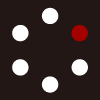
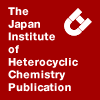
HETEROCYCLES
An International Journal for Reviews and Communications in Heterocyclic ChemistryWeb Edition ISSN: 1881-0942
Published online by The Japan Institute of Heterocyclic Chemistry
e-Journal
Full Text HTML
Received, 28th April, 2011, Accepted, 31st May, 2011, Published online, 13th June, 2011.
DOI: 10.3987/COM-11-S(P)16
■ Synthetic Approach to N,N,N',N'-Tetrakis[(2-pyridylmethyl)ethylenediamine] (TPEN) Derivatives through Ruthenium-Catalyzed-[2+2+2]cycloaddition
Yuji Miyazaki, Fujimaru Tanaka, Kenji Takeshita, and Atsunori Mori*
Department of Chemical Science and Engineering, Faculty of Engineering, Kobe University, Rokkodai-cho, Nada-ku, Kobe 657-8501, Japan
Abstract
Hydrophobic TPEN (N,N,N',N'-tetrakispyridylmethyl-1,2-ethylenediamine) derivatives are prepared by [2+2+2]cycloaddition of 1,ω-diyne and bromoacetonitrile leading to bromomethylpyridine and following reaction of 1,2-ethylenediamine. The obtained TPEN derivatives are employed for extraction of a soft metal ion such as cadmium(II).INTRODUCTION
TPEN, N,N,N',N'-tetrakis[(2-pyridylmethyl)-1,2-ethylenediamine], which is composed of four 2-pyridylmethyl groups on 1,2-ethylenediamine, recently attracts much attention as a multidentate ligand with six nitrogen donors for coordination of various metal ions.1 Although it is potentially available as an agent for solvent extraction, the major drawback of TPEN is solubility to water and easy protonating characteristics under acidic conditions. A solution to this problem is the introduction of hydrophobic substituents onto the TPEN structure. We have shown that several hydrophobic TPEN derivatives are available as an effective extraction agent for soft metal ions and several minor actinides (MA),2 which are considerably important for the separation process of MA from lanthanides in the treatment of high level atomic waste (HLW) produced in the nuclear power plant.3 Synthesis of such TPEN derivatives has been performed with several steps to obtain substituted halomethylpyridine that is a precursor of the reaction of 1,2-ethylenediamine.2 Accordingly, design and synthesis of novel hydrophobic TPEN derivatives, if it can be prepared in a short and straightforward sequence, are highly intriguing.
On the other hand, transition metal-catalyzed [2+2+2]cycloaddition with three triple bonds leading to the formation of aromatic ring is extensively studied and several metal catalysts are shown to be effective.4 It was also shown that pyridine derivatives are obtained by the reaction of two alkynes and a nitrile through the [2+2+2]cycloaddition.5 We envisaged that the reaction would be available to the formation of hydrophobic TPEN derivatives. Herein, we report synthesis of a new class of TPEN derivatives of highly hydrophobic characteristics, which involve high performance in the solvent extraction of soft metal cadmium inos.
RESULTS AND DISCUSSION
We performed the preparation of 2-bromomethylpyridine with 1, ω-diyne and bromoacetonitrile as described in the literature.5c-e The reaction of bromoacetonitrile (1) with 1,6-heptadiyne (2a) in the presence of 10 mol% of Cp*Ru(cod)Cl (3) as a catalyst lead to the formation of 2-bromomethylpyridine 4a in 57% yield. Although the reaction proceeded smoothly, it was found to be important to add diyne 2 slowly, otherwise, undesirable side product 5 was preferentially formed. Another bromomethylpyridine derivative was also synthesized in a similar manner6 to afford the corresponding 4b in 68% yield. The obtained pyridine derivatives 4 were subjected to the reaction of 1,2-ethylenediamine in the presence of diisopropylethylamine (1.0 equiv) to furnish the corresponding TPEN derivatives 6 in 58% and 79% yields, respectively, as summarized in Scheme 1.
Extraction behavior of thus obtained TPEN derivative 6 for soft metal ions was studied with cadmium(II) ion, which serves as a class of soft Lewis acid and employed as a model study for the extraction of minor actinides, by solvent extraction experiments.2a-c A 1.0 mM solution of Cd(NO3)2, whose pH was controlled to 1-3 by the addition of nitric acid and ammonium nitrate. The cadmium ion was extracted into the 1 mM chloroform solution containing equimolar amount of TPEN 6 toward Cd2+ by shaking the mixture at 25 ºC for 5 min. The extracted amount of Cd2+ ion (%E) was estimated by measuring the concentration of remaining Cd2+ in the aqueous phase by ICP analysis.7 Table 1 summarizes the results of %extraction under several pH values. As summarized in the table, extraction performance of TPEN derivative 6 is moderate to good under various pH regions. It is particularly noteworthy to exhibit little drop of the performance in 6b under highly acidic conditions.2b This is probably due to the effect of hydrophobic properties by the introduction of the ring fusion toward pyridine.
Our interest was then focused on the use of TPEN derivatives as a polymer gel for temperature-dependent change of extraction behaviors. We have been shown that TPEN-NIPA gels, which is a copolymer of NIPA (N-isopropyl acrylamide) and a TPEN derivative, extracts soft metal ions from the corresponding aqueous solution containing the ion and exhibits temperature-dependent change of extraction since poly-NIPA reversibly swells and shrinks at LCST (lower critical solution temperature = ca. 32 ºC in poly-NIPA) in aqueous media.2d-f Thus, we designed a pyridine derivative 4c that possessed a polymerizable double bond. Synthesis of 4c was carried out as shown in Scheme 2. Methacryl amide was treated with propargyl bromide 7 to afford N,N-dipropargylated amide 2c in 78% yield. The obtained 4c was subjected to the [2+2+2]cyclization with bromoacetonitrile in the presence of ruthenium catalyst 3 to afford 2-(bromomethyl)pyridine 4c in 65% yield. Following reaction with 1,2-ethylenediamine resulted to give TPEN 6c as a comonomer in 64% yield. TPEN-NIPA gel was prepared by the radical polymerization of NIPA and 6c (2.5 mol%) with 4.1 mol% of AIBN as an initiator. The synthetic pathway was summarized in Scheme 3.
The obtained TPEN-NIPA gel was subjected to the extraction of cadmium(II) ion at different temperatures (0 and 50 ºC) and pH (2 and 5). Although it was unable to study spectroscopic characterization of TPEN-NIPA gel due to the little solubility in most of organic and aqueous solvents, the gel exhibited reversible swelling and shrinking between 0 ºC and 50 ºC. Solvent extraction with 6c was also examined as a control experiment. Table 2 shows extraction behavior of 6c at the pH range of 1-3. It was found that the extraction performance of 6c was slightly inferior to those of 6a and 6b probably due to more hydrophilic characteristics by the introduction of amide group.
Figure 1 summarizes the results of extraction at pH = 2 and 5 (at 0 and 50 ºC), respectively, with 6c-NIPA gel. At pH = 5, the gel extracted cadmium ion highly effectively in the swollen state at 0 ºC, while little extraction was observed in the shrinked gel at 50 ºC. Although the extracted amount of Cd was lower at pH = 2, the similar temperature-dependent change was also observed. The extraction performance was comparable to that with TPEN-NIPA gel derived from the 2-propenyloxy and 2-methyl-2-propenyloxy derivatives.2e,g
In summary, we have designed several TPEN derivatives and synthesis of the obtained TPEN 6 was performed. Total synthetic scheme of 6 was simple and the synthetic reactions proceeded smoothly. The synthetic strategy is recognized as a facile preparative method for TPEN derivatives, accordingly. The synthesized TPEN derivatives was found to show excellent extraction performances both in the liquid-liquid extraction and the extraction with polymer gel. Further studies with TPEN derivatives particularly directed to the extraction of minor actinides are now under way.
EXPERIMENTAL
General. Unless otherwise noted, all the synthetic experiments were conducted under a nitrogen atmosphere with standard Schlenk technique. 1H and 13C NMR spectra were measured by Varian Gemini 300 spectrometer (300 MHz for 1H; 75 MHz for 13C). Chemical shifts were expressed in ppm using CHCl3 (7.24 ppm) or the central peak of CDCl3 (77 ppm) as an internal standards. IR spectra were recorded on Bruker Alpha with an ATR attachment (Ge). High resolution mass spectra (HRMS, ESI) were measured by JEOL JMS-T100LP AccuTOF LC-Plus with a JEOL MS-5414 DART attachment. ICP-AES analyses were carried out by SII SPS3100 at the Center for Supports to Research and Education Activities of Kobe University. For thin layer chromatography (TLC) analyses throughout this work, Merck precoated TLC plates (silica gel 60 F254) were used. Ruthenium catalyst (Cp*Ru(cod)Cl) was prepared by the literature procedure5e and employed for the catalytic reaction. Other chemicals were purchased and used without further purification.
General procedure for the [2+2+2]Cycloaddition of 1,ω-dialkyne with bromoacetonitrile in the presence of Cp*Ru(cod)Cl: To a solution of Cp*Ru(cod)Cl (38 mg, 0.1 mmol) in 9 mL of CH2Cl2 was added bromoacetonitrile (70 µL, 1.0 mmol) dropwise. After stirring at room temperature for 15 min, 1,ω-diyne (1.5 mmol) dissolved in 9 mL of CH2Cl2 was added dropwise to the resulting mixture. Stirring was continued for 10 h at room temperature. Solvent was removed under reduced pressure to leave a crude oil, which was purified by column chromatography on silica gel using hexane:EtOAc = 3:1 as an eluent to afford 121 mg of bromomethylpyridine 4a (57% yield). 1H NMR (CDCl3) δ 2.10 (tt, J=4.5, 4.5 Hz, 2H), 2.89 (t, J=4.5 Hz, 2H), 2.91 (t, J=4.5 Hz, 2H), 4.53 (s, 2H), 7.30 (s, 1H), 8.40 (s, 1H); 13C NMR (CDCl3) δ 24.9, 29.9, 32.5, 34.3, 119.6, 139.7, 145.2, 154.1, 155.0; IR: 3009, 2963, 2868, 1602, 1482, 1210, 890, 768, 622 cm-1; HRMS (DART+) found: m/z 212.0075, Calcd for C9H1179BrN (M+H): 212.0075.
Bromomethylpyridine 4b derived from 2b and bromoacetonitrile: Synthesis of 4b was carried out in a similar manner to that of 4a to afford 244 mg of 4b (68% yield). 1H NMR (CDCl3) δ 1.26 (t, J=7.1 Hz, 6H), 3.58 (s, 2H), 3.60 (s, 2H), 4.21 (q, J=7.1 Hz, 4H), 4.53 (s, 2H), 7.31 (s, 1H), 8.40 (s, 1H); 13C NMR (CDCl3) δ 13.9, 33.9, 37.9, 40.0, 60.0, 62.0, 119.3, 135.8, 145.1, 151.0, 155.1, 170.7; IR: 3422, 2977, 1733, 1441, 1251, 1191, 1070, 859, 704, 629 cm-1; HRMS (DART+) found: m/z 356.0490, Calcd for C15H1979BrNO4 (M+H): 356.0498.
TPEN derivative 6a: To a solution of bromomethylpyridine 4a (1.89 g, 8.91 mmol) and diisopropylethylamine (1.55 mL, 8.91 mmol) in 50 mL of MeCN was added 1,2-ethylenediamine (119 µL, 1.78 mmol) under a nitrogen atmosphere. The resulting mixture was heated at 80 °C for 12 h and then cooled to room temperature. After removal of the solvent, the crude product was purified by column chromatography on alumina using EtOAc:MeOH = 20:1 as an eluent to afford 601 mg of 6a (58% yield). 1H NMR (CDCl3) δ 1.99 (tt, J=4.4, 4.5 Hz, 8H), 2.71 (s, 4H), 2.75 (t, J=4.5 Hz, 8H), 2.80 (t, J=4.4 Hz, 8H), 3.67 (s, 8H), 7.29 (s, 4H), 8.25 (s, 4H); 13C NMR (CDCl3) δ 24.9, 29.8, 32.5, 52.1, 60.5, 118.8, 138.1, 144.3, 154.0, 157.1; IR: 3003, 2950, 2842, 1679, 1606, 1562, 1108, 883, 768 cm-1; HRMS (ESI+) found: m/z 585.3702, Calcd for C38H45N6 (M+H): 585.3706.
TPEN derivative 6b: Synthesis of 6b was performed in a similar manner to that of 6a (79% yield). 1H NMR (CDCl3) δ 1.24 (t, J=7.1 Hz, 24H), 2.76 (s, 4H), 3.52 (s, 8H), 3.55 (s, 8H), 3.70 (s, 8H), 4.19 (q, J=7.1 Hz, 16H), 7.29 (s, 4H), 8.30 (s, 4H); 13C NMR (CDCl3) δ 13.5, 37.4, 39.7, 51.6, 52.5, 59.6, 61.4, 118.3, 134.1, 144.0, 149.7, 157.6, 170.5; IR: 2981, 2820, 1731, 1608, 1445, 1269, 1185, 1068, 862, 721 cm-1; HRMS (ESI+) found: m/z 1161.5396, Calcd for C62H77N6O16 (M+H): 1161.5396.
N,N-Dipropargylmethacrylamide (2c): To a solution of methacrylamide (2.55 g, 30 mmol) in 150 mL of MeCN were added propargyl bromide (6.47 mL, 75 mmol) and KOH (4.21 g, 75 mmol). The resulting mixture was stirred at room temperature for 2 days to afford 3.72 g of 2c (77% yield). 1H NMR (CDCl3) δ 1.97 (dd, J=1.0, 1.6 Hz, 3H), 2.26 (s, 2H), 4.31 (s, 4H), 5.24 (dq, J=1.0, 1.6 Hz), 5.27 (dq, J=1.0, 1.0 Hz ); 13C NMR (CDCl3) δ 20.1, 32.5- 39.1, 72.5-73.1, 78.0-78.1, 116.7, 139.3, 171.5; IR: 3292, 3085, 2979, 2119, 1651, 1625, 1455, 1294, 1193, 926, 663 cm-1; HRMS (DART+) found: m/z 162.0919, Calcd for C10H12NO (M+H): 162.0919.
[2+2+2]Cycloaddition of 2c with bromoacetonitrile: The reaction was carried out in a similar manner to that for the synthesis of 2c to afford 546 mg of 4c (65% yield). 1H NMR (CDCl3) δ 2.00 (s, 3H), 4.55 (s, 2H), 4.85 (s, 2H), 4.88 (s, 2H), 5.26 (s, 1H), 5.37 (s, 1H), 7.33-7.41 (s, 1H), 8.47-8.54 (s, 1H); 13C NMR (CDCl3) δ 19.4, 33.4, 51.1, 51.6, 116.6, 117.6, 132.3, 140.3, 143.5, 146.7, 155.5, 170.4; IR: 3084, 3040, 2975, 2864, 1650, 1615, 1572, 1266, 937, 785, 632 cm-1; HRMS (DART+) found: m/z 281.0286, Calcd for C12H1479BrN2O (M+H): 281.0290.
TPEN derivative 6c: The synthesis was performed in a similar manner to that of 6a to afford 354 mg of 6c (64% yield). 1H NMR (CDCl3) δ 1.80 (s, 12H), 2.62 (s, 4H), 3.60 (s, 8H), 4.63 (s, 8H), 4.69 (s, 8H), 5.08 (s, 4H), 5.18 (s, 4H), 7.22-7.28 (s, 4H), 8.23-8.28 (s, 4H); 13C NMR (CDCl3) δ 20.0, 51.7, 52.1, 53.8, 60.3, 117.1, 131.7, 140.9, 143.5, 144.0, 146.6, 158.9, 171.3; IR: 3078, 2921, 2864, 1650, 1615, 1426, 1174, 925, 789 cm-1; HRMS (ESI+) found: m/z 861.4545, Calcd for C50H57N10O4 (M+H): 861.4564.
Preparation of TPEN-NIPA gel by copolymerization of 6c and N-isopropyl acrylamide: To a 10 mL of screwcapped test tube were added N-isopropylacrylamide (13.6 mg, 0.12 mmol) and TPEN derivative 6c (0.003 mmol). The mixture was dissolved in 0.024 mL of DMF and AIBN (4.1 mol%) was added in one portion to the solution. The resulting mixture was heated at 60 ºC for 18 h. Then, the mixture was cooled to room temperature and washed with water repeatedly. Water was removed under reduced pressure at 50 ºC to leave a colorless solid. Since the obtained TPEN-NIPA gel was found to be insoluble in any organic solvents as well as in water, attempted characterization of the polymer gel was unsuccessful.
Solvent extraction of Cd2+ with TPEN derivatives: A 1 mM of aqueous Cd(NO3)2 solution was prepared. The pH value of the solution was controlled to 1.0 to 3.0 by the addition of 1 M of aq. NH4NO3 and 1 M of HNO3. TPEN derivative (0.01 mmol) was dissolved in a 10 mL of chloroform. The aqueous 1 mM Cd(NO3)2 solution (1.0 mL) and the organic solutions (1.0 mL) was stirred vigorously at 25 ºC for 5 min. Aliquot of the aqueous phase (0.8 mL) was taken and diluted with 3.2 mL of distilled water. The resulting solution was subjected to ICP-AES analysis to estimate the concentration of Cd2+. Calculation of %extraction value was performed by the following equation.
%E = 100·D/(D+1), {D = ([Cd2+]ini-[Cd2+])/[Cd2+]}
[Cd2+]ini: concentration of Cd2+ in water before extraction
[Cd2+]: concentration of Cd2+ in water after extraction
Extraction of cadmium(II) ion with TPEN-NIPA gel: A 1 mM of aqueous Cd(NO3)2 solution was prepared. The pH value of the solution was controlled to 2.0 and 5.0 by the addition of 1 M of aq NH4NO3 and 1 M of HNO3. TPEN-NIPA gel (2.5 mol% of TPEN contents), whose concentration of the TPEN moiety was controlled to be 3.0 μmol, was added to 1.5 mL of the aqueous solution. Vigorous stirring of the mixture was continued for 60 min at 5 °C or 50 °C. An aliquot of the solution (0.2 mL) was taken and the solution was diluted by distilled water to 4.0 mL, which was subjected to ICP-AES analysis. The percent extraction value was calculated as
%E = 100·D/(D+1), {D = ([Cd2+]ini‒[ Cd2+])/[ Cd2+] }
[Cd2+]ini: concentration of Cd2+ in water before extraction;
[Cd2+]: concentration of Cd2+ in water after extraction.
ACKNOWLEDGEMENTS
This work was partially supported by KAKENHI (21655051) for Challenging Exploratory Research by Japan Society for the Promotion of Science (JSPS).
References
1. a) N. Hirayama, S. Iimuro, K. Kubono, H. Kokusen, and T. Honjo, Anal. Chim. Acta, 1997, 339, 115; CrossRef b) M. Sokolov, K. Umakoshi, and Y. Sasaki, Inorg. Chem., 2002, 41, 6237; CrossRef c) L. Zhang, H. Tang, J. Tang, Y. Shen, L. Meng, M. Radosz, and N. Arulsamy, Macromolecules, 2009, 42, 4531. CrossRef
2. a) T. Kida, Y. Inaba, W. Watanabe, Y. Nakajima, S. Fukuoka, K. Takeshita, and A. Mori, Chem. Lett., 2010, 39, 774; CrossRef b) T. Ogata, K. Takeshita, G. A. Fugate, and A. Mori, Separation Science and Technology, 2008, 43, 2630; CrossRef c) T. Ogata, K. Takeshita, K. Tsuda, and A. Mori, Separation and Purification Technology, 2009, 68, 288; CrossRef d) S. Fukuoka, T. Kida, Y. Nakajima, T. Tsumagari, W. Watanabe, Y. Inaba, A. Mori, T. Matsumura, Y. Nakano, and K. Takeshita, Tetrahedron, 2010, 66, 1721; CrossRef e) T. Maekawa, T. Kida, Y. Miyazaki, W. Watanabe, Y. Inaba, K. Takeshita, and A. Mori, Bull. Chem. Soc. Jpn., 2011, 84, 122; CrossRef f) K. Takeshita, K. Ishida, Y. Nakano, and T. Matsumura, Chem. Lett., 2007, 36, 1032; CrossRef g) Y. Inaba, T. Tsumagari, T. Kida, W. Watanabe, Y. Nakajima, S. Fukuoka, A. Mori, T. Matsumura, Y. Nakano, and K. Takeshita, Polym. J., in press. CrossRef
3. a) Z. Kolarik, Chem. Rev., 2008, 108, 4208; CrossRef b) B. S. Creaven, D. F. Donlon, and J. McGinley, Coord. Chem. Rev., 2009, 253, 893. CrossRef
4. S. Saito and Y. Yamamoto, Chem. Rev., 2000, 100, 2901. CrossRef
5. a) B. Heller and M. Hapke, Chem. Soc. Rev., 2007, 36, 1085; CrossRef b) J. A. Varela and C. Saá, Chem. Rev., 2003, 103, 3787; CrossRef c) Y. Yamamoto, R. Ogawa, and K. Itoh, J. Am. Chem. Soc., 2001, 123, 6189; CrossRef d) Y. Yamamoto, K. Kinpara, H. Nishiyama, and K. Itoh, Adv. Synth. Catal., 2005, 347, 1913; CrossRef e) Y. Yamamoto, K. Kinpara, R. Ogawa, H. Nishiyama, and K. Itoh, Chem. Eur. J., 2006, 12, 5618; CrossRef f) H. Hara, M. Hirano, and K. Tanaka, Org. Lett., 2008, 10, 2537; CrossRef g) K. Kase, A. Goswami, K. Ohtaki, E. Tanabe, N. Saino, and S. Okamoto, Org. Lett., 2007, 9, 931. CrossRef
6. L. Q. Xu, F. Yao, and G. D. Fu, Macromolecules, 2009, 42, 6385; CrossRef G. V. Myasoedova, O. B. Mokhodoeva, and I. V. Kubrakova, Anal. Sci., 2007, 23, 1031. CrossRef