CrossRef T. Ishimaru, N. Shibata, T. Horikawa, N. Yasuda, S. Nakamura, T. Toru, and M. Shiro, Angew. Chem. Int. Ed., 2008, 47, 4157. CrossRef
4. W. Zheng, Z. Zhang, M. J. Kaplan, and J. C. Antilla, J. Am. Chem. Soc., 2011, 133, 3339; CrossRef M.-X. Zhao, Z.-W. Zhang, M.-X. Chen, W.-H. Tang, and M. Shi, Eur. J. Org. Chem., 2011, 3001. CrossRef
5. Hydroxylation of oxindoles: T. Ishimaru, N. Shibata, J. Nagai, S. Nakamura, T. Toru, and S. Kanemasa, J. Am. Chem. Soc., 2006, 128, 16488; CrossRef D. Sano, K. Nagata, and T. Itoh, Org. Lett., 2008, 10, 1593; CrossRef T. Bui, N. R. Candeias, and C. F. Barbas, III, J. Am. Chem. Soc., 2010, 132, 5574. CrossRef
6. Selected leading examples of nucleophilic addition to isatins: R. Shintani, M. Inoue, and T. Hayashi, Angew. Chem. Int. Ed., 2006, 45, 3353; CrossRef P. Y. Toullec, R. B. C. Jagt, J. G. de Vries, B. L. Feringa, and A. J. Minnaard, Org. Lett., 2006, 8, 2715; CrossRef A. V. Malkov, M. A. Kabeshov, M. Bella, O. Kysilka, D. A. Malyshev, K. Pluhácková, and P. Kocovsky, Org. Lett., 2007, 9, 5473; CrossRef H. Lai, Z. Huang, Q. Wu, and Y. Qin, J. Org. Chem., 2009, 74, 283; CrossRef S. Nakamura, N. Hara, H. Nakashima, K. Kubo, N. Shibata, and T. Toru, Chem. Eur. J., 2008, 14, 8079; CrossRef D. Tomita, K. Yamatsugu, M. Kanai, and M. Shibasaki, J. Am. Chem. Soc., 2009, 131, 6946; CrossRef J. Itoh, S. B. Han, and M. J. Krische, Angew. Chem. Int. Ed., 2009, 48, 6313; CrossRef T. Itoh, H. Ishikawa, and Y. Hayashi, Org. Lett., 2009, 11, 3854 and references therein. See also, reference 8. CrossRef
7. For diastereoselective approaches using chiral auxiliaries: T. Emura, T. Esaki, K. Tachibana, and M. Shimizu, J. Org. Chem., 2006, 71, 8559; CrossRef G. Lesma, N. Landoni, T. Pilati, A. Sacchetti, and A. Silvani, J. Org. Chem., 2009, 74, 4537. CrossRef
8. Y.-X. Jia, J. M. Hillgren, E. L. Watson, S. P. Marsden, and E. P. Kündig, Chem. Commun., 2008, 4040. CrossRef
9. S. Sato, M. Shibuya, N. Kanoh, and Y. Iwabuchi, Chem. Commun., 2009, 6264; CrossRef S. Sato, M. Shibuya, N. Kanoh, and Y. Iwabuchi, J. Org. Chem., 2009, 74, 7522. CrossRef
10. L. Cheng, L. Liu, D. Wang, and Y.-J. Chen, Org. Lett., 2009, 11, 3874; CrossRef Z.-Q. Qian, F. Zhou, T.-P. Du, B.-L. Wang, M. Ding, X.-L. Zhao, and J. Zhou, Chem. Commun., 2009, 6753; CrossRef T. Bui, M. Borregan, and C. F. Barbas, III, J. Org. Chem., 2009, 74, 4537; CrossRef T. Bui, G. Hernández-Torres, C. Milite, and C. F. Barbas, III, Org. Lett., 2010, 12, 5696; CrossRef T. Zhang, L. Cheng, L. Liu, D. Wang, and Y.-J. Chen, Tetrahedron: Asymmetry, 2010, 21, 2800. CrossRef
11. A part of results in this article was communicated previously, see: S. Mouri, Z. Chen, H. Mitsunuma, M. Furutachi, S. Matsunaga, and M. Shibasaki, J. Am. Chem. Soc., 2010, 132, 1255. CrossRef
12. Z. Yang, Z. Wang, S. Bai, K. Shen, D. Chen, X. Liu, L. Lin, and X. Feng, Chem. Eur. J., 2010, 16, 6632; CrossRef K. Shen, X. Liu, G. Wang, L. Lin, and X. Feng, Angew. Chem. Int. Ed., 2011, 50, 4684.
13. For selected examples, see: S. Handa, V. Gnanadesikan, S. Matsunaga, and M. Shibasaki, J. Am. Chem. Soc., 2007, 129, 4900; CrossRef S. Handa, K. Nagawa, Y. Sohtome, Y. S. Matsunaga, and M. Shibasaki, Angew. Chem. Int. Ed., 2008, 47, 3230; CrossRef H. Mihara, Y. Xu, N. E. Shepherd, S. Matsunaga, and M. Shibasaki, J. Am. Chem. Soc., 2009, 131, 8384; CrossRef Z. Chen, M. Furutachi, Y. Kato, S. Matsunaga, and M. Shibasaki, Angew. Chem. Int. Ed., 2009, 48, 2218; CrossRef S. Handa, V. Gnanadesikan, S. Matsunaga, and M. Shibasaki, J. Am. Chem. Soc., 2010, 132, 4925; CrossRef Y. Xu, L. Lin, M. Kanai, S. Matsunaga, and M. Shibasaki, J. Am. Chem. Soc., 2011, 133, 5791; CrossRef G. Lu, T. Yoshino, H. Morimoto, S. Matsunaga, and M. Shibasaki, Angew. Chem. Int. Ed., 2011, 50, 4382. CrossRef
14. Y. Kato, M. Furutachi, Z. Chen, H. Mitsunuma, S. Matsunaga, and M. Shibasaki, J. Am. Chem. Soc., 2009, 131, 9168. CrossRef
15. H. Mitsunuma and S. Matsunaga, Chem. Commun., 2011, 469; CrossRef For the utility of Ni2-catalyst in other reactions, see: Z. Chen, H. Morimoto, S. Matsunaga, and M. Shibasaki, J. Am. Chem. Soc. 2008, 130, 2170; CrossRef Y. Xu, G. Lu, S. Matsunaga, and M. Shibasaki, Angew. Chem. Int. Ed., 2009, 48, 3353; CrossRef S. Mouri, Z. Chen, S. Matsunaga, and M. Shibasaki, Chem. Commun., 2009, 5138; CrossRef N. E. Shepherd, H. Tanabe, Y. Xu, S. Matsunaga, and M. Shibasaki, J. Am. Chem. Soc., 2010, 132, 3666. CrossRef
16. For selected examples of related bifunctional bimetallic Schiff base complexes in asymmetric catalysis, see V. Annamalai, E. F. DiMauro, P. J. Carroll, and M. C. Kozlowski, J. Org. Chem., 2003, 68, 1973 and references therein; CrossRef M. Yang, C. Zhu, F. Yuan, Y. Huang, and Y. Pan, Org. Lett., 2005, 7, 1927; CrossRef W. Hirahata, R. M. Thomas, E. B. Lobkovsky, and G. W. Coates, J. Am. Chem. Soc., 2008, 130, 17658; CrossRef C. Mazet and E. N. Jacobsen, Angew. Chem. Int. Ed., 2008, 47, 1762; CrossRef B. Wu, J. C. Gallucci, J. R. Parquette, and T. V. RajanBabu, Angew. Chem. Int. Ed., 2009, 48, 1126; CrossRef B. Wu, J. R. Parquette, and T. V. RajanBabu, Science, 2009, 326, 1662. CrossRef
17. In our previous studies on direct Mannich-type reactions and Michael reactions using Ni2-1, Co2-1 and Mn2-1 complexes, corresponding monometallic Ni-, Co-, and Mn-2a, 2b, and 2c complexes resulted in poor to modest reactivity and enantioselectivity. See, references 14 and 15.