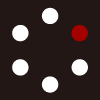
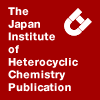
HETEROCYCLES
An International Journal for Reviews and Communications in Heterocyclic ChemistryWeb Edition ISSN: 1881-0942
Published online by The Japan Institute of Heterocyclic Chemistry
e-Journal
Full Text HTML
Received, 28th July, 2011, Accepted, 16th September, 2011, Published online, 26th September, 2011.
DOI: 10.3987/COM-11-S(P)87
■ Diastereoselective Synthesis of (±)-Deethyleburnamonine Using a Catalytic Cyclopropane Ring-Opening / Friedel-Crafts Alkylation Strategy
Dadasaheb V. Patil, Marchello A. Cavitt, and Stefan France*
School of Chemistry and Biochemistry, Georgia Institute of Technology, 901 Atlantic Drive, Atlanta, Georgia 30332-0400, U.S.A.
Abstract
A short, diastereoselective synthesis of (±)-deethyleburnamonine is reported with an overall yield of ~18% over six steps. The key synthetic step involves an indium(III)-catalyzed tandem ring-opening/Friedel-Crafts alkylation of a donor-acceptor-acceptor amino cyclopropane to generate the ABDE portion of the target.The eburnan1 alkaloids and the structurally-related tacaman2 alkaloids represent a large group of biologically-active, naturally-occurring indole alkaloids that are isolated from several plants of the Apocyanaceae and Tabernaemontana genera (Figure 1). Each member of the eburnan and tacaman families is characterized by a common pentacyclic framework (1) that contains either a cis- or trans-fused D/E ring system.3 While the vast majority of compounds have a cis-fused D/E ring system, several important biologically-active derivatives, such as vindeburnol (2), possess a trans-fused junction. The eburnan skeleton has an ethyl group at C(20), while the tacaman skeleton has the ethyl group at C(14) instead.4 Many of these compounds exhibit a variety of pharmacological activities, ranging from antitumor activity to muscle-stimulating activity.5 Over the past 10 years, numerous efforts to fully understand the compounds’ modulatory effects on brain circulation and neuronal homeostasis have been reported.6 For example, vincamine (3) has been shown to have neuroprotective effects resulting from the blockage of voltage-gated sodium ion channels. Similarly, vinpocetine (5), the most extensively studied cogener of the eburnan class, is currently prescribed in Europe (tradename: Cavinton) for the treatment of disorders arising from cerebrovascular and cerebral neurodegenerative diseases.7 This therapeutic potential has led to intense pharmacological and synthetic studies over the past several decades.8
Of the synthetic strategies that have been reported for the assembly of the pentacyclic core, the most common approaches involve preparation of the ABCD ring system, with subsequent E-ring formation. The ABCD ring system is most often prepared by one of the following methods: (1) a Pictet-Spengler/Bischler-Napieralski cyclization;9 (2) a Michael-type annulation;10 or (3) annulation reactions of dihydro-β-carboline derivatives.11 Recently, Padwa published an alternate route to the eburnan and tacaman alkaloids that involves a Rh(II)-catalyzed intramolecular dipolar cycloaddition of an α-diazo indoloamide, followed by reductive ring-opening and base-induced keto-amide ring contraction to build the full ADCDE skeleton.12
In contrast to previous reports, our approach to the eburnan and tacaman alkaloids involves the initial assembly of the ABDE ring and stems from our recent success in the tandem ring-opening/intramolecular π cyclizations of donor-acceptor cyclopropanes, or homo-Nazarov cyclizations13 (Scheme 1).14 These reactions involve the acid-promoted heterolytic ring-opening of a donor-acceptor cyclopropane to generate an acyclic cation, which is trapped intramolecularly by an adjacent π system to generate a six-membered cyclic oxyallyl cation. Deprotonation followed by tautomerization then affords the cyclohexenone product. We demonstrated that In(OTf)3 (30 mol %) successfully catalyzes the cyclizations of alkenyl cyclopropyl ketones and cyclopropyl heteroaryl ketones to form the functionalized cyclohexyl rings.15 The use of the donor-acceptor cyclopropanes bearing a secondary electron acceptor (an ester group) is essential, as it permits effective catalysis under mild reaction conditions. We recently disclosed an efficient method for the facile construction of 6,7,8,9-tetrahydropyrido[1,2-a]indole derivatives 9 in good to excellent yields (up to > 99%) using a tandem ring-opening/Friedel-Crafts alkylation strategy (Scheme 2).16 The methodology is highly modular, operationally simple and amenable to a large variety of functional groups and substitution patterns.
Encouraged by these results, we reasoned that the optimized method could be applied to the construction of the pentacyclic framework of the eburnan and tacaman classes of alkaloids, both of which possess the 6,7,8,9-tetrahydropyrido[1,2-a]indole ring system. For instance, the tetracyclic ABDE system was readily assembled from aminocyclopropane 10 using our method (Scheme 3). Under the standard reaction conditions, 10 provides the desired tetracyclic hydropyrido[1,2-a]indole based product 11 in 97% yield. It is important to note that the D/E ring contains only the 20,21-cis-ring junction with 11 being formed with a 7.5:1 diastereomeric preference for the all-cis isomer. This result led us to choose (±)-deethyleburnamonine (12) as an initial synthetic target because it represents the simplest example of both the alkaloid classes (no ethyl group present). Moreover, the compound has interesting pharmacological properties.17 (±)-Deethyleburnamonine was most recently synthesized by Lounasmaa in nine steps with an overall yield of ~18-20%.18 In this report, we describe a short, diastereoselective approach to (±)-deethyleburnamonine using a catalytic tandem ring-opening/Friedel-Crafts alkylation protocol developed in our laboratory.
Our retrosynthetic approach is outlined in Scheme 4. We envisioned that (±)-dieethyleburnamonine would arise from the ABDE tetracycle 13 following N-deprotection, N-alkylation (C-ring formation), and decarbalkoxylation. Tetracycle 13 would be generated from the N-acylated indolyl cyclopropane 14 via our indium-catalyzed tandem cyclization.
Success of the proposed route is predicated on facile access to cyclopropane 14. Synthesis of 14 is readily achievable in three steps (Scheme 5). N-Acylation of commercially-available 3-(2-bromoethyl)- 1H-indole provided the required β-ester amide 15 in 84% yield. Next, diazo transfer with tosyl azide provided the α-diazo ester 16 in 88% yield. Subsequent rhodium-catalyzed cyclopropanation in the presence of the Cbz-protected cyclic enamine 17 afforded the requisite cyclopropane 14 in 81% yield. Upon treatment with In(OTf)3, cyclopropane 14 readily cyclized to form tetracycle 13 in 97% yield. The major product formed was the all-cis diastereomer with 8:1 dr. With 13 in hand, we anticipated that C-ring formation (N-alkylation) should be facile upon Cbz-deprotection. Unfortunately, the desired product was not observed when a variety of deprotection conditions were attempted, including hydrogenation.
To alleviate this issue, the Boc-protected enamine 18 was employed, which afforded cyclopropane 19 in 49% yield. The lower yield observed for the Boc-protected enamine is most likely due to the increased steric interference in the transition state between the t-Bu group of the enamine and the multi-dentate esp ligand of the rhodium carbenoid during cyclopropanation. Cyclization of 19 similarly provided the all-cis diasteromer 20 (~3:1 dr) but with less efficiency than the Cbz case (97% vs 71%), which may also be a result of the steric influence of the Boc t-butyl group. Fortunately, when 20 was subjected to TFA, both deprotection and C-ring closure occurred, generating 21 in 87% yield. Finally, Krapcho decarbalkoxylation19 provided (±)-deethyleburnamonine (12) in 85% yield.
In summary, we report a short, diastereoselective total synthesis of (±)-deethyleburnamonine. The key steps of the synthesis involve: (a) a tandem ring-opening/Friedel-Crafts alkylation to assemble the tetracyclic ABDE ring system with a cis D/E fused ring junction; (b) a TFA-promoted N-Boc deprotection/N-alkylation to generate the C-ring; and (3) a Krapcho decarbalkoxylation to generate the target. Using this protocol, (±)-deethyleburnamonine was obtained in 18% overall yield over six steps. Efforts towards the synthesis of other members of the eburnan and tacaman classes are currently underway and will be reported in due course.
EXPERIMENTAL
All reactions were carried out in pre-dried glassware from the oven where additional moisture was removed by flame drying the reaction vessel. Each reaction proceeded under a nitrogen atmosphere, and dry solvents were used, unless stated otherwise. Tetrahydrofuran and diethyl ether were distilled from a sodium/benzophenone ketyl under nitrogen and stored in a Schlenk flask. Acetonitrile and dichloromethane were purified by distillation from CaH2 under N2 prior to use. All other reagents were purchased from Acros, Sigma-Aldrich, Fluka, VWR, Merck, Alfa Aesar, TCI and Strem (for metal catalysts) and used without further purification. Chromatographic purification was performed as flash chromatography with Dynamic Adsorbents silica gel (32-65 μm) and solvents indicated as eluent with 0.1-0.5 bar pressure. For quantitative flash chromatography, technical grade solvents were utilized. Analytical thin-layer chromatography (TLC) was performed on EMD silica gel 60 F254 TLC glass plates. Visualization was accomplished with UV light, aqueous basic potassium permanganate (KMnO4) solution, iodine, aqueous acidic dinitrophenylhydrazine (DNP) solution, aqueous acidic p-anisaldehyde (PAA) solution, and ethanol solution of phosphomolybdic acid (PMA) followed by heating. Each yield refers to isolated analytically pure material. Infrared (IR) spectra were obtained using a Nicolet 4700 FTIR with an ATR attachment from SmartOrbit Thermoelectronic Corp. The IR bands are characterized as weak (w), medium (m), and strong (s). Proton and carbon nuclear magnetic resonance spectra (1H NMR and 13C NMR) were recorded on a Varian Mercury Vx 300 spectrometer or a Varian Mercury Vx 400 spectrometer with solvent resonances as the internal standard (1H NMR: CDCl3 at 7.26 ppm; 13C NMR: CDCl3 at 77.0 ppm). 1H NMR data are reported as follows: chemical shift (ppm), multiplicity (s = singlet, d = doublet, dd = doublet of doublets, dt = doublet of triplets, ddd = doublet of doublet of doublets, t = triplet, m = multiplet), coupling constants (Hz), and integration. Mass spectra were obtained using a VG-70SE instrument.
Preparation of N-Cbz-protected aminocyclopropane (14)
In a round bottom flask charged with a magnetic stir bar, Rh2esp2 (1.4 mg, 1.87 μmol) was dissolved in CH2Cl2 (6 mL). The reaction vessel was cooled to 0 ˚C and benzyl 3,4-dihydropyridine-1(2H)- carboxylate (17, 0.037 g, 0.187 mmol) was added. After 10 min, 3-(3-(2-bromoethyl)-1H-indol-1-yl)- 2-diazo-3-oxopropanoate (16, 0.085 g, 0.243 mmol) was dissolved in CH2Cl2 (2 mL) and syringed into the reaction mixture. After 10 min, the ice bath was removed and the reaction proceeded at room temperature. After 12 h, the solution was quenched with saturated thiourea and stirred for 30 min. The organic layer was separated and the aqueous layer was extracted three times with CH2Cl2. The combined organic layers were washed with brine, dried over Na2SO4, and concentrated under reduced pressure. The residue was purified by silica gel flash chromatography (30% EtOAc/Hexanes, Rf 0.25) to afford 14 as a light brown oil (0.081 g, 81%). (Rotamers!!!) 1H NMR (300 MHz, CDCl3) δ 8.47 (d, J = 8.2 Hz, 1H), 7.57 – 7.28 (m, 8H), 7.05 (d, J = 43.0 Hz, 1H), 5.19 (d, J = 3.2 Hz, 2H), 4.74 (d, J = 21.7 Hz, 1H), 3.79 (d, J = 3.3 Hz, 3H), 3.71 – 3.51 (m, 4H), 3.35 – 3.10 (m, 3H), 2.46 – 2.25 (m, 1H), 2.25 – 2.01 (m, 1H), 2.00 – 1.76 (m, 2H). 13C NMR (75 MHz, CDCl3) δ 168.4, 168.3, 167.3, 167.2, 165.7, 165.7, 165.6, 165.5, 153.5, 152.9, 136.0, 135.9, 130.3, 130.1, 130.0, 128.5, 128.3, 128.2, 128.1, 128.0, 127.9, 126.8, 126.5, 126.3, 125.9, 125.7, 124.3, 124.1, 123.3, 123.1, 122.9, 122.4, 122.2, 120.4, 119.7, 119.2, 119.1, 118.6, 117.4, 117.1, 116.9, 116.7, 111.4, 111.3, 110.7, 110.6, 67.8, 67.7, 58.0, 57.9, 57.7, 53.4, 52.9, 52.9, 52.8, 43.3, 42.1, 41.9, 38.8, 38.7, 31.3, 30.9, 28.6, 28.4, 23.7, 23.2, 23.0, 22.9, 21.2, 19.3, 19.2. IR: 2951.9 (w), 2928.1 (w), 2847.1 (w), 1761.3 (s), 1703.26 (s), 1451.7 (s), 1370.7 (m), 1275.5 (m), 1218.3 (m), 1170.7 (m), 742.1 (s) cm-1. HRMS (ESI) M/Z+ Calc. 538.1103, Obs. 538.1094.
Preparation of N-Cbz-protected ABDE tetracycle (13)
2-Benzyl 7-methyl 7-(3-(2-bromoethyl)-1H-indole-1-carbonyl)-2-azabicyclo[4.1.0]heptane-2,7-dicarboxylate (15, 0.045 g, 0.083 mmol) was added to a solution of In(OTf)3 (0.014 g, 0.025 mmol) in CH2Cl2 (3 mL) at room temperature. Upon completion as monitored by TLC, the reaction mixture was quenched with water and extracted three times with CH2Cl2. The combined organic layers were washed with brine, dried over Na2SO4 and concentrated under reduced pressure. The residue was purified by silica gel flash column chromatography (25% EtOAc/Hexanes, Rf 0.25) to afford 13 as a pale brown oil (0.098 g, 98%) after 2 h. Diastereomeric ratio: (8:1). 1H NMR (300 MHz, CDCl3) δ 8.41 (dd, J = 14.5, 6.9 Hz, 1.07), 7.50 – 7.19 (m, 9.14), 5.93 (dd, J = 17.0, 4.6 Hz, 1), 5.30 – 5.06 (m, 2.54), 4.09 (d, J = 13.2 Hz, 1.16), 3.92 – 3.57 (m, 4.64), 3.56 – 2.76 (m, 5.17), 2.75 – 2.48 (m, 2.28), 1.77 (d, J = 10.4 Hz, 1.25), 1.53 (s, 2.17), 1.43 – 1.26 (m, 1.08). 13C NMR (75 MHz, CDCl3) δ 168.1, 162.8, 155.1, 136.0, 134.7, 130.2, 130.0, 129.1, 129.0, 128.5, 128.2, 128.1, 125.6, 125.5, 124.4, 124.3, 117.9, 117.8, 117.0, 116.7, 116.5, 68.2, 68.1, 55.9, 53.1, 52.5, 48.3, 42.5, 39.7, 37.7, 30.0, 27.2, 27.5, 26.6, 24.6. IR: 2950.4 (w), 2910.7 (w), 2880.6 (w), 1755.7 (s), 1719.2 (s), 1442.8 (m), 1275.6 (m), 758.2 (s) cm-1. HRMS (ESI) M/Z+ Calc. 538.1103, Obs. 538.1105.
Preparation of N-Boc-protected aminocyclopropane (19)
In a round bottom flask charged with a magnetic stir bar, Rh2esp2 (1.0 mg, 1.319 μmol) was dissolved in CH2Cl2 (15 mL). The reaction vessel was cooled to 0 ˚C and tert-butyl 3,4-dihydropyridine-1(2H)- carboxylate (17, 0.201 g, 1.098 mmol) was added. After 10 min, methyl 3-(3-(2-bromoethyl)-1H-indol-1- yl)-2-diazo-3-oxopropanoate (16, 0.500 g, 1.422 mmol) was dissolved in CH2Cl2 (5 mL) and syringed into the reaction mixture. After 10 min, the ice bath was removed and the reaction proceeded at room temperature. After 12 h, the solution was quenched with saturated aqueous thiourea and stirred for 30 min. The organic layer was separated and the aqueous layer was extracted three times with CH2Cl2. The combined organic layers were washed with brine, dried with Na2SO4, and concentrated under reduced pressure. The residue was purified by silica gel flash chromatography (30% EtOAc/Hexanes, Rf 0.25) afforded 19 as a colorless oil (0.271 g, 49%). 1H NMR (300 MHz, CDCl3) δ 8.47 (d, J = 8.1 Hz, 1H), 7.56 – 7.45 (m, 1H), 7.43 – 7.28 (m, 2H), 6.98 (d, J = 66.6 Hz, 1H), 4.74 (d, J = 9.4 Hz, 1H), 3.79 (d, J = 3.8 Hz, 3H), 3.70 – 3.35 (m, 4H), 3.27 (t, J = 6.9 Hz, 2H), 3.17 (t, J = 6.8 Hz, 1H), 2.45 – 2.04 (m, 2H), 1.93 – 1.76 (m, 2H), 1.47 (d, J = 7.5 Hz, 9H). 13C NMR (75 MHz, CDCl3) δ 168.6, 165.8, 152.6, 151.8, 136.1, 129.9, 129.8, 127.9, 126.9, 126.7, 125.8, 124.1, 122.2, 121.9, 120.3, 119.6, 118.4, 117.1, 110.1, 109.3, 81.2, 81.1, 58.2, 52.9, 43.3, 42.2, 41.1, 34.6, 31.6, 31.2, 28.5, 28.4, 28.2, 25.3, 23.7, 23.0, 22.6, 21.4, 14.1. IR: 2998 (w), 2942.3 (w), 1725.2 (s), 1628.9 (s), 1468.9 (s), 1342.1 (s), 1185.6 (s), 760.4 (s) cm-1. HRMS (ESI) M/Z+ Calc. 504.1300, Obs. 504.1251.
Preparation of N-Boc-protected ABDE tetracycle (20)
2-tert-Butyl 7-methyl 7-(3-(2-bromoethyl)-1H-indole-1-carbonyl)-2-azabicyclo[4.1.0]heptane-2,7- dicarboxylate (19, 0.075 g, 0.148 mmol) was added to a solution of In(OTf)3 (0.030 g, 0.053 mmol) in CH2Cl2 (10 mL) at room temperature. Upon completion as determined by TLC, the reaction mixture was quenched with water and extracted three times with CH2Cl2. The combined organic layers were washed with brine, dried over Na2SO4 and concentrated under reduced pressure. The residue was purified by silica gel flash column chromatography (25% EtOAc/Hexanes, Rf 0.30) to afford 20 as a colorless oil (0.053 g, 71%) after 3 h. Diastereomeric ratio: (3.2:1). 1H NMR (300 MHz, CDCl3) δ 8.55 – 8.43 (m, 1.11H), 7.57 – 7.49 (m, 1.16), 7.42 – 7.30 (m, 2.5), 5.97 (s, 1), 4.05 (t, J = 11.6 Hz, 090), 3.84 (s, 1), 3.76 (d, J = 3.9 Hz, 2.87), 3.73 – 3.63 (m, 1.17), 3.52 (dt, J = 20.5, 12.0 Hz, 2.33), 3.33 – 3.05 (m, 2.64), 2.65 (d, J = 12.1 Hz, 2.39), 2.29 (d, J = 10.6 Hz, 0.22), 1.86 (d, J = 13.0 Hz, 1), 1.69 – 1.49 (m, 13.63). 13C NMR (75 MHz, CDCl3) 168.2, 167.5, 163.0, 154.6, 154.3, 134.7, 134.5, 130.3, 130.1, 130.0, 129.7, 129.5, 125.4, 124.4, 124.2, 118.0, 117.9, 117.8, 116.7, 116.5, 81.1, 81.1, 56.0, 53.6, 53.1, 52.5, 50.2, 42.5, 40.3, 39.9, 37.6, 29.9, 28.4, 28.3, 27.5, 26.8, 24.7, 24.4, 22.8. IR: 2997.9 (w), 2961.3 (w), 1766.3 (m), 1726.7 (s), 1469.1 (s), 1186.1 (m), 760.3 (s), 663.1 (m) cm-1. HRMS (ESI) M/Z+ Calc. 504.1300, Obs. 504.1255.
Preparation of N-Boc-protected pentacycle (21)
Tetracycle 20 (0.030 g, 0.059 mmol) was dissolved in trifluoroacetic acid (2 mL) and stirred for 2 h. Saturated aqueous NaHCO3 was slowly added to quench the reaction. The resulting mixture was extracted three times with CH2Cl2, washed with brine, dried over MgSO4, and concentrated under reduced pressure. The residue was purified by silica gel flash chromatography (1.5% MeOH/CH2Cl2, Rf 0.40) to afford 21 as a colorless oil (0.0168 g, 87% yield). Diastereomeric ratio: (5:1). 1H NMR (300 MHz, CDCl3) δ 8.41 – 8.30 (m, 1.11), 7.46 – 7.40 (m, 1.27), 7.36 – 7.25 (m, 2.45), 4.46 – 4.34 (m, 1.11), 3.84 (s, 0.60), 3.75 (d, J = 3.8 Hz, 3.24), 3.68 – 3.59 (m, 9.42), 3.36 – 3.28 (m, 2.61), 2.98 – 2.74 (m, 3.24), 2.68 – 2.33 (m, 4.88), 1.65 (ddd, J = 10.6, 9.2, 7.6 Hz, 4.01). 13C NMR (75 MHz, CDCl3) δ 168.6, 167.4, 164.0, 160.9, 135.3, 135.0, 128.4, 128.3, 127.7, 127.5, 126.9, 126.4, 124.8, 124.0, 122.1, 120.9, 120.3, 118.8, 117.2, 116.7, 112.6, 104.9, 77.4, 77.0, 76.6, 70.3, 55.3, 53.6, 53.1, 49.5, 48.4, 44.2, 35.8, 33.8, 32.4, 29.6, 27.0, 24.8, 16.1, 14.6. HRMS (ESI) M/Z+ Calc. 324.1434, Obs. 324.1470.
Preparation of (±)-deethyleburnamonine (12)
To a 10 mL round bottom flask equipped with a stir bar, β-amide ester (21, 0.050 g, 0.154 mmol), NaCl (9.46 mg, 0.161 mmol), water (5.55 µL, 0.308 mmol) and DMSO (3 mL) were added at room temperature. The flask was fitted with a reflux condenser and heated to 150 ˚C with vigorous stirring. After heating for 16 h, TLC analysis indicated consumption of starting material. The reaction was cooled and diluted with 7:3 Hexanes/Et2O (25 mL) and washed three times with water. The organic layer was dried over MgSO4 and concentrated under reduced pressure. The residue was purified by silica gel flash chromatography (1.5% MeOH/CH2Cl2, Rf 0.40) to afford 12 as a white solid (0.0349 g, 85% yield). 1H NMR (300 MHz, CDCl3) δ 7.46 – 7.41 (m, 1H), 7.36 – 7.27 (m, 2H), 4.40 – 4.30 (m, 1H), 3.39 – 3.29 (m, 2H), 3.02 – 2.83 (m, 2H), 2.72 – 2.57 (m, 3H), 2.46 (qdd, J = 10.9, 5.3, 3.1 Hz, 3H), 1.69 – 1.55 (m, 3H), 1.00 – 0.81 (m, 1H). 13C NMR (75 MHz, CDCl3) δ 167.3, 134.3, 131.3, 129.8, 124.3, 123.8, 118.0, 116.2, 112.7, 53.4, 50.4, 44.5, 39.7, 34.3, 25.3, 24.7, 16.3. The physical characterization of the product matches the previously reported data in the literature (see ref. 17).
ACKNOWLEDGEMENTS
S. F. thanks the NSF FACES Program for a Career Initiation Grant and ORAU for the Ralph E. Powe Junior Faculty Enhancement Award. M. A. C. thanks the Ford Foundation (Diversity Fellowship), the NSF (Graduate Research Fellowship), and Georgia Tech (Presidential Fellowship) for generous support.
References
1. C. S. Szántay and A. Nemes, “The Indoles: the monoterpenoid indole alkaloids”, ed. by J. E. Saxton, John Wiley & Sons, New York, 1994, pp. 437-486; M. Lounasmaa and A. Tolvanen, “The Alkaloids”, ed. by G. A. Cordell, Vol. 42, Academic Press, New York, 1992, pp. 1-116.
2. T. A. Van Beek, R. Verpoorte, and A. B. Svendsen, Tetrahedron, 1984, 40, 737; CrossRef T. A. Van Beek, P. P. Lankhorst, R. Verpoorte, and A. B. Svendsen, Tetrahedron Lett., 1982, 23, 4827. CrossRef
3. M. P. Cava, S. S. Tjoa, Q. A. Ahmed, and A. I. Da Rocha, J. Org. Chem., 1968, 33, 1055. CrossRef
4. For the biogenetic numbering system used in this paper, see: J. Le Men and W. I. Taylor, Experientia, 1965, 21, 508. CrossRef
5. “The Vinca Alkaloids”, ed. by W. I. Taylor and N. R. Farnsworth, Marcel Dekker, New York, 1973.
6. A. Vas and B. Gulyas, Med. Res. Rev., 2005, 25, 737. CrossRef
7. A. E. Medina, PNAS, 2010, 107, 9921; CrossRef K.-I. Jeon, X. Xu, T. Aizawa, J. H. Lim, H. Jono, D.-S. Kwon, J.-I. Abe, B. C. Berk, J.-D. Li, and C. Yan, PNAS, 2010, 107, 9795. CrossRef
8. For leading references, see: D. B. England and A. Padwa, J. Org. Chem., 2008, 73, 2792. CrossRef
9. For representative examples of ABCD ring formation using Pictet-Spengler/Bischler-Napieralski cyclizations, see: J. L. Herrmann, R. J. Cregge, J. E. Richman, C. L. Semmelhack, and R. H. Schlessinger, J. Am. Chem. Soc., 1974, 96, 3702; CrossRef P. Pfaffli, W. Oppolzer, R. Wenger, and H. Hauth, Helv. Chim. Acta, 1975, 58, 1131; CrossRef Y. Langlois, A. Pouilhes, D. Genin, R. Z. Andriamialisoa, and N. Langlois, Tetrahedron, 1983, 39, 3755; CrossRef M. Lounasmaa and A. Tolvanen, J. Org. Chem., 1990, 55, 4044. CrossRef
10. For representative examples of ABCD ring formation using Michael-type alkylation, see: G. Rossey, A. Wick, and E. Wenkert, J. Org. Chem., 1982, 47, 4745; CrossRef L. Szabó, J. Sápi, G. Kalaus, G. Argay, A. Kálmán, E. Baitz-Gács, J. Tamás, and C. Szántay, Tetrahedron, 1983, 39, 3737; CrossRef A. Nemes, L. Czibula, G. Visky, M. Farkas, and J. Kreidl, Heterocycles, 1991, 32, 2329. CrossRef
11. For representative examples of ABCD ring formation using annulation reactions, see: G. Hugel, J. Levy, and J. Le Men, C. R. Acad. Sci., Ser. C, 1972, 274, 1350; W. Oppolzer, H. Hauth, P. Pfaffli, and R. Wenger, Helv. Chim. Acta, 1977, 60, 1801; CrossRef B. Danieli, G. Lesma, G. Palmisano, and B. Gabetta, J. Chem. Soc., Chem. Commun., 1981, 908. CrossRef
12. D. B. England and A. Padwa, Org. Lett., 2007, 9, 3249. CrossRef
13. For a review on the homo-Nazarov cyclization, see: F. De Simone and J. Waser, Chimia, 2009, 63, 162. CrossRef
14. While the term “homo-Nazarov” cyclization is used in the literature to describe this type of transformation, it is somewhat misleading since it describes the resulting product (or reaction intermediate) and not the mechanism of the reaction. Thus, we describe the reactions as tandem ring-opening/intramolecular π cyclizations.
15. D. V. Patil, L. H. Phun, and S. France, Org. Lett., 2010, 12, 5684; CrossRef L. H. Phun, D. V. Patil, M. A. Cavitt, and S. France, Org. Lett., 2011, 13, 1952. CrossRef
16. D. V. Patil, M. A. Cavitt, P. Grzybowski, and S. France, Chem. Commun., 2011, 47, 10278. CrossRef
17. A. R. Stoit and U. K. Pandit, Tetrahedron, 1989, 45, 849; CrossRef L. Szporny, Actual Pharm., 1977, 29, 87.
18. M. Lounasmaa, D. D. Belle, and A. Tolvanen, Heterocycles, 1999, 51, 1125; CrossRef M. Lounasmaa, M. Berner, M. Brunner, H. Suomalainen, and A. Tolvanen, Tetrahedron, 1998, 54, 10205; CrossRef M. Lounasmaa, L. Miikki, and A. Tolvanen, Tetrahedron, 1996, 52, 9925; CrossRef A. R. Stoit and U. K. Pandit, Tetrahedron, 1989, 45, 849; CrossRef H. P. Husson, T. Imbert, C. Thal, and P. Potier, Bull. Soc. Chim. Fr., 1973, 2013.
19. A. P. Krapcho, E. G. E. Jahngen, Jr., A. J. Lovey, and F. W. Short, Tetrahedron Lett., 1974, 1091. CrossRef