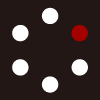
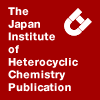
HETEROCYCLES
An International Journal for Reviews and Communications in Heterocyclic ChemistryWeb Edition ISSN: 1881-0942
Published online by The Japan Institute of Heterocyclic Chemistry
e-Journal
Full Text HTML
Received, 1st August, 2011, Accepted, 5th September, 2011, Published online, 13th September, 2011.
■ Synthesis of Norbornane-Fused 1,3-Dithiolanes and Evaluation of 1,3-Dithiolane-Containing Polymers as Absorbants for Mercury(II) Salts
R. Alan Aitken,* Kati M. Aitken, Stuart Lambert, Richard Playfair, and Neil J. Wilson
School of Chemistry, University of St. Andrews, Fife KY 16 9ST, U.K.
Abstract
Hydrolysis of the zwitterionic adducts formed from Bu3P, CS2 and norbornene or norbornadiene gives Bu3PO and the corresponding norbornane-fused 1,3-dithiolanes. Oxidation of these gives endo-sulfoxides while attempted hydrolysis using HgCl2 and CdCO3 instead leads to formation of crystalline 1:1 HgCl2 adducts. Based on this observation, a range of robust and readily accessible polymers containing norbornane-fused dithiolane units are evaluated for absorption of HgCl2 from aqueous solution.INTRODUCTION
Some time ago we reported that strained bicyclic alkenes such as norbornene (bicyclo[2.2.1]heptene) react with Bu3P and CS2 to generate the intermediate ylide 1 which, in the presence of an aldehyde, undergoes a Wittig reaction to form alkylidenedithiolanes 2.1 In the absence of an aldehyde, a pink solid adduct formulated as 3 can be isolated and this undergoes cycloaddition with acetylenic esters such as DMAD to give dihydrotetrathiafulvalene derivatives 4.2 With norbornadiene the corresponding adduct 7 was formed and could also be converted into bis(alkylidenedithiolanes)1 and bis- (dihydrotetrathiafulvalenes).2 In the course of chromatographic purification of the products from these reactions we consistently isolated traces of stable byproducts which appeared to have the 2-unsubstituted dithiolane structures 5 and 8. We report here their synthesis and characterisation as well as their remarkable affinity for mercuric chloride.
RESULTS AND DISCUSSION
The structure of product 5 suggested its formation by hydrolysis of the ylide 1 which is in equilibrium with the CS2 adduct 3 and this was supported by concomitant formation of Bu3PO. After 3 was found to be inert towards aqueous acetic or hydrochloric acid, successful hydrolysis was achieved by stirring a CH2Cl2 solution of 3 with wet alumina for 18 h. Filtration, evaporation and chromatography of the residue on alumina gave dithiolane 5 as a colourless liquid in 30% yield. By changing to silica for both hydrolysis and chromatography, the same product was obtained but in only 6% yield. In the case of 7, wet alumina hydrolysis followed by distillation to remove Bu3PO and sublimation of the residue gave 8 as colourless crystals in 51% yield whereas use of silica gave only 8%.
In order to obtain a crystalline derivative of the liquid dithiolane 5, it was subjected to oxidation with mCPBA and afforded the monosulfoxide 9 in moderate yieldafter recrystallisation. In the case of the bis(dithiolane) 8, treatment with two equivalents of mCPBA afforded a mixture of regioisomeric disulfoxides 10 and 11 whose identity was clear from their 1H and particularly 13C NMR spectra. The differing symmetry of the two compounds results in the bridgehead CH groups being distinct in 10 but equivalent in 11. It is notable that in each case there was no evidence of further oxidation, formation of two sulfoxide functions on the same ring, nor formation of stereoisomeric sulfoxides. All sulfoxide functions are believed to be endo as shown.
Given the ready availability of the reagents used to prepare 5, we were interested to investigate its cleavage to the corresponding dithiol 6 which might thus provide a general method for overall conversion of strained alkenes into the corresponding 1,2-dithiols. This transformation is currently not easy to achieve and one of the few methods known involves reaction of norbornene with sulfur and ammonia in DMF to give the 1,2,3-trithiolane followed by reduction of this with sodium in liquid ammonia.3 The standard method to cleave 1,3-dithiolanes, first introduced by Hach in 19534 and subsequently much used to cleave the homologous 1,3-dithianes, involves treatment with mercuric chloride and cadmium carbonate in hot ethanol. When this was applied to compounds 5 and 8, however no trace of the expected thiols 6 and 13 was obtained. Instead the products were colourless solids which proved to be the 1:1 adducts 12 and 14 with mercuric chloride. These showed NMR data very little different from the starting dithiolanes and elemental analysis data in reasonable agreement with expectation. Such adducts from cyclic sulfides are in fact well known, with typical examples involving systems such as 1,3,5-trithiane,5 1,4-oxathiane,6 tetrathiacyclotetradecane,7 dithiapropellanes,8 and tetrahydrotetrathiafulvalene.9 In fact the 13C NMR data for 12 and 14 together with the 1:1 ratio observed in each case allow us to predict the precise mode of coordination of the HgCl2, with equal coordination of HgCl2 to both sulfurs in 12 giving a symmetrical structure with only 5 13C NMR signals. In 14, the 1:1 ratio as well as observation of only 4 13C NMR signals is consistent with a polymeric structure with a repeat unit as shown involving one HgCl2 coordinated between the four sulfurs of two adjacent molecules of 8. These structures are in fact in good agreement with the previous HgCl2 complexes mentioned above, where there are examples of one HgCl2 linked to two sulfurs from the same ring in a monomeric structure as for 12,7and HgCl2 shared between two sulfurs from different rings to form either a monomeric6,9 or polymeric5,8 structure, or shared between four sulfurs in a polymeric structure like 14.8
The high affinity of these norbornane-fused dithiolanes for mercury prompted us to evaluate the mercury affinity of a series of polymers whose preparation we reported some time ago,10 by treatment of norbornadiene with tributylphosphine and carbon disulfide and an aromatic di- or trialdehyde. Using isophthalaldehyde and terephthalaldehyde respectively gave polymers 15 and 16 while mesitaldehyde gave the three dimensional structure 17.10 In addition a copolymer 18 formed using a 2:3 mixture of mesitaldehyde and terephthalaldehyde with a hybrid structure between 16 and 17 was also prepared and evaluated. All the polymers are highly insoluble stable solids that are readily formed from low cost
starting materials and contain a high proportion of sulfur (e.g. 37% by weight for 15 and 16). They would thus seem to have considerable potential for removal of mercury ions from water, an area that has attracted considerable recent interest, with a range of sulfur-containing silica materials being among the most effective.11 In a brief preliminary study, a sample of the para polymer 16 (1 g) was placed in a short glass column between layers of sand and covered with water. When a solution of HgCl2 (100 mg) in water (10 cm3) was applied and eluted with water (50 cm3), evaporation of the water collected gave only 30 mg of HgCl2. The absorbed mercury caused the initially yellow polymer to turn grey-green and could be partly recovered by washing through with 1 M NaCl solution. A more detailed quantitative study was therefore undertaken.
For this the polymers (1 g) were mixed with sand (2–2.5 g) and placed in a glass column between two layers of pure sand. The column was then wetted thoroughly with water and a solution of HgCl2 in water (50 cm3) was passed through, followed by water (50 cm3). The mercury from the collected solutions was determined by gravimetric analysis after precipiation as [Cu(en)2] [HgI4].12 The method was first validated by analysis of five samples containing between 5 mg and 110 mg of HgCl2 in water (100 cm3). A calibration curve was constructed and showed a good correlation with R2 = 0.9992 (see Experimental Section). This was then used to correct the weights obtained. The results are presented in Table 1.
After some experimentation, optimum conditions were established. The polymer, in the finely powdered form directly as obtained from the synthesis,10 (1 g) was mixed with sand (2.5 g) since this gave efficient absorption and a slow flow rate such that the mercury solution (50 cm3) passed through under gravity within 12 h. Using less sand resulted in much slower flow (up to 1 week) while using more gave faster flow (3 h) but resulted in lower mercury absorption. Sand (1 g) was placed in the column, followed by the sand/polymer mixture and finally sand (7.5 g). Only then was the column thoroughly wetted from above (without applying external pressure) resulting in an evenly packed column with no visible air bubbles. Wetting at an earlier stage led to the light polymer floating up on top of the water. The four polymers were now compared (Table 1, entries 1–4) and it is clear that the meta polymer 15 behaved very poorly while the other three all gave good absorption. It might also be noted that 15 was white while the other three polymers were all yellow in colour. The determination of run 2 was repeated (run 5) and this gives an idea of the reproducibility of the results. Changing to a wider column with a thinner layer of polymer led to faster flow rate and consequently lower absorption of mercury (run 6). When the colum from run 6 was washed thoroughly with aq NaCl then water and reused for another run, its absorption capacity was less but still significant (run 7), and this trend was continued when regenerating and reusing a third time led to a further fall to 2/3 of the original capacity.
These results are encouraging bearing in mind the inherently low cost of the polymers, particularly 16, and their robust nature. The improvement in performance by 17 and the copolymer 18 is unexpected and merits further detailed studies.
EXPERIMENTAL
Melting points were recorded on a Reichert hot-stage microscope and are uncorrected. IR spectra were determined on a Perkin-Elmer 1710 spectrometer as Nujol mulls or thin films. All NMR spectra were obtained using a Varian Gemini 2000 spectrometer, at 300 MHz for 1H and 75 MHz for 13C, and are reported in ppm to high frequency of TMS as internal standard. Spectra were recorded in CDCl3 unless otherwise stated. Mass spectra were recorded using an AEI Kratos MS-50 spectrometer using EI (70 eV) or, where noted, CI (isobutane). Elemental analysis was performed using a Carlo-Erba 1106 analyser. Alumina was Laporte Industries alumina Type H.
Starting Materials
Compounds 3 and 71 as well as polymers 15, 16 and 1710 were prepared as described previously. Polymer 18 was prepared using the same method10 but with a mixture of mesitaldehyde and terephthalaldehyde in a 2:3 molar ratio.
Preparation of 2,6-exo-3,5-dithiatricyclo[5.2.1.02,6]decane 5
4-Dithiocarboxylato-4-tri-n-butylphosphonio-3,5-dithiatricyclo[5.2.1.02,6]decane 3 (4.50 g, 10 mmol) was dissolved in CH2Cl2 (150 mL) and Al2O3 (2.00 g) preadsorbed with water (0.20 g, 11.11 mmol) was added. The mixture was stirred for 24 h, Al2O3 removed by filtration, and the CH2Cl2 removed in vacuo. Flash column chromatography (Al2O3, CH2Cl2—petroleum, 1:1) of the residue followed by distillation (Kugelrohr) gave the product 5 (0.52 g, 30%) as a colourless oil; bp 95 ˚C/ 0.2 Torr; IR 1716, 1654, 1471, 1450, 1313, 1299, 1183 and 1043 cm–1; 1H NMR δ 3.866 and 3.761 (AB pattern, J = 11 Hz, 2H, 4-H), 3.603 (d, J = 2 Hz, 2H, 2,6-H), 2.35 (s, 2H, 1,7-H), 1.908 (dt, 1H, J = 10, 2 Hz, 10-H), 1.617 (second order pattern*, 2J = 8, 3J = 2, 3J = 1, 4J = 2 Hz, 2H, 8,9endo-H), 1.208 (second order pattern*, 2J = 8, 3J = 2, 3J = 1 Hz, 2H, 8,9exo-H), 1.11 (dt, J = 10, 2 Hz, 1H, 10-H); 13C NMR δ 62.0 (C-2, C-6), 43.6 (C-1, C-7), 37.3 (C-4), 32.7 (C-10), 27.7 (C-8, C-9); MS m/z 172 (M+, 100), 126 (33), 105 (44), 93 (52) and 66 (49). Anal. Calcd for C8H12S2: C, 55.76; H, 7.02. Found: C, 55.44; H, 6.85.
(* Coupling constants derived by simulation)
Preparation of 2,6-exo-8,12-exo-3,5,9,11-tetrathiatetracyclo[5.5.1.02,6.08,12]tridecane 8
2,6-exo-8,12-exo-4,10-Bis(dithiocarboxylato)-4,10-bis(tri-n-butylphosphonio)-3,5,9,11-tetrathiatetracyclo[5.5.1.02,6.08,12]tridecane 7 (6.00 g, 7.49 mmol) was suspended in CH2Cl2 (100 mL) and Al2O3 (2.00 g) preadsorbed with water (0.20 g, 11.1 mmol) was added. The mixture was stirred for 16 h, the Al2O3 removed by filtration, and the CH2Cl2 removed in vacuo. The residue was Kugelrohr distilled to remove tri-n-butylphosphine oxide (105 ˚C, 0.1 Torr) and the remaining material sublimed (160 ˚C, 0.1 Torr) to give the product 8 (0.94 g, 51%) as colourless crystals; mp 158–159 ˚C; IR 1448, 1420, 1312, 1292, 1264, 1220, 1188, 1169, 1079, 1029, 922, 858 cm–1; 1H NMR δ 3.97 and 3.85 (AB pattern, J = 11 Hz, 4H, 4,10-H), 3.66 (s, 4H, 2,6,8,12-H), 2.45 (s, 2H, 1,7-H), 1.88 (s, 2H, 13-H); 13C NMR δ 60.9 (C-2, C-6, C-8, C-12), 50.1 (C-1, C-7), 37.5 (C-4, C-10), 27.0 (C-13); MS m/z 248 (M+, 77), 157 (27), 104 (63), 57 (100). Anal. Calcd for C9H12S4: C, 43.51; H, 4.87. Found: C, 43.77; H, 4.88.
Preparation of 2,6-exo-3,5-dithiatricyclo[5.2.1.02,6]decane 3-endo-oxide 9
A solution of m-chloroperoxybenzoic acid (70–75%, 0.265 g, 1.49–1.60 mmol) in CH2Cl2 (10 mL) was added to a solution of 2,6-exo-3,5-dithiatricyclo[5.2.1.02,6]decane 5 (0.25 g, 1.45 mmol) in CH2Cl2 (50 mL). The solution was stirred for 16 h to afford a purple solution (λmax 558 nm). This was washed with saturated aqueous NaHCO3 solution (3 × 30 mL), dried and the solvent removed by evaporation to give a pale yellow oil which crystallised upon standing. Recrystallisation from hexane/EtOAc gave the title compound 9 (0.12 g, 43%) as colourless crystals; mp 97–99 ˚C; IR 2796, 1452, 1314, 1300, 1254, 1076, 1042 cm–1; 1H NMR δ 4.02 and 3.60 (AB pattern, J = 10 Hz, 2H, 4-H), 3.57 and 2.92 (AB pattern, J = 6 Hz, 2H, 2,6-H), 2.92 (br s, 1H, 1-H), 2.22 (br s, 1H, 7-H), 1.81 and 1.30 (AB pattern, J = 10 Hz, 2H, 10-H), 1.79–1.62 (m, 2H, 8,9-H), 1.28–1.14 (m, 2H, 8,9-H); 13C NMR δ 76.9 (C-2), 52.5 (C-6), 50.1 and 41.0 (C-1, C-7), 38.9 (C-4), 34.2 (C-10), 27.3 (C-8, C-9); MS (CI) m/z 189 (M+H+, 100), 126 (13), 93 (12), 66 (7), 56 (25). Anal. Calcd for C8H12OS2: C, 51.03; H, 6.42. Found: C, 50.89; H, 6.36.
Preparation of 2,6-exo-8,12-exo-3,5,9,11-tetrathiatetracyclo [5.5.1.02,6.08,12]tridecane dioxide 10/11
A solution of m-chloroperoxybenzoic acid (70–75%, 0.29 g, 1.17–1.25 mmol) in CH2Cl2 (10 mL) was added to a solution of 2,6-exo-8,12-exo-3,5,9,11-tetrathiatetracyclo[5.5.1.02,6.08,12]tridecane 8 (0.25 g, 1.01 mmol) in CH2Cl2 (50 mL). The solution was stirred for 5 h, a second portion of m-chloroperoxybenzoic acid (0.29 g, 1.17–1.25 mmol) added and the solution stirred for a further 18 h after which time a white precipitate was observed to have formed. The mixture was washed with saturated aqueous NaHCO3 solution (3 × 30 mL) and the solvent removed in vacuo to give the title compound 10/11 (0.18 g, 64%) as a white powder as a mixture of isomers; mp 240–242 ˚C); IR 1460, 1389, 1315, 1294, 1258, 1242, 1155, 1083, 1049, 1022, 930, 901 cm–1; MS (CI) m/z 281 (M+H+, 100), 265 (7), 187 (26); HRMS: Calcd for C9H13O2S4 (M+H): 280.9798. Found: 280.9787.
2,6-exo-8,12-exo-3,5,9,11-tetrathiatetracyclo[5.5.1.02,6.08,12]tridecane 3,11-dioxide 10; 1H NMR (CD3OD) δ 4.40 and 3.94 (AB pattern, J = 5.5 Hz, 4H, 4,10-H), 3.77 and 3.17 (AB pattern, J = 4 Hz, 4H, 2,6,8,12-H), 3.42 (s, 1H, 1-H), 2.23 (s, 1H, 7-H), 1.99 (s, 2H, 13-H); 13C NMR δ 76.6 (C-2, C-12), 52.1 (C-6, C-8), 52.0 (C-1), 47.1 (C-7), 45.0 (C-4, C-10), 32.1 (C-13).
2,6-exo-8,12-exo-3,5,9,11-tetrathiatetracyclo[5.5.1.02,6.08,12]tridecane 3,9-dioxide 11; 1H NMR (CD3OD) δ 4.40 and 3.93 (AB pattern, J = 5.5 Hz, 4H, 4,10-H), 3.82 and 3.12 (AB pattern, J = 4 Hz, 4H, 2,6,8,12-H), 2.80 (s, 2H, 1,7-H) and 1.99 (s, 2H, 13-H); 13C NMR δ 76.4 (C-2, C-8), 52.5 (C-6, C-12), 50.1 (C-1, C-7), 44.8 (C-4, C-10), 30.8 (C-13).
Reaction of 2,6-exo-3,5-dithiatricyclo[5.2.1.02,6]decane 5 with HgCl2
To a solution of 2,6-exo-3,5-dithiatricyclo[5.2.1.02,6]decane 5 (0.35 g, 2.03 mmol) in EtOH (10 mL) was added cadmium carbonate (0.38 g, 2.2 mmol). A solution of mercury(II) chloride (0.60 g, 2.2 mmol) in EtOH (10 mL) was added dropwise over a period of 2 h and the mixture stirred for 3 h at 50–60 ˚C. Unreacted CdCO3 was removed by filtration, and the solid washed with hot water (20 mL) which resulted in a white precipitate being formed in the filtrate. This was removed by filtration and dried over P2O5 in a vacuum dessicator to give the product 12 (0.74 g, 82%) as a white powder; mp 116–118 ˚C; IR 1458, 1312, 1299, 1251, 1148, 1139, 947 cm–1; 1H NMR δ 4.17 and 4.05 (AB pattern, J = 11 Hz, 2H, 4-H), 3.93 (d, J = 2 Hz, 2H, 2,6-H), 2.49 (m, 2H, 1,7-H), 1.65–1.44 (m, 3H, 8,9,10-H syn), 1.33 (d, J = 8 Hz, 2H, 8,9-H), 1.20 (d, J = 10 Hz, 1H, 10-H anti); 13C NMR δ 62.9 (C-2, C-6), 45.1 (C-1, C-7), 39.6 (C-4), 34.5 (C-10), 28.0 (C-8, C-9); MS m/z 272 (HgCl2+, 45), 237 (HgCl+, 3), 202 (Hg+, 17), 172 (dithiolane+, 100), 126 (27), 105 (35), 93 (42). Anal. Calcd for C8H12Cl2HgS2: C, 21.65; H, 2.73. Found: C, 20.87; H, 2.40.
Reaction of 2,6-exo-8,12-exo-3,5,9,11-tetrathiatetracyclo [5.5.1.02,6.08,12]tridecane 8 with HgCl2
To a solution of 2,6-exo-8,12-exo-3,5,9,11-tetrathiatetracyclo[5.5.1.02,6.08,12]tridecane 8 (0.50 g, 2.01 mmol) in THF (25 mL) and water (2 mL) was added mercury(II) chloride (1.10 g, 4.05 mmol) and cadmium carbonate (0.70 g, 4.05 mmol). The mixture was heated under reflux for 52 h after which unreacted CdCO3 was removed by filtration and hot water (25 mL) added to the filtrate. The resulting precipitate was isolated by filtration and dried over P2O5 in a vacuum dessicator to give the product 14 (0.87 g, 83%) as colourless crystals; mp 189–191 ˚C; IR 1736, 1460, 1317, 1293, 1083, 921, 868 cm–1; 1H NMR δ 4.00 and 3.89 (AB pattern, J = 11 Hz, 4H, 4,10-H), 3.70 (s, 4H, 2,6,8,12-H), 2.48 (s, 2H, 1,7-H), 1.86 (s, 2H, H-13); 13C NMR δ 60.4 (C-2, C-6, C-8, C-12), 50.9 (C-1, C-7), 38.5 (C-4, C-10), 28.0 (C-13); MS m/z 272 (HgCl2+, 9), 248 (dithiolane+, 100), 202 (Hg+, 19), 169 (12), 143 (7), 123 (14), 104 (69). Anal. Calcd for C9H12Cl2HgS4: C, 20.79; H, 2.33. Found: C, 23.45; H, 2.47.
Mercury absorption studies
The columns used were standard small glass chromatography columns equipped with a sintered glass disc at the bottom and were of either 1 cm or 2.5 cm outside diameter, leading respectively to 3.5 cm and 1 cm deep layers of the polymer/sand mixtures. After filling as described earlier, the mercury solutions were allowed to run through under gravity and the collected eluates were analysed as follows:12
A neutral solution of HgCl2 (0–0.50 mmol) in distilled water (100 cm3) was treated with KI (2–3 g) and heated to 80 °C. It was then treated with a boiling solution of [Cu(en)2](NO3)2 (0.16 g, 0.52 mmol) and the mixture was allowed to cool to room temperature overnight. The precipitate of [Cu(en)2][HgI4] was filtered off using a weighed crucible, washed with a small amount of an aqueous solution of KI (0.1%) and [Cu(en)2](NO3)2 (0.1%), followed by EtOH (10 cm3) and Et2O (10 cm3). The crucible was dried in a vacuum desiccator and weighed to get the yield of the complex.
References
1. R. A. Aitken, T. Massil, and S. V. Raut, J. Chem. Soc., Chem. Commun., 1994, 927; R. A. Aitken, K. Carcas, L. Hill, T. Massil, and S. V. Raut, Tetrahedron, 1997, 53, 2261. CrossRef
2. R. A. Aitken, L. Hill, and P. Lightfoot, Tetrahedron Lett., 1997, 38, 7927. CrossRef
3. T. C. Shields and A. N. Kurtz, J. Am. Chem. Soc., 1969, 91, 5415. CrossRef
4. V. Hach, Chem. Listy, 1953, 47, 227 [Chem. Abstr., 1955, 49, 1021].
5. W. R. Costello, A. T. McPhail, and G. A. Sim, J. Chem. Soc. (A), 1966, 1190. CrossRef
6. R. S. McEwen and G. A. Sim, J. Chem. Soc. (A), 1967, 271. CrossRef
7. N. W. Alcock, N. Herron, and P. Moore, J. Chem. Soc., Dalton Trans., 1978, 394. CrossRef
8. F. H. Herbstein, P. A. Ashkenazi, M. Kaftory, M. Kapon, G. M. Reisner, and D. Ginsburg, Acta Cryst., Sect. B, 1986, 42, 575. CrossRef
9. M. D. Glick, W. H. Isley, and A. R. Siedle, Inorg. Chem., 1981, 20, 3819. CrossRef
10. R. A. Aitken, L. Hill, T. Massil, M. B. Hursthouse, and K. M. Abdul Malik, Tetrahedron, 1997, 53, 10441. CrossRef
11. J. Brown, L. Mercier, and M. Jaroniec, Chem. Commun., 1999, 69; CrossRef A. M. Liu, K. Hidajat, S. Kawi, and D. Y. Zhao, Chem. Commun., 2000, 1145; CrossRef V. Antochshuk and M. Jaroniec, Chem. Commun., 2002, 258; CrossRef L. Zhang, W. Zhang, J. Shi, Z. Hua, Y. Li, and J. Yan, Chem. Commun., 2003, 210. CrossRef
12. A. I. Vogel, 'A Textbook of Quantitative Inorganic Analysis', Longmans, London, 3rd Edition, 1961, p. 488.