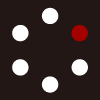
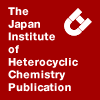
HETEROCYCLES
An International Journal for Reviews and Communications in Heterocyclic ChemistryWeb Edition ISSN: 1881-0942
Published online by The Japan Institute of Heterocyclic Chemistry
e-Journal
Full Text HTML
Received, 30th June, 2011, Accepted, 8th August, 2011, Published online, 10th August, 2011.
DOI: 10.3987/REV-11-SR(P)5
■ Recently Discovered Naturally Occurring Heterocyclic Organohalogen Compounds
Gordon W. Gribble*
6128 Burke Laboratory, Department of Chemistry, Dartmouth College, Hanover, New Hampshire 03755, U.S.A.
Abstract
Amongst the more than 5,000 naturally occurring organohalogen compounds, both biotic and abiotic, are a diverse collection of structurally novel heterocycles. The present review covers this fascinating area of natural products as presented over the past three years.CONTENTS
I. Introduction
II. Marine Organisms
A. Algae
B. Sponges
C. Corals
D. Tunicates
E. Nudibranchs
F. Bryozoans
G. Fungi
H. Bacteria
I. Cyanobacteria
J. Miscellaneous Marine Animals
III. Terrestrial Organisms
A. Plants
B. Fungi
C. Bacteria
D. Slime Molds
E. Higher Animals
IV. Summary
V. References
I. INTRODUCTION
The number of known natural organohalogen compounds has grown from 30 in 19681 to 200 in 19732 to more than 5,000 today.3-5 A number of factors account for this extraordinary increase: (1) a revitalization of natural products research in the search for new medicinal compounds, (2) the development of improved isolation, separation, and identification techniques (e.g., SCUBA, remote unmanned submersible vessels, 2D NMR, HRMS), (3) selective bioassays to identify biologically active extracts, and (4) an awareness of folk medicine and ethnobotany for guidance to potentially important organisms.
Thus, despite the persistent ignorance of the prevalence of organohalogen in nature,6,7 the number of identified natural organohalogen compounds continues to increase. The present review surveys heterocyclic organohalogen natural products reported in the period 2009-2011. As defined by the journal, coverage includes epoxides, lactones, and lactams along with traditional heterocycles.
II. Marine organisms
A. Algae
Seaweeds are a rich source of organohalogen compounds,8 epitomized by the favorite edible seaweed of native Hawaiians, Asparagopsis taxiformis ("limu kohu"), which contains more than 100 organohalogens and is prized for its rich aroma and flavor.9,10
The red algal genus Laurencia comprises at least 140 species and continues to yield novel halogenated compounds. Laurencia composita from the East China Sea contains the new 2-bromospironippol (1) and 10-bromo-3-chloro-2,7-epoxychamigr-9-en-8β-ol (2), along with several known organohalogens.11,12 A collection of Laurencia saitoi from the Hainan Chinese coast has furnished 2-hydroxyluzofuranone (3), 2-hydroxyluzofuranone B (4), 4-hydroxypalisadin C (5), (9S)-2-bromo-3-chloro-6,9-epoxybisabola-7(14),10-diene (6), (9R)-2-bromo-3-chloro-6,9-epoxybisabola-7(14),10-diene (7), and 10-bromo-3-chloro-2,7-epoxychamigr-9-en-8α-ol (8), an epimer of 2.13,14
A sample of Laurencia glandulifera from the island of Crete contains five novel halogenated tetrahydrofurans (e.g., 9, 10) along with a putative biosynthetic precursor 11.15
The new chamigrenes, gomerolactones A-D, two of which contain chlorine (12, 13), were isolated from Laurencia majuscula in the Canary Islands.16 This site also yielded eight new halogenated acetogenins from a collection of Laurencia marilzae, including 12-epoxyobtusallene IV (14) and marilzallene (15).17
A Brazilian Laurencia catarinensis contains seven new related halogenated sesquiterpenes, four of which, (5S)-5-acetoxycaespitol (16-19), are shown.18 A Philippines sample of Laurencia sp. has furnished laurefurenynes A-F, two of which contain bromine (20, 21).19
The Fijian red alga Callophycus serratus is a treasure trove of brominated diterpene-benzoate macrolides, the bromophycolides, and 12 new examples were discovered recently, bringing the total to 21.20,21 Bromophycolide S (22) is shown here.21 The Australian red alga Chondria armata, a genus closely related to Laurencia, has yielded three novel brominated triterpene polyethers, the aplysiols C-E (e.g., 23), along with several previously known halogenated compounds.22 Noteworthy, this paper revises the relative stereochemistry of aplysiol B.
The first example of a bastadin-type metabolite to be isolated from a seaweed is lithothamnin A (24) from the red alga Lithothamnion fragilissimum found in Palau.23
The lesser explored brown algae have also yielded novel heterocyclic organohalogens in the past three years. Thus, 4'-chlorostypotriol triacetate (25) was identified in Stypopodium flabelliforme from the Easter Island region (after peracetylation of the extract).24 A Russian population of the brown alga Dicytyota dichotoma has provided the novel dictyohydroperoxide (26),25 and an Australian sample of Sargassum fallax contains the unusual meroditerpenoid fallachromenoic acid (27).26
The marine chrysophyte alga Chrysophaeum taylori has yielded eight new antimicrobial chrysophaentins A-H, four of which are shown here A-D (28-31).27 These compounds are vaguely related to the liverwort bazzanins.4
B. Sponges
Like marine algae, sponges have long been an amazingly rich source of halogenated metabolites. It is likely that associated bacteria are the origin of at least some of the compounds described herein. Many sponge metabolites are heterocyclic derivatives of bromine-containing tyrosines. New examples include the prototypical (but non-heterocycle) JBIR-44 (32) from an Okinawan sponge Psammaplysilla purpurea,28 the adenine-substituted aphrocallistin (33) from a deep water collection of Aphrocallistes beatrix in Florida,29 and tyrokeradines A (34) and B (35) from an Okinawan Verongida sponge, compounds that contain a novel imidazolyl-quinolone subunit.30
The commonly encountered spirocyclohexadienyl isoxazole moiety is seen with the new sunabedine (36) from an Okinawan Verongida sponge.31 Pseudoceratinazole A (not shown) from the Australian Pseudoceratina sp. would appear to be a diastereomer of 36 from the data given.32 The Australian sponge Hyattella sp. contains the new antimalarial psammaplysin G (37)33 and psammaplysin F (38),34 which is very potent against the malaria parasite, and was isolated along with 37 from an Australian Pseudoceratina sp. sponge.34
An Okinawan Pseudoceratina sp. contains the new ceratinadins A-C (39-41),35 and the Australian sponge Ianthella flabelliformis has yielded three new bastadins, bastadin 25 (42), 15-O-sulfonatobastadin 11 (43), and bastadin 26 (44).36 The related sponge Ianthella cf. reticulata contains (E,Z)-bastadin 19 (not shown), a diastereomer of the known bastadin 19, and the novel dioxepine bastadin 3 (45).37
A dazzling array of new bromotryptophan-derived sponge metabolites was also described over the past three years. The Australian Ancorina sp. produces the antimalarial (+)-7-bromotrypargine (46),38 and the highly intricate dictazoles A and B (47), and the related dictazolines C-E (not shown) were isolated from a Panamanian sponge Smenospongia cerebriformis, all but one of which contain at least one bromine.39
Seven new analogues of the well-known 2-bromoindole cyclodepsipeptide jaspamide (= jasplakinolide) from the sponge Jaspis splendens have been described by three groups.40-42 These include jaspamides M and N,40 R,41 and jasplakinolides Z3, Z4, Z5, and V.42 This latter study reports the revision of jasplakinolides R1 (48) (= jaspamide R) and W. Paltolide C (49) is a related 6-bromoindole cyclic peptide found in the Palau sponge Theonella swinhoei.43
Four new chlorine-containing cyclic depsipeptides, mirabamides E, F, G (50), and H (51), were isolated from the Australia Torres Strait sponge Stelletta clavosa.44 Mirabamides E and F are l-rhamnosyl derivatives of G and H, respectively. The Australian sponge Suberea clavata contains clavatadines C (52) and D (53), novel guanidine alkaloids related to the known purealdin L.45
New examples of the pyrroloiminoquinone discorhabdins continue to be discovered, four of which contain bromine,46-48 e.g., 16a,17a-dehydrodiscorhabdin W (54).47
A collection of the recently discovered Agelas linnaei from Indonesia has yielded 11 new bromopyrroles (e.g., 55-60), including the new taurine analogue 61.49
Sponges of the Agelasidae family have also yielded several new dimeric pyrrole-2-aminoimidazole alkaloids, several of which are brominated. Thus, benzoseptrin B (62)50 and C (63)51,52 were found in Agelas cf. mauritiana (Guadalcanal), Phakellia sp. (New Caledonia),50 Agelas dendromorpha (New Caledonia),51 and Agelas sp. (Okinawa).52 In addition, one of these studies uncovered the new agelastatins E (64) and F (65).51
Other examinations of Agelas sponges have yielded the bromopyrrole alkaloids nagelamides O and P, mukanadins E and F,53 and nagelamides Q and R,54 while a study of Stylissa carteri has uncovered the new stylissazoles A-C.55 The New Caledonian Cymbastela cantharella contains two new dihydrohymenialdisines,56 and the acanthamides A-D along with two other new bromopyrrole alkaloids that were identified in Acanthostylotella sp.57 Unfortunately, space does not permit depicting these metabolites.
The Fijian sponge Siliquariaspongia sp. has afforded a series of bromine-containing motualevic acids, one of which is a heterocycle, motualevic acid F (66), along with the corresponding methyl ester (4E)-(R)-antazirine (67).58 A Chinese Xestospongia testudinaria contains 39 new brominated polyunsaturated lipids, the xestospongienes, several of which are lactones, e.g., xestospongiene A (68) and xestospongiene Z12 (69), and one is a furan, xestospongiene Z11 (70).59 The unique polyketide phosphodiesters, franklinolides A-C (71-73), were isolated from a Geodia sp. sponge encrusted with a Halichondria sp.60
A Western Australia Phorbas sp. has produced the new phorbasides G-I (e.g., 74) in nanogram quantities.61 The black marine sponge Halichondria okadai yielded pinnarine (75), a new member of the novel halichlorine family of macrocyclic alkaloids.62
C. Corals
In contrast to marine algae and sponges, gorgonian soft corals generally contain chlorine and not bromine, and typically embedded in a dazzling array of briarane diterpenoids.
Recent examples include briaexcavatin U (76)63 and excavatoid P (77)64 from the cultured octocoral Briareum excavatum, and juncin ZII (78)65 and juncenolides H-J (79-81)66 from Junceella juncea living in the South China Sea.
The gorgonian Junceella fragilis continues to be the source of numerous new briaranes, such as the chlorine containing fragilides E (82),67 F (83),68 G (84),68 12-epi-G (85),69 H (86),70 J (87),71 and robustolide L (88).71 The first iodine-containing briaranes to be found in nature are the dichotellides A-E (89-93) from Dichotella gemmacea.72
The novel 15-chlorodeoxypseudopterolide (94), the first halogenated pseudopterane diterpene to be discovered, was isolated from the Bahamian octocoral Pseudopterogorgia acerosa.73 Whereas 94 displays activity against the HCT116 cell line, the nonchlorinated derivative is inactive. The soft coral Lobophytum pauciflorum contains seven biscembranoids, one of which, lobophytone D (95), is chlorinated.74
Interestingly, only 95 of this series is active in inhibiting inducible nitric oxide synthase. The Antarctic soft coral Alcyonium grandis has yielded six chlorine-containing sesquiterpenoids, but only 96 is a heterocycle.75
D. Tunicates
Tunicates (= ascidians) (class Ascidiacea) are a rich and diverse source of halogenated metabolites, most of which are bromine-containing heterocycles.
The Australian ascidian Aplidium caelestis produces the novel quinolone carboxylic acids, caelestines A-D (97-100), a previously unknown class of natural organohalogens.76 An unidentified Madagascan tunicate has yielded the first marine proaporphine alkaloids, saldedines A (101) and B (102).77
The sub-arctic ascidian Synoicum pulmonaria from the coast of Norway has yielded synoxazolidinones A-C (103-105), marine metabolites containing the rare 4-oxazolidinone in nature.78,79 Tunicates of the genus Eudistoma continue to afford brominated β-carboline alkaloids, and a recent example is eudistomidin G (106) from the Okinawan Eudistoma glaucus.80 The New Zealand ascidian Didemnum sp., also a rich repository of bromine-containing alkaloids, has yielded didemnidine B (107) along with the non-brominated didemnidine A.81
Leptoclinidamine C (108) was identified in the Australian ascidian Leptoclinides durus and this novel bromoindole alkaloid contains the rare 5-(methylthio)histidine unit.82 An ascidian from the Great Barrier Reef, Eusynstyela latericus, has furnished the three dimeric 6-bromoindole metabolites eusynstyelamides A-C (109-111).83
E. Nudibranchs
Only one heterocyclic organohalogen was reported from a nudibranch in this three year time frame. The novel benzofuro[3,2-b]pyrrole 112 was actually isolated from a Pseudoalteromonas sp. on the surface of an unidentified nudibranch collected in Oahu.84
F. Bryozoans
The Australian bryozoan Amathia wilsoni contains the novel wilsoniamines A (113) and B (114), which possess the hexahydropyrrolo[1,2-c]imidazol-1-one ring system, unprecedented in nature.85 The New Zealand Pterocella vesiculosa has yielded the new β-carboline alkaloid 115.86 The prolific Flustra foliacea continues to exhibit its synthetic virtuosity and a collection of this animal from Scandinavia and Canada has yielded nine new brominated flustramine alkaloids, 116-124.87 Two related dimers (not shown) may be isolation artifacts.
The Arctic bryozoan Tegella cf. spitzbergensis has afforded the new eusynstyelamides D-F (125-127), in addition to the enantiomer (not shown) of eusynstyelamide B (110).88
G. Fungi
Although not found in a marine fungus, one of the first halogenated natural products to be identified was griseofulvin in Penicillium griseo-fulvum. A new derivative of this fungal metabolite is 128, isolated from the marine mangrove fungus Sporothrix sp. from the South China Sea coast.89 The marine fungus Acremonium sp. found living on a Stelletta sp. sponge on the Korean coast produces the sesquiterpenoids acremofuranone A (129) and B (130), along with several non-heterocyclic chlorinated analogues.90 One of three new cochliomycins, cochliomycin C (131), was isolated from a gorgonian (Dichotella gemmacea)-derived fungus Cochliobolus lunatus collected in the South China Sea. These novel resorcylic acid lactones display activity against larval settlement of the barnacle Balanus amphitrite.91
The sponge Halichondria japonica-derived fungus Gymnascella dankaliensis has afforded four new gymnastatins I-K (132-135) when the culture medium contains bromide.92 The novel notoamide N (136) was isolated from a marine Aspergillus sp. fungus, the only one of 16 related new metabolites from this organism to contain chlorine.93
The fungus Chaetomium globosum that was originally isolated from the marine fish Mugil cephalus has yielded a large number of cytotoxic metabolites designated as chaetomugilins. New examples include chaetomugilins G (137),94 H (138),94 seco-A (139),95 seco-D (140),95 11-epi-A (141),96 and 4'-epi-A (142).96
H. Bacteria
Like the study of marine fungi, the exploration of marine bacteria has intensified in recent years,97 and one notable member of this class, salinosporamide A (NPI-0052), has advanced into human clinical trials as an anticancer agent.98 The organism that produces salinosporamide A, Salinispora tropica, has also afforded antiprotealide (143),99 while the related marine sediment bacterium, Salinispora arenicola, from Palau produces the chlorohydrin saliniquinone C (144), which is related to the pluramycin-altromycin class of metabolites.100 Saliniquinone A (not chlorinated) displays potent inhibition of the human colon HCT-116 cell line (IC50 9.9 x 10-9 M). A marine Saccharomonospora sp. from a La Jolla California sediment has yielded the novel alkaloid lodopyridone (145), which is also active against the HCT-116 cell line.101 The marine bacterium Thermovibrio ammonificans living on a deep-sea hydrothermal vent chimney on the East Pacific Rise yielded its first identified secondary metabolites, ammonificins A (146) and B (147).102
A marine-derived sediment bacterium from Fiji, Nocardiopsis sp., has afforded fijiolides A (148) and B (149).103 These inhibitors of NFκB activation possess a chlorocyclopenta[a]indene ring system similar to that found in the sporolides and cyanosporasides. Marine Streptomyces species are a rich source of unique halogenated metabolites, including mansouramycin B (150) isolated from a sediment collected on the North Coast of Germany,104 ammosamides A (151) and B (152) from a Bahaman bottom sediment,105 and JBIR-34 (153) and JBIR-35 (154) from a sponge (Haliclona sp.)-derived Streptomyces from Japan.106 Both ammosamides A and B show significant in vitro cytotoxicity against HCT-116 (IC50 = 0.32 µM).105
The novel nitropyrrolins A-E were isolated from a marine-derived bacterium of the Streptomycetaceae family, and two of these metabolites, C (155) and E (156), are chlorohydrins.107 Although the source of the growing collection of polyhalogenated 1'-methyl-1,2'-bipyrroles (157) has yet to be established, their environmental ubiquity is expanding to being found in various marine mammals108 and in the liver of the tiger shark (Galeocerto cuvier).109 Most likely these unusual halogenated bipyrroles are the product of marine bacteria.
A La Jolla deep ocean sediment has yielded what is probably a new Streptomyces species, which produces the novel bipyrrolic marinopyrroles A-F.110 New examples of these remarkable compounds reported in 2010 include marinopyrroles C (158), D (159), E (160) and F (161),111 which, except for F (161), exist as optically active atropoenantiomers, similar to that observed for 5,5’-dichloro-1,1’-dimethyl-3,3’,4,4’-tetrabromo-2,2’-bipyrrole.112 The marinopyrroles display excellent activity against methicillin-resistant Staphylococcus aureus bacteria.110,111 The marine dinoflagellate Karlodinium veneficum produces several powerfully toxic ichthyotoxins, including karlotoxin 2 (= KmTx 2) (162),113 65-E-chloro-KmTx 1 (163),114 and 64-E-chloro-KmTx 3 (164).114 These compounds are responsible for massive fish kills and significant economic loss to the seafood industry.
I. Cyanobacteria
More than 1,000 natural products have been identified in cyanobacteria (blue-green algae),115 many of which contain halogen, usually chlorine. Three new chlorinated ambiguine K, M, and O isonitriles (165-167) were isolated from cultured Fischerella ambigua.115 Both 165 and 166 show potent activity against Mycobacterium tuberculosis and Bacillus anthracis. Further study of this same cyanobacterium characterized the new fischambiguine B (168) along with related non-chlorinated analogues.116
The cyanobacteria genus Lyngbya is an amazing source of novel metabolites, including many chlorine-containing compounds. Leading the genus is Lyngbya majuscula, and a recent study of this alga from Grenada has identified four new organochlorines, two of which, itralamides A (169) and B (170), are depsipeptides.117 A Floridian sample of this cyanobacterium has afforded the new 8-epi-malyngamide C (171), the latest in a series of about 30 known malyngamides, most of which do not contain a heterocyclic ring.118 A related epoxide-containing metabolite is 8-O-acetyl-8-epi-malyngamide C (172) that was found in a Grenada collection of Lyngbya majuscula.119 This investigation also identified 171 in the cyanobacterium.
A study of Lyngbya bouillonii from Guam has discovered six new organochlorine and organobromine metabolites, 2-epi-lyngbyaloside (173), 18E- (174) and 18Z-lyngbyaloside C (175), 27-deoxylyngbyabellin A (176), lyngbyabellin J (177), and laingolide B (178), along with several related previously known compounds.120 A separate study of this Guamanian cyanobacterium discovered bouillomide B (179).121
A different cyanobacterium from Guam, Lyngbya semiplena, has afforded three new cyclodepsipeptides including the bromine-containing lyngbyastatin 10 (180).122 Another bromophenol, bisebromoamide (181), was identified in an Okinawan Lyngbya sp.,123 but the original structure was revised slightly by total synthesis.124
Two different cyanobacteria species, Symploca cf. hydnoides from Guam125 and Oscillatoria margaritifera from Panama,126 contain the same collection of cyclic depsipeptides, the veraguamides, several of which contain the rare bromoacetylene function. Veraguamides A (182) and B (183) were found in both studies,125,126 while K (184) and L (185) were only characterized from Oscillatoria margaritifera.126 Veraguamide A was quite potent against the H-460 human lung cancer cell line (LD50 = 0.14 µM).126
The fresh water cyanobacterium Microcystis sp. from a water reservoir in Israel yielded the novel aeruginosins KY642 (186) and KY608 (187).127 A different reservoir in Israel containing a Microcystis aeruginosa bloom afforded seven chlorinated protease inhibitor micropeptins: HU1069 (188), HU989 (189), HU1041 (190), HU975 (191), HU895A (192), HU909 (193), and HU895B (194).128 These compounds inhibit trypsin with IC50's in the range 0.7-5.2 µM, and chymotrypsin with IC50's of 2.8-72 µM. The cyanobacterium Anabaena constricta has furnished the novel indole alkaloid bromoanaindolone as an unequal mixture of both enantiomers (195).129
J. Miscellaneous Marine Animals
Over the years the sea hare Aplysia dactylomela has provided synthetic chemists with a multitude of enticing target molecules. A recent examination of this animal from Puerto Rico has revealed the novel bromotriterpene polyethers aplysqualenol A (196) and B (197),130 which are related to the well known thyrsiferol and suggestive of a dietary source for the sea hare. The sea anemone Parazoanthus axinellae contains five new hydantoin alkaloids, two of which are the bromine-containing parazoanthine D (198) and E (199).131 Bunodosine 391 (200) is a toxic component of the venom of the sea anemone Bunodosoma cangicum.132 This compound displays potent analgesic activity as mediated by serotonin receptors. The marine ciliate Pseudokeronopsis riccii contains the novel bromopyrrole keronopsamides A-C (201-203).133
III. TERRESTRIAL ORGANISMS
While fewer in overall numbers, terrestrial naturally occurring organohalogens have a longer history of discovery than marine organohalogens, and new examples continue to be unearthed. As in Section II, only new heterocyclic compounds discovered in 2009-2011 are presented.
A. Plants
Naturally occurring iridoids and secoiridoids, both chlorinated and non-chlorinated, have a long history in folk medicine as beneficial phytochemicals.134 The roots of Valeriana officinalis from China have yielded volvaltrate B (204) along with a non-chlorine containing analogue.135 Three new chlorinated iridoid glucosides, asystasioside E (205) and longifoliosides A (206) and B (207) were isolated from Veronica longifolia.136 The latter two compounds display radical-scavenging activity. The Chilean plant Mimulus glabratus contains the new 6-chlorohalleridone (208), a rare 2-chlorocyclohexenone, along with halleridone, also known as rengyolone.137
An investigation of the Chinese medicinal plant Ligularia atroviolacea has identified 24 compounds, including the new furoeremophilane derivative 209.138 Another Chinese herbal medicinal plant, Lindera aggregata, has afforded five new sesquiterpene lactones, including linderagalactone A (210), which possesses a novel rearranged carbon framework.139 The Chinese perennial herb Artemisia anomala, which is also used in Chinese folk medicine as an analgesic and antibiotic, contains the new guaianolide artanomalide B (211).140
The Tanzanian tree Tessmannia densiflora produces the isocoumarin 212,141 and the Chinese medicinal plant Blumea riparia, which is used to treat gynecological diseases, contains the novel xanthene blumeaxanthene II (213), the first example of a halogenated xanthene from a plant.142 The Asian evergreen tree Podocarpus macrophyllus contains the new makilactone E (214) along with several non-chlorinated bisnor- and norditerpene dilactones.143 Crassifoside H (215) is a glucosyl-fused tetracyclic chlorophenol found in the Chinese folk medicine plant Curculigo glabrescens.144
Although halogenated steroids are rare in nature, several chlorine-containing withanolides are known, and a recent example is 216, isolated from the Pakistanian Withania somnifera.145 The chloro lactone triterpenoid 217, a derivative of the ubiquitous oleanolic acid, was characterized from the roots of Actinidia chinensis, a Chinese folk medicine anticancer plant.146
B. Fungi
Some of the earliest reported natural organohalogen compounds were described from terrestrial fungi; for example, drosophilin A methyl ether, sporidesmin A, 2,4-dichlorophenol, ascochlorin, and chloromethane.4
The fungus Leptoxyphium sp., also known as genus Caldariomyces, contains the new dichlorodiketopiperazine 218, which is 10-20 times more active than the nonchlorinated analogue in the inhibition of CCL2-induced chemotaxis.147 The Japanese discomycete fungus Lachnum palme has afforded the novel dibenzo-α-pyrones palmariol A (219) and B (220).148 Dehydrofuligoic acid (221) is a novel yellow polyene-pyrone that is found in the mushroom Fuligo septica f. flava.149
The parasitic fungus Isaria tenuipes produces the novel bicyclic hemiacetal isariotin F (222). In addition to its infection of insect pupae and larvae, isariotin F exhibits activity against the malaria parasite and displays activity in several human cancer cell lines.150 Four of the six photosensitive polyketide malbranpyrroles isolated from the thermophilic Malbranchea sulfurea fungus living in fumarole soil in Taiwan are the 3-chloropyrrole derivatives malbranpyrroles C-F (223-226).151 Unlike these latter metabolites, the two nonchlorinated compounds were not cytotoxic against several human cancer cell lines.
A new atpenin, NBRI23477 A (227), was isolated from the fermentation broth of Penicillium atramentosum PF1420 from a Japanese soil sample. This novel chlorine-containing pyridine inhibited the growth of the human prostate cancer DU-145 cell line.152 The ascochlorin precursor LL-Z1272α epoxide (228) is produced by a mutant of Ascochyta viciae.153 Two studies of the fungus Pochonia chlamydosporia var. chlamydosporia have harvested nine new chlorinated radicicol analogues, pochonins G-I (229-231)154 and K-P (232-237).155 Like the well known radicicol, these pochonins inhibit WNT-5A expression. WNT-5A is a secretory glycoprotein implicated in hair loss.
The azaphilones are a large collection of related fungal metabolites produced via a polyketide biosynthesis and numbering nearly 200 compounds, many of which contain chlorine.156 The name for these biologically active compounds derives from their facile reaction with nitrogen nucleophiles ("aza-Michael" acceptors). Several new azaphilones have been reported in the past three years. A study of the microfungus Chaetomium sp., obtained from emu scat, resulted in the isolation of the new chaetoviridin E (238) and revision of the previously known chaetoviridins B and D.157 Chaetoviridin E is a diastereomer of chaetoviridin A. Chaetomugilin D (239) was isolated from Chaetomium globosum, an endophytic fungus growing on the Chinese medicinal plant Ginkgo biloba.158 This new azaphilone is the dehydroxy analogue of the previously known chaetomugilin A, which was also found in this fungus. A separate study of this fungus found growing on the leaves of Viguiera robusta yielded five new chlorinated azaphilones, 5'-epichaetoviridin A (240), 4'-epichaetoviridin F (241), 12β-hydroxychaetoviridin C (242), and chaetoviridins G (243) and H (244).159 The absolute configuration of 240 was determined by Mosher's method.
The fungus Chaetomium brasiliene from Thailand afforded ten mollicellins, four of which are new and one of these, mollicellin M (245), is chlorinated.160 All of these mollicellins show antimalarial, antifungal, anti-tuberculosis, and anticancer activity to some degree. The endophytic microfungus Pestalotiopsis sp., grown on damp white rice, yielded the novel caprolactams, pestalactams A-C, two of which, A (246) and C (247), are chlorinated.161
The endophytic fungus Pestalotiopsis fici was isolated from the branches of the tea plant (Camellia sinensis) in China and cultured to yield the five chlorinated chloropupukeanolides A-E (248-252) and chloropupukeanone A (253).162,163 These stunningly complex natural products, which show significant anti-HIV and cytotoxic activity, feature a chloropupukeanane core, a novel spiroketal peroxide (248 and 249),162 and a chlorinated tricyclo[4.3.1.03,7]decane ring system suggestive of a Diels-Alder biosynthesis.162,163 Chloropestolide A (254) is another member of this extraordinary structural class.164
C. Bacteria
Terrestrial bacteria have a long and rich history in providing structurally unique and biologically potent halogenated natural products, the epitome being vancomycin, which has been used for 60 years to treat penicillin-resistant infections.
The three novel antibiotics, neopyrrolomycins B-D (255-257), are potent against Gram-positive pathogens and were obtained from a Streptomyces sp., which was isolated from an Arizona watershed soil sample.165 The closely related neopyrrolomycin A was characterized in 1990. The structure of celeastramycin A (258) has been revised by total synthesis.166 This Streptomyces sp. metabolite suppresses the production of IL-8 (IC50 0.06 µg/mL) in human endothelial cells. A soil sample from the Shaanxi province of China contains Streptomyces alboflavus, which produces NW-G01 (259), a novel cyclic hexadepsipeptide antibiotic, with strong antibacterial activity against Bacillus subtilis, Bacillus cereus, Staphylococcus aureus, and methicillin-resistant Staphylococcus aureus.167-169
Another Streptomyces sp. from an Australian soil sample has afforded two novel chlorinated actinomycins, Y1 (260) and Y2 (261).170 Genetically engineered Streptomyces fungicidicus has yielded three new enduracidin analogues 262-264.171
D. Slime Molds
Fuligoic acid (265) is a novel chlorinated polyene-pyrone found in the slime mold (myxomycete) Fuligo septica f. flava.172
E. Higher Animals
The Ecuadorian poison frog Epipedobates anthonyi, which contains the famous epibatidine, also possesses the novel alkaloid phantasmidine (266) along with N-methylepibatidine (267).173 Both of these alkaloids display activity at nicotinic acetylcholine receptors, although phantasmidine has an altered selectivity relative to epibatidine.
IV. SUMMARY
The field of organohalogen natural products has truly blossomed into an enormous scientific discipline. Ironically, evidence indicates that organohalogen compounds — both biotic and abiotic — have been on earth for centuries if not eons. Microfossils in Precambrian rocks, which are a billion years old, are identical to the cyanobacterium Nostoc, and other microfossils are morphologically indistinguishable from Oscillatoria, two present-day species rich in organohalogen metabolites.174
Several studies have identified polychlorinated dibenzo-p-dioxins and dibenzofurans in ancient sediments, which may have both a biotic and abiotic origin. Some halogenated bipyrroles (e.g., 157) and polybrominated diphenyl ethers were discovered in an archived sample of whale oil from the 1921 final voyage of the Charles W. Morgan whaling ship.175
Studies of emissions from several volcanoes have uncovered a large number of organohalogens, including heterocycles.176 Given the discovery of hydrogen chloride and hydrogen fluoride in interstellar space,177,178 it is tantalizing to foresee the identification of organohalogen compounds amongst the more than 100 stable organic compounds already detected in interstellar space.179 Peatlands, which comprise 2% of the earth’s continental surface, have accumulated 280-1,000 million tons of organochlorines during the postglacial period.180
But what is the raison d’être for natural organohalogens? This is the big question! Some organohalogens function as recyclers of halogen, as pheromones and hormones, as natural pesticides and anti-feedants, as anti-foulants, and as structural proteins. The benefit to mankind is that many organohalogen compounds display enormous biological activity that may lead to clinical drugs. Indeed, most of these promising compounds are heterocycles.
References
1. L. Fowden, Proc. Roy. Soc. B, 1968, 171, 5. CrossRef
2. J. F. Siuda and J. F. DeBernardis, Lloydia, 1973, 36, 107.
3. G. W. Gribble, unpublished compilation; the published total number of biogenic and abiogenic organohalogens through 2007 is 4,714 (refs. 4,5).
4. G. W. Gribble, Prog. Chem. Org. Nat. Prod., 1996, 68, 1.
5. G. W. Gribble, Prog. Chem. Org. Nat. Prod., 2010, 91, 1.
6. “Some types of synthetic compounds, including halogenated hydrocarbons such as PCB, are not found in nature,” I. B. Weinstein, Science, 1990, 250, 745.
7. “Unlike metals, most of these compounds [halogenated hydrocarbons] are man-made and do not occur naturally,” R. B. Clark “Marine Pollution,” 5th Ed. Oxford, 2001, p. 126.
8. For a review, see M. T. Cabrita, C. Vale, and A. P. Rauter, Mar. Drugs, 2010, 8, 2301. CrossRef
9. R. E. Moore, Acct. Chem. Res., 1977, 10, 40. CrossRef
10. O. McConnell and W. Fenical, Phytochemistry, 1977, 16, 367. CrossRef
11. N.-Y. Ji, X.-M. Li, K. Li, J. B. Gloer, and B.-G. Wang, Biochem. Syst. Ecol., 2009, 36, 938. CrossRef
12. N.-Y. Ji, X.-M. Li, and B.-G. Wang, Helv. Chim. Acta, 2010, 93, 2281. CrossRef
13. H. Su, Z.-H. Yuan, J. Li, S.-J. Guo, L.-P. Deng, L.-J. Han, X.-B. Zhu, and D.-Y. Shi, Helv. Chim. Acta, 2009, 92, 1291. CrossRef
14. N.-Y. Ji, X.-M. Li, K. Li, and B.-G. Wang, Helv. Chim. Acta, 2009, 92, 1873. CrossRef
15. M. Kladi, C. Vagias, P. Papazafiri, S. Brogi, A. Tafi, and V. Roussis, J. Nat. Prod., 2009, 72, 190. CrossRef
16. A. R. Díaz-Marrero, I. Brito, J. M. de la Rosa, L. D'Croz, O. Fabelo, C. Ruiz-Pérez, J. Darias, and M. Cueto, Eur. J. Org. Chem., 2009, 1407. CrossRef
17. A. Gutiérrez-Cepeda, J. J. Fernández, L. V. Gil, M. López-Rodríguez, M. Norte, and M. L. Souto, J. Nat. Prod., 2011, 74, 441. CrossRef
18. C. Lhullier, M. Falkenberg, E. Ioannou, A. Quesada, P. Papazafiri, P. A. Horta, E. P. Schenkel, C. Vagias, and V. Roussis, J. Nat. Prod., 2010, 73, 27. CrossRef
19. W. M. Abdel-Mageed, R. Ebel, F. A. Valeriote, and M. Jaspars, Tetrahedron, 2010, 66, 2855. CrossRef
20. A. L. Lane, E. P. Stout, A.-S. Lin, J. Prudhomme, K. Le Roch, C. R. Fairchild, S. G. Franzblau, M. E. Hay, W. Aalbersberg, and J. Kubanek, J. Org. Chem., 2009, 74, 27. CrossRef
21. A.-S. Lin, E. P. Stout, J. Prudhomme, K. Le Roch, C. R. Fairchild, S. G. Franzblau, W. Aalbersberg, M. E. Hay, and J. Kubanek, J. Nat. Prod., 2010, 73, 275. CrossRef
22. A. R. B. Ola, A.-M. Babey, C. Motti, and B. F. Bowden, Aust. J. Chem., 2010, 63, 90. CrossRef
23. A. W. W. Van Wyk, K. M. Zuck, and T. C. McKee, J. Nat. Prod., 2011, 74, 12. CrossRef
24. C. Areche, A. San-Martín, J. Rovirosa, J. Soto-Delgado, and R. Contreras, Phytochemistry, 2009, 70, 1315. CrossRef
25. S. A. Kolesnikova, E. G. Lyakhova, A. I. Kalinovsky, P. S. Dmitrenok, S. A. Dyshlovoy, and V. A. Stonik, Aust. J. Chem., 2009, 62, 1185. CrossRef
26. P. Reddy and S. Urban, Phytochemistry, 2009, 70, 25. CrossRef
27. A. Plaza, J. L. Keffer, G. Bifulco, J. R. Lloyd, and C. A. Bewley, J. Am. Chem. Soc., 2010, 132, 906. CrossRef
28. T. Fujiwara, J.-H. Hwang, A. Kanamoto, H. Nagai, M. Takagi, and K. Shin-ya, J. Antibiot., 2009, 62, 393. CrossRef
29. A. E. Wright, G. P. Roth, J. K. Hoffman, D. B. Divlianska, D. Pechter, S. H. Sennett, E. A. Guzmán, P. Linley, P. J. McCarthy, T. P. Pitts, S. A. Pomponi, and J. K. Reed, J. Nat. Prod., 2009, 72, 1178. CrossRef
30. H. Mukai, T. Kubota, K. Aoyama, Y. Mikami, J. Fromont, and J. Kobayashi, Bioorg. Med. Chem. Lett., 2009, 19, 133. CrossRef
31. N. Maru, T. Koyama, O. Ohno, K. Yamada, and D. Uemura, Heterocycles, 2010, 82, 371. CrossRef
32. Y. Feng, R. A. Davis, M. L. Sykes, V. M. Avery, D. Camp, and R. J. Quinn, Tetrahedron Lett., 2010, 51, 4847. CrossRef
33. X. Yang, R. A. Davis, M. S. Buchanan, S. Duffy, V. M. Avery, D. Camp, and R. J. Quinn, J. Nat. Prod., 2010, 73, 985. CrossRef
34. M. Xu, K. T. Andrews, G. W. Birrell, T. L. Tran, D. Camp, R. A. Davis, and R. J. Quinn, Bioorg. Med. Chem. Lett., 2011, 21, 84.
35. Y. Kon, T. Kubota, A. Shibazaki, T. Gonoi, and J. Kobayashi, Bioorg. Med. Chem. Lett., 2010, 20, 4569. CrossRef
36. A. R. Carroll, S. M. Kaiser, R. A Davis, R. W. Moni, J. N. A. Hooper, and R. J. Quinn, J. Nat. Prod., 2010, 73, 1173. CrossRef
37. L. Calcul, W. D. Inman, A. A. Morris, K. Tenney, J. Ratnam, J. H. McKerrow, F. A. Valeriote, and P. Crews, J. Nat. Prod., 2010, 73, 365. CrossRef
38. R. A. Davis, S. Duffy, V. M. Avery, D. Camp, J. N. A. Hooper, and R. J. Quinn, Tetrahedron Lett., 2010, 51, 583. CrossRef
39. J. Dai, J. I. Jiménez, M. Kelly, and P. G. Williams, J. Org. Chem., 2010, 75, 2399. CrossRef
40. F. Gala, M. V. D'Auria, S. De Marino, V. Sepe, F. Zollo, C. D. Smith, S. N. Keller, and A. Zampella, Tetrahedron, 2009, 65, 51. CrossRef
41. S. S. Ebada, V. Wray, N. J. de Voogd, Z. Deng, W. Lin, and P. Proksch, Mar. Drugs, 2009, 7, 435. CrossRef
42. K. R. Watts, B. I. Morinaka, T. Amagata, S. J. Robinson, K. Tenney, W. M. Bray, N. C. Gassner, R. S. Lokey, J. Media, F. A. Valeriote, and P. Crews, J. Nat. Prod., 2011, 74, 341. CrossRef
43. A. Plaza, J. L. Keffer, J. R. Lloyd, P. L. Colin, and C. A. Bewley, J. Nat. Prod., 2010, 73, 485. CrossRef
44. Z. Lu, R. M. Van Wagoner, M. K. Harper, H. L. Baker, J. N. A. Hooper, C. A. Bewley, and C. M. Ireland, J. Nat. Prod., 2011, 74, 185. CrossRef
45. M. S. Buchanan, A. R. Carroll, D. Wessling, M. Jobling, V. M. Avery, R. A. Davis, Y. Feng, J. N. A. Hooper, and R. J. Quinn, J. Nat. Prod., 2009, 72, 973. CrossRef
46. M. El-Naggar and R. J. Capon, J. Nat. Prod., 2009, 72, 460. CrossRef
47. T. Grkovic and B. R. Copp, Tetrahedron, 2009, 65, 6335. CrossRef
48. M. Na, Y. Ding, B. Wang, B. L. Tekwani, R. F. Schinazi, S. Franzblau, M. Kelly, R. Stone, X.-C. Li, D. Ferreira, and M. T. Hamann, J. Nat. Prod., 2010, 73, 38. CrossRef
49. T. Hertiani, R. Edrada-Ebel, S. Ortlepp, R. W. M. van Soest, N. J. de Voogd, V. Wray, U. Hentschel, S. Kozytska, W. E. G. Müller, and P. Proksch, Bioorg. Med. Chem., 2010, 18, 1297. CrossRef
50. J. Appenzeller, S. Tilvi, M.-T. Martin, J.-F. Gallard, H. El-bitar, E. T. H. Dau, C. Debitus, D. Laurent, C. Moriou, and A. Al-Mourabit, Org. Lett., 2009, 11, 4874. CrossRef
51. S. Tilvi, C. Moriou, M.-T. Martin, J.-F. Gallard, J. Sorres, K. Patel, S. Petek, C. Debitus, L. Ermolenko, and A. Al-Mourabit, J. Nat. Prod., 2010, 73, 720. CrossRef
52. T. Kubota, A. Araki, T. Yasuda, M. Tsuda, J. Fromont, K. Aoyama, Y. Mikami, M. R. Wälchli, and J. Kobayashi, Tetrahedron Lett., 2009, 50, 7268. CrossRef
53. T. Yasuda, A. Araki, T. Kubota, J. Ito, Y. Mikami, J. Fromont, and J. Kobayashi, J. Nat. Prod., 2009, 72, 488. CrossRef
54. A. Araki, T. Kubota, K. Aoyama, Y. Mikami, J. Fromont, and J. Kobayashi, Org. Lett., 2009, 11, 1785. CrossRef
55. K. Patel, R. Laville, M.-T. Martin, S. Tilvi, C. Moriou, J.-F. Gallard, L. Ermolenko, C. Debitus, and A. Al-Mourabit, Angew. Chem. Int. Ed., 2010, 49, 4775.
56. P. Sauleau, P. Retailleau, S. Nogues, I. Carletti, L. Marcourt, R. Raux, A. Al Mourabit, and C. Debitus, Tetrahedron Lett., 2011, 52, 2676. CrossRef
57. S. S. Ebada, R. Edrada-Ebel, N. J. de Voogd, V. Wray, and P. Proksch, Nat. Prod. Commun., 2009, 4, 47.
58. J. L. Keffer, A. Plaza, and C. A. Bewley, Org. Lett., 2009, 11, 1087. CrossRef
59. W. Jiang, D. Liu, Z. Deng, N. J. de Voogd, P. Proksch, and W. Lin, Tetrahedron, 2011, 67, 58. CrossRef
60. H. Zhang, M. M. Conte, and R. J. Capon, Angew. Chem. Int. Ed., 2010, 49, 9904. CrossRef
61. D. S. Dalisay and T. F. Molinski, J. Nat. Prod., 2010, 73, 679. CrossRef
62. S. Xu, H. Yoshimura, N. Maru, O. Ohno, H. Arimoto, and D. Uemura, J. Nat. Prod., 2011, 74, 132. CrossRef
63. P.-J. Sung, M.-R. Lin, and M. Y. Chiang, Chem. Lett., 2009, 38, 154. CrossRef
64. P.-J. Sung, G.-Y. Li, Y.-D. Su, M.-R. Lin, Y.-C. Chang, T.-H. Kung, C.-S. Lin, Y.-H. Chen, J.-H. Su, M.-C. Lu, J. Kuo, C.-F. Weng, and T.-L. Hwang, Mar. Drugs, 2010, 8, 263. CrossRef
65. S. H. Qi, S. Zhang, P. Y. Qian, and H. H. Xu, Chem. Nat. Compd., 2009, 45, 49. CrossRef
66. S.-S. Wang, Y.-H. Chen, J.-Y. Chang, T.-L. Hwang, C.-H. Chen, A. T. Khalil, and Y.-C. Shen, Helv. Chim. Acta, 2009, 92, 2092. CrossRef
67. P.-J. Sung, G.-Y. Li, Y.-P. Chen, I.-C. Huang, B.-Y. Chen, S.-H. Wang, and S.-K. Huang, Chem. Lett., 2009, 38, 454. CrossRef
68. P.-J. Sung, S.-H. Wang, M. Y. Chiang, Y.-D. Su, Y.-C. Chang, W.-P. Hu, C.-Y. Tai, and C.-Y. Liu, Bull. Chem. Soc. Jpn., 2009, 82, 1426. CrossRef
69. Y.-C. Chang, T.-L. Hwang, S.-K. Huang, L.-W. Huang, M.-R. Lin, and P.-J. Sung, Heterocycles, 2010, 81, 991. CrossRef
70. P.-J. Sung, S.-H. Wang, Y.-C. Chang, Y.-H. Chen, M.-R. Lin, I.-C. Huang, J.-J. Chen, J.-J. Li, T.-H. Kung, L.-S. Fang, W.-H. Wang, and C.-F. Weng, Bull. Chem. Soc. Jpn., 2010, 83, 1074. CrossRef
71. S.-H. Wang, Y.-C. Chang, M. Y. Chiang, Y.-H. Chen, T.-L. Hwang, C.-F. Weng, and P.-J. Sung, Chem. Pharm. Bull., 2010, 58, 928. CrossRef
72. J.-F. Sun, H. Huang, X.-Y. Chai, X.-W. Yang, L. Meng, C.-G. Huang, X.-F. Zhou, B. Yang, J. Hu, X.-Q. Chen, H. Lei, L. Wang, and Y. Liu, Tetrahedron, 2011, 67, 1245. CrossRef
73. A. S. Kate, K. Richard, B. Ramanathan, and R. G. Kerr, Can. J. Chem., 2010, 88, 318. CrossRef
74. P. Yan, Y. Lv, L. van Ofwegen, P. Proksch, and W. Lin, Org. Lett., 2010, 12, 2484. CrossRef
75. M. Carbone, L. Núñez-Pons, F. Castelluccio, C. Avila, and M. Gavagnin, J. Nat. Prod., 2009, 72, 1357. CrossRef
76. S. Yin, G. M. Boyle, A. R. Carroll, M. Kotiw, J. Dearnaley, R. J. Quinn, and R. A. Davis, J. Nat. Prod., 2010, 73, 1586. CrossRef
77. H. Sorek, A. Rudi, I. Goldberg, M. Aknin, and Y. Kashman, J. Nat. Prod., 2009, 72, 784. CrossRef
78. M. Tadesse, M. B. Strøm, J. Svenson, M. Jaspars, B. F. Milne, V. Tørfoss, J. H. Andersen, E. Hansen, K. Stensvåg, and T. Haug, Org. Lett., 2010, 12, 4752. CrossRef
79. M. Tadesse, J. Svenson, M. Jaspars, M. B. Strøm, M. H. Abdelrahman, J. H. Andersen, E. Hansen, P. E. Kristiansen, K. Stensvåg, and T. Haug, Tetrahedron Lett., 2011, 52, 1804. CrossRef
80. Y. Takahashi, H. Ishiyama, T. Kubota, and J. Kobayashi, Bioorg. Med. Chem. Lett., 2010, 20, 4100. CrossRef
81. R. Finlayson, A. N. Pearce, M. J. Page, M. Kaiser, M.-L. Bourguet-Kondracki, J. L. Harper, V. L. Webb, and B. R. Copp, J. Nat. Prod., 2011, 74, 888. CrossRef
82. A. R. Carroll and V. M. Avery, J. Nat. Prod., 2009, 72, 696. CrossRef
83. D. M. Tapiolas, B. F. Bowden, E. Abou-Mansour, R. H. Willis, J. R. Doyle, A. N. Muirhead, C. Liptrot, L. E. Llewellyn, C. W. W. Wolff, A. D. Wright, and C. A. Motti, J. Nat. Prod., 2009, 72, 1115. CrossRef
84. D. Fehér, R. Barlow, J. McAtee, and T. K. Hemscheidt, J. Nat. Prod., 2010, 73, 1963. CrossRef
85. A. R. Carroll, S. Duffy, M. Sykes, and V. M. Avery, Org. Biomol. Chem., 2011, 9, 604. CrossRef
86. M. Till and M. R. Prinsep, J. Nat. Prod., 2009, 72, 796. CrossRef
87. S. J. Rochfort, S. Moore, C. Craft, N. H. Martin, R. M. Van Wagoner, and J. L. C. Wright, J. Nat. Prod., 2009, 72, 1773. CrossRef
88. M. Tadesse, J. N. Tabudravu, M. Jaspars, M. B. Strøm, E. Hansen, J. H. Andersen, P. E. Kristiansen, and T. Haug, J. Nat. Prod., 2011, 74, 837. CrossRef
89. L. Wen, Z. Guo, Q. Li, D. Zhang, Z. She, and L. L. P. Vrijmoed, Chem. Nat. Compd., 2010, 46, 363. CrossRef
90. P. Zhang, B. Bao, H. T. Dang, J. Hong, H. J. Lee, E. S. Yoo, K. S. Bae, and J. H. Jung, J. Nat. Prod., 2009, 72, 270. CrossRef
91. C.-L. Shao, H.-X. Wu, C.-Y. Wang, Q.-A. Liu, Y. Xu, M.-Y. Wei, P.-Y. Qian, Y.-C. Gu, C.-J. Zheng, Z.-G. She, and Y.-C. Lin, J. Nat. Prod., 2011, 74, 629. CrossRef
92. T. Amagata, K. Takigawa, K. Minoura, and A. Numata, Heterocycles, 2010, 81, 897. CrossRef
93. S. Tsukamoto, T. Kawabata, H. Kato, T.J. Greshock, H. Hirota, T. Ohta, and R. M. Williams, Org. Lett., 2009, 11, 1297. CrossRef
94. T. Yamada, M. Yasuhide, H. Shigeta, A. Numata, and R. Tanaka, J. Antibiot., 2009, 62, 353. CrossRef
95. T. Yamada, Y. Muroga, and R. Tanaka, Mar. Drugs, 2009, 7, 249. CrossRef
96. Y. Muroga, T. Yamada, A. Numata, and R. Tanaka, Helv. Chim. Acta, 2010, 93, 542. CrossRef
97. H. Rahman, B. Austin, W. J. Mitchell, P. C. Morris, D. J. Jamieson, D. R. Adams, A. M. Spragg, and M. Schweizer, Mar. Drugs, 2010, 8, 498. CrossRef
98. W. Fenical, P. R. Jensen, M. A. Palladino, K. S. Lam, G. K. Lloyd, and B. C. Potts, Bioorg. Med. Chem., 2009, 17, 2175. CrossRef
99. R. R. Manam, V. R. Macheria, G. Tsueng, C. W. Dring, J. Weiss, S. T. C. Neuteboom, K. S. Lam, and B. C. Potts, J. Nat. Prod., 2009, 72, 295. CrossRef
100. B. T. Murphy, T. Narender, C. A. Kauffman, M. Woolery, P. R. Jensen, and W. Fenical, Aust. J. Chem., 2010, 63, 929. CrossRef
101. K. N. Maloney, J. B. MacMillan, C. A. Kauffman, P. R. Jensen, A. G. DiPasquale, A. L. Rheingold, and W. Fenical, Org. Lett., 2009, 11, 5422. CrossRef
102. E. H. Andrianasolo, L. Haramaty, R. Rosario-Passapera, K. Bidle, E. White, C. Vetriani, P. Falkowski, and R. Lutz, J. Nat. Prod., 2009, 72, 1216. CrossRef
103. S.-J. Nam, S. P. Gaudêncio, C. A. Kauffman, P. R. Jensen, T. P. Kondratyuk, L. E. Marler, J. M. Pezzuto, and W. Fenical, J. Nat. Prod., 2010, 73, 1080. CrossRef
104. U. W. Hawas, M. Shaaban, K. A. Shaaban, M. Speitling, A. Maier, G. Kelter, H. H. Fiebig, M. Meiners, E. Helmke, and H. Laatsch, J. Nat. Prod., 2009, 72, 2120. CrossRef
105. C. C. Hughes, J. B. MacMillan, S. P. Gaudêncio, P. R. Jensen, and W. Fenical, Angew. Chem. Int. Ed., 2009, 48, 725. CrossRef
106. K. Motohashi, M. Takagi, and K. Shin-ya, J. Nat. Prod., 2010, 73, 226. CrossRef
107. H. C. Kwon, A. P. D. M. Espindola, J.-S. Park, A. Prieto-Davó, M. Rose, P. R. Jensen, and W. Fenical, J. Nat. Prod., 2010, 73, 2047. CrossRef
108. K. C. Pangallo and C. M. Reddy, Environ. Sci. Technol., 2009, 43, 122. CrossRef
109. K. Haraguchi, Y. Hisamichi, Y. Kotaki, Y. Kato, and T. Endo, Environ. Sci. Technol., 2009, 43, 2288. CrossRef
110. C. C. Hughes, A. Prieto-Davo, P. R. Jensen, and W. Fenical, Org. Lett., 2008, 10, 629. CrossRef
111. C. C. Hughes, C. A. Kauffman, P. R. Jensen, and W. Fenical, J. Org. Chem., 2010, 75, 3240. CrossRef
112. N. Rosenfelder, P. Ostrowicz, L. Fu, G. W. Gribble, S. A. Tittlemier, W. Frey, and W. Vetter, J. Chromatogr. A, 2010, 1217, 2050. CrossRef
113. J. Peng, A. R. Place, W. Yoshida, C. Anklin, and M. T. Hamann, J. Am. Chem. Soc., 2010, 132, 3277. CrossRef
114. R. M. Van Wagoner, J. R. Deeds, A. O. Tatters, A. R. Place, C. R. Tomas, and J. L. C. Wright, J. Nat. Prod., 2010, 73, 1360. CrossRef
115. S. Mo, A. Krunic, G. Chlipala, and J. Orjala, J. Nat. Prod., 2009, 72, 894. CrossRef
116. S. Mo, A. Krunic, B. D. Santarsiero, S. G. Franzblau, and J. Orjala, Phytochemistry, 2010, 71, 2116. CrossRef
117. J. I. Jiménez, T. Vansach, W. Y. Yoshida, B. Sakamoto, P. Pörzgen, and F. D. Horgen, J. Nat. Prod., 2009, 72, 1573. CrossRef
118. J. C. Kwan, M. Teplitski, S. P. Gunasekera, V. J. Paul, and H. Luesch, J. Nat. Prod., 2010, 73, 463. CrossRef
119. H. Gross, K. L. McPhail, D. E. Goeger, F. A. Valeriote, and W. H. Gerwick, Phytochemistry, 2010, 71, 1729. CrossRef
120. S. Matthew, L. A. Salvador, P. J. Schupp, V. J. Paul, and H. Luesch, J. Nat. Prod., 2010, 73, 1544. CrossRef
121. B. K. Rubio, S. M. Parrish, W. Yoshida, P. J. Schupp, T. Schils, and P. G. Williams, Tetrahedron Lett., 2010, 51, 6718. CrossRef
122. J. C. Kwan, K. Taori, V. J. Paul, and H. Luesch, Mar. Drugs, 2009, 7, 528. CrossRef
123. T. Teruya, H. Sasaki, H. Fukazawa, and K. Suenaga, Org. Lett., 2009, 11, 5062. CrossRef
124. X. Gao, Y. Liu, S. Kwong, Z. Xu, and T. Ye, Org. Lett., 2010, 12, 3018. CrossRef
125. L. A. Salvador, J. S. Biggs, V. J. Paul, and H. Luesch, J. Nat. Prod., 2011, 74, 917. CrossRef
126. E. Mevers, W.-T. Liu, N. Engene, H. Mohimani, T. Byrum, P. A. Pevzner, P. C. Dorrestein, C. Spadafora, and W. H. Gerwick, J. Nat. Prod., 2011, 74, 928. CrossRef
127. A. Raveh and S. Carmeli, Phytochemistry Lett., 2009, 2, 10. CrossRef
128. S. Gesner-Apter and S. Carmeli, J. Nat. Prod., 2009, 72, 1429. CrossRef
129. R.-B. Volk, U. Girreser, M. Al-Refai, and H. Laatsch, Nat. Prod. Res., 2009, 23, 607. CrossRef
130. B. Vera, A. D. Rodríguez, E. Avilés, and Y. Ishikawa, Eur. J. Org. Chem., 2009, 5327. CrossRef
131. N. Cachet, G. Genta-Jouve, E. L. Regalado, R. Mokrini, P. Amade, G. Culioli, and O. P. Thomas, J. Nat. Prod., 2009, 72, 1612. CrossRef
132. A. J. Zaharenko, G. Picolo, W. A. Ferreira, Jr., T. Murakami, K. Kazuma, M. Hashimoto, Y. Cury, J. C. de Freitas, M. Satake, and K. Konno, J. Nat. Prod., 2011, 74, 378. CrossRef
133. G. Guella, R. Frassanito, I. Mancini, T. Sandron, L. Modeo, F. Verni, F. Dini, and G. Petroni, Eur. J. Org. Chem., 2010, 427. CrossRef
134. B. Dinda, D. R. Chowdhury, and B. C. Mohanta, Chem. Pharm. Bull., 2009, 57, 765. CrossRef
135. P.-C. Wang, J.-M. Hu, X.-H. Ran, Z.-Q. Chen, H.-Z. Jiang, Y.-Q. Liu, J. Zhou, and Y.-X. Zhao, J. Nat. Prod., 2009, 72, 1682. CrossRef
136. S. R. Jensen, C. H. Gotfredsen, U. S. Harput, and I. Saracoglu, J. Nat. Prod., 2010, 73, 1593. CrossRef
137. M. Plovano, J. Garbarine, L. Tomassini, and M. Nicoletti, Nat. Prod. Commun., 2009, 4, 1637.
138. X. Wang, L. Sun, K. Huang, S. Shi, L. Zhang, J. Xu, H. Peng, X. Sun, L. Wang, X. Wu, Y. Zhao, X. Li, J. Stöckigt, and J. Qu, Chem. Biodivers., 2009, 6, 1053. CrossRef
139. L.-S. Gan, Y.-L. Zheng, J.-X. Mo, X. Liu, X.-H. Li, and C.-X. Zhou, J. Nat. Prod., 2009, 72, 1497. CrossRef
140. J. Wen, H. Shi, Z. Xu, H. Chang, C. Jia, K. Zan, Y. Jiang, and P. Tu, J. Nat. Prod., 2010, 73, 67. CrossRef
141. C. Kihampa, M. H. H. Nkunya, C. C. Joseph, S. M. Magesa, A. Hassanali, M. Heydenreich, and E. Kleinpeter, Phytochemistry, 2009, 70, 1233. CrossRef
142. L. Huang, T. Lei, C. Lin, X. Kuang, H. Chen, and H. Zhou, Fitoterapia, 2010, 81, 389. CrossRef
143. K. Sato, Y. Inaba, H.-S. Park, T. Akiyama, T. Koyama, H. Fukaya, Y. Aoyagi, and K. Takeya, Chem. Pharm. Bull., 2009, 57, 668. CrossRef
144. C.-C. Zhu, T.-M. Wang, K.-J. Wang, and N. Li, Z. Naturforsch., 2009, 64b, 1077.
145. M. I. Choudhary, S. Hussain, S. Yousuf, A. Dar, Mudassar, and Atta-ur-Rahman, Phytochemistry, 2010, 71, 2205. CrossRef
146. Y.-X. Xu, Z.-B. Xiang, Y.-S. Jin, Y. Shen, and H.-S. Chen, Fitoterapia, 2010, 81, 920. CrossRef
147. P. Klausmeyer, O. M. Z. Howard, S. M. Shipley, and T. G. McCloud, J. Nat. Prod., 2009, 72, 1369. CrossRef
148. T. Matumoto, T. Hosoya, and H. Shigemori, Heterocycles, 2010, 81, 1231. CrossRef
149. A. Shintani, K. Toume, Y. Yamamoto, and M. Ishibashi, Heterocycles, 2010, 82, 839. CrossRef
150. T. Bunyapaiboonsri, S. Yoiprommarat, K. Intereya, P. Rachtawee, N. L. Hywel-Jones, and M. Isaka, J. Nat. Prod., 2009, 72, 756. CrossRef
151. Y.-L. Yang, W.-Y. Liao, W.-Y. Liu, C.-C. Liaw, C.-N. Shen, Z.-Y. Huang, and S.-H. Wu, Chem. Eur. J., 2009, 15, 11573. CrossRef
152. M. Kawada, I. Momose, T. Someno, G. Tsujiuchi, and D. Ikeda, J. Antibiot., 2009, 62, 243. CrossRef
153. K. Hosono, J. Ogihara, T. Ohdake, and S. Masuda, J. Antibiot., 2009, 62, 571. CrossRef
154. H. Shinonaga, Y. Kawamura, A. Ikeda, M. Aoki, N. Sakai, N. Fujimoto, and A. Kawashima, Tetrahedron Lett., 2009, 50, 108. CrossRef
155. H. Shinonaga, Y. Kawamura, A. Ikeda, M. Aoki, N. Sakai, N. Fujimoto, and A. Kawashima, Tetrahedron, 2009, 65, 3446. CrossRef
156. N. Osmanova, W. Schultze, and N. Ayoub, Phytochem. Rev., 2010, 9, 315. CrossRef
157. S. R. Kingsland and R. A. Barrow, Aust. J. Chem., 2009, 62, 269. CrossRef
158. J.-C. Qin, Y.-M. Zhang, J.-M. Gao, M.-S. Bai, S.-X. Yang, H. Laatsch, and A.-L. Zhang, Bioorg. Med. Chem. Lett., 2009, 19, 1572. CrossRef
159. W. S. Borges, G. Mancilla, D. O. Guimarães, R. Durán-Patrón, I. G. Collado, and M. T. Pupo, J. Nat. Prod., 2011, 74, 1182. CrossRef
160. P. Khumkomkhet, S. Kanokmedhakul, K. Kanokmedhakul, C. Hahnvajanawong, and K. Soytong, J. Nat. Prod., 2009, 72, 1487. CrossRef
161. R. A. Davis, A. R. Carroll, K. T. Andrews, G. M. Boyle, T. L. Tran, P. C. Healy, J. A. Kalaitzis, and R. G. Shivas, Org. Biomol. Chem., 2010, 8, 1785. CrossRef
162. L. Liu, S. Niu, X. Lu, X. Chen, H. Zhang, L. Guo, and Y. Che, Chem. Commun., 2010, 46, 460. CrossRef
163. L. Liu, T. Bruhn, L. Guo, D. C. G. Götz, R. Brun, A. Stich, Y. Che, and G. Bringmann, Chem. Eur. J., 2011, 17, 2604. CrossRef
164. L. Liu, Y. Li, S. Liu, Z. Zheng, X. Chen, H. Zhang, L. Guo, and Y. Che, Org. Lett., 2009, 11, 2836. CrossRef
165. D. C. Hopp, J. Rhea, D. Jacobsen, K. Romari, C. Smith, J. Rabenstein, M. Irigoyen, M. Clarke, L. Francis, M. Luche, G. J. Carr, and U. Mocek, J. Nat. Prod., 2009, 72, 276. CrossRef
166. H. Kikuchi, M. Sekiya, Y. Katou, K. Ueda, T. Kabeya, S. Kurata, and Y. Oshima, Org. Lett., 2009, 11, 1693. CrossRef
167. Z. Guo, L. Shen, Z. Ji, J. Zhang, L. Huang, and W. Wu, J. Antibiot., 2009, 62, 201. CrossRef
168. Z. Guo, Z. Ji, J. Zhang, J. Deng, L. Shen, W. Liu, and W. Wu, J. Antibiot., 2010, 63, 231. CrossRef
169. Z. Guo, Z. Ji, J. Zhang, J. Deng, L. Shen, W. Liu, and W. Wu, J. Antibiot., 2010, 63, 733. CrossRef
170. J. Bitzer, M. Streibel, H.-J. Langer, and S. Grond, Org. Biomol. Chem., 2009, 7, 444. CrossRef
171. X. Yin, Y. Chen, L. Zhang, Y. Wang, and T. M. Zabriskie, J. Nat. Prod., 2010, 73, 583. CrossRef
172. A. Shintani, T. Ohtsuki, Y. Yamamoto, T. Hakamatsuka, N. Kawahara, Y. Goda, and M. Ishibashi, Tetrahedron Lett., 2009, 50, 3189. CrossRef
173. R. W. Fitch, T. F. Spande, H. M. Garraffo, H. J. C. Yeh, and J. W. Daly, J. Nat. Prod., 2010, 73, 331. CrossRef
174. S. W Fox and K. Dose, ‘Molecular Evolution and the Origin of Life,’ Marcel Dekker, New York, 1977.
175. E. L. Teuten and C. M. Reddy, Environ. Pollut., 2007, 145, 668. CrossRef
176. F. M. Schwandner, T. M. Seward, A. P. Grize, P. A. Hall, and V. J. Dietrich, J. Geophys. Res., 2004, 109, D04301. CrossRef
177. G. A. Blake, J. Keene, and T. G. Phillips, Astrophys. J., 1985, 295, 501. CrossRef
178. D. A. Neufeld, J. Zmuidzinas, P. Schilke, and T. G. Phillips, Astrophys. J., 1997, 488, L141. CrossRef
179. G. Winnewisser and E. Herbst, Top. Curr. Chem., 1987, 139, 119. CrossRef
180. F. Keppler and H. Biester, Chemosphere, 2003, 52, 451. CrossRef