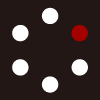
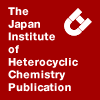
HETEROCYCLES
An International Journal for Reviews and Communications in Heterocyclic ChemistryWeb Edition ISSN: 1881-0942
Published online by The Japan Institute of Heterocyclic Chemistry
e-Journal
Full Text HTML
Received, 30th July, 2011, Accepted, 5th September, 2011, Published online, 12th September, 2011.
DOI: 10.3987/COM-11-S(P)91
■ Synthesis of Oxacalix[2]m-terphenyl[2]triazine and Its Functionalizations
Muhammad Moazzam Naseer, De-Xian Wang, and Mei-Xiang Wang*
Beijing National Laboratory for Molecular Sciences, CAS Key Laboratory of Molecular Recognition and Function, Institute of Chemistry, Chinese Academy of Sciences, Beijing 100190, China
Abstract
Oxacalix[2]m-terphenyl[2]triazine, a macrocyclic host molecule of an expanded cavity, was synthesized from 1,3-(4-hydroxyphenyl)benzene and cyanuric chloride using the fragment coupling strategy. Aromatic nucleophilic substitution reaction of chlorotriazine moieties with di(2-hydroxyethyl)amine and di(2-pyridylmethyl)amine afforded the corresponding upper-rim functionalized oxacalix[2]m-terphenyl[2]triazines in good yields.Heteroatom-bridged calixaromatics or heteracalixaromatics are an emerging generation of macrocyclic host molecules.1 In contrast to conventional calixarenes,2 the bridging heteroatoms can adopt different electronic configurations and form various conjugation systems with their adjacent aromatic rings, producing resultantly the fine-tuned conformational and cavity structures.3 Furthermore, the electronic feature of the macrocyclic cavity is also subjected to regulation by the interplay between linking heteroatoms and aromatic rings.4 Unique structural properties have rendered heteracalixaromatics versatile macrocyclic host molecules in the study of molecular recognition and supramolecular self-assembly.1,4-7
We developed a few years ago the fragment coupling approach for the synthesis of azacalix[m]arene[n]pyridines6a and of oxygen- and nitrogen-bridged calix[2]arene[2]triazines3a starting from simple and readily available aromatic dielectrophilic and dinucleophilic reactants. A large number of heteracalixaromatics have been prepared since then either through the step-wise fragment coupling method or the one-pot synthesis protocol.1,8 To construct heteracalixaromatics of expanded cavity in order to selectively include larger volume guest species, one of the strategies involves the employment of fused (hetero)aromatic rings instead of benzene or six-membered heteroarene fragments. For example, Katz and his co-workers have demonstrated successfully the formation of oxacalix[2]naphthalene[2]naphthyridine from the reaction of 2,7-dihydroxynaphthalene with 2,7-dichloro-1,8-naphthyridine.9 We envisioned that, in addition to fused (hetero)aromatic rings, conformationally rigid or shape-persistent diol compounds such as 1,3-bis(4-hydroxyphenyl)benzene would serve as a large size dinucleophilic component in the construction of heteracalixaromatics of a giant cavity. We report herein the efficient synthesis of oxacalix[2]m-terphenyl[2]triazine and its functionalization.
Based on the fragment coupling strategy,3a,6a we first prepared a linear trimeric intermediate 3. In the presence of diisopropylethylamine (DIPEA) as an acid scavenger, 1,3-bis(4-hydroxyphenyl)benzene 1, which was obtained from the Suzuki coupling reaction between 1,3-dibromobenzene and 4-methoxyphenylboronic acid followed by demethylation reaction,10 reacted with an excess amount of cyanuric chloride 2 smoothly in THF at 0 oC to afford 3 in 79% yield (Scheme 1). The synthesis of oxacalix[2]m-terphenyl[2]triazine 4 was then attempted under different conditions (Table 1). As assembled in Table 1, the synthesis of macrocycle 4 was strongly determined by both the base and the solvent employed. When reaction was conducted in chloroform, for example, the use of inorganic bases including Na2CO3, K2CO3 and Cs2CO3 gave only a trace amount of the desired product 4 (entries 1 to 3). Surprisingly, triethylamine as a base in chloroform had a detrimental effect on the formation of product 4 (entry 4), whereas DIPEA acted as an effective base to promote the formation of oxacalix[2]m-terphenyl[2]triazine 4 in 65% yield (entry 5). It seemed that the use of a sterically bulky and non-nucleophilic base such as DIPEA is beneficial for the macrocyclization. Change of organic solvent from chloroform to 1,4-dioxane and tetrahydrofuran (THF) resulted in very sluggish reaction and very low yields (entries 6 and 7). The combination of DIPEA with acetone (entry 8) or acetonitrile (entry 9) appeared almost equally effective as that of DIPEA with chloroform, albeit the slightly diminished yields were observed.
Having had macrocyclic product in hand, we then examined its conversion into functionalized heteracalixaromatics. It is well established that all three chloro groups of cyanuric chloride can be consecutively and selectively substituted by various nucleophiles under different conditions.3a Taking the advantage of the reactivity of chlorotriazine within macrocycle 4 towards nucleophiles, aromatic nucleophilic displacement of chloro by amines was executed. Pleasingly, the reaction of 4 with functionalized amines such as di(2-hydroxyethyl)amine 5a and di(2-pyridylmethyl)amine 5b proceeded efficiently at room temperature with the aid of K2CO3. The oxacalix[2]m-terphenyl[2]triazines 6a and 6b, which are upper-rim functionalized with hydrogen bond donors and chelating groups, were isolated in 81% and 77% yield, respectively (Scheme 2).
The structure of all oxacalix[2]m-terphenyl[2]triazine products 4 and 6 were established on the basis of spectroscopic data and microanalysis. It is interesting to note that there is only one set of proton and carbon signals being observed in each of their 1H and 13C NMR spectra, respectively. It indicates convincingly that the macrocyclic structures are very fluxional in solution and interconversions of different conformational structures take place rapidly at ambient temperature relative to the NMR time scale.
The macrocyclic structure was determined unambiguously by the X-ray single crystal structure of oxacalix[2]m-terphenyl[2]triazine 4. Several structural features are worth addressing. In the solid state, compound 4 adopts roughly 1,3-alternate conformation, similar to most of the heteracalix[2]arene[2]triazines reported to date.1 While two triazine rings, which are in conjugation with bridging oxygen atoms as indicated by the bond lengths (see caption of Figure 1), are nearly edge-to-edge orientated, two central benzene rings of two m-terphenyl units tend to face-to-face paralleled. Benzene rings connected to bridging oxygen atoms are almost perpendicular to the triazine ring. The upper rim distance between two triazine rings and two central benzene rings are 11.2 Å and 4.1 Å, respectively, forming a large rectangular cavity.
In conclusion, we have synthesized oxacalix[2]m-terphenyl[2]triazine, a heteracalixaromatics of expanded cavity, from the reaction of 1,3-bis(4-hydroxyphenyl)benzene with cyanuric chloride on the basis of the fragment coupling approach. Aromatic nucleophilic substitution reaction of chlorotriazine with di(2-hydroxyethyl)amine and di(2-pyridylmethyl)amine led to the functionalized macrocyclic host molecules. The study of their molecular recognition behaviors is actively pursued in the laboratory and will be reported in due course.
EXPERIMENTAL
Starting Materials. 1,3-Bis(4-hydroxyphenyl)benzene 110 was prepared according to literature procedures, and cyanuric chloride 2 and di(2-hydroxyethyl)amine 5a were purchased from local chemical suppliers.
Typical Procedure for the Preparation of Intermediate 3. To an ice-bath cooled solution of cyanuric chloride 2 (1.38 g, 7.5 mmol) in THF (50 mL) was added dropwise a mixture of 1,3-(4-hydroxyphenyl)benzene 1 (0.78 g, 3 mmol) and diisopropylethylamine (0.93 g, 7.2 mmol) in THF (25 mL) during 1 h. The reaction mixture was stirred for another 4 h. After removal of diisopropylethylamine hydrochloride salt through filtration, the filtrate was concentrated and chromatographed on a silica gel column (100-200) with a mixture of petroleum ether and CHCl3 (5:1) as the mobile phase to give pure 3 (1.32 g, 79%) as a white solid: mp 211-212 °C; 1H NMR (300 MHz, CDCl3) δ 7.80 (s, 1H), 7.72 (d, J = 8.7 Hz, 4H), 7.65 – 7.51 (m, 3H), 7.28 (d, J = 8.7 Hz, 4H); 13C NMR (75 MHz, CDCl3) δ 173.1, 171.1, 150.5, 140.6, 139.8, 129.4, 128.7, 126.4, 126.1, 121.3; IR (KBr) ν 1540, 1511, 1430, 1299, 1195, 1011 cm-1; EI-MS m/z 556 (78), 558 (100), 560 (50). Anal. Calcd for C24H12Cl4N6O2: C, 51.64; H, 2.17; N, 15.06. Found: C, 51.48; H, 2.22; N, 14.94.
Typical Procedure for the Synthesis of Oxacalix[2]m-terphenyl[2]triazine 4. A mixture of 1 (0.26 g, 1 mmol), 3 (0.56 g, 1 mmol) and DIPEA (0.31 g, 2.4 mmol) in CHCl3 (300 mL) was stirred at room temperature for 8 h. The mixture was then filtered and solvent was removed using a rotary evaporator. The residue was chromatographed on a silica gel column (100-200) with petroleum ether and chloroform (1:3) as the mobile phase to give pure 4 (0.48 g, 65%) as a white solid: mp 255-256 °C; 1H NMR (300 MHz, CDCl3) δ 7.53-7.51 (m, 2H), 7.41 (d, J = 8.7 Hz, 8H), 7.38 – 7.27 (m, 6H), 7.06 (d, J = 8.7 Hz, 8H); 13C NMR (75 MHz, CDCl3) δ 174.2, 172.1, 150.8, 140.2, 138.8, 129.2, 127.6, 126.0. 124.5, 121.4; IR (KBr) ν 1550, 1446, 1365, 1195 cm-1; MS [MALDI-TOF] m/z 747.3 [M + H+] (100), 749.3 (65), 751.3 (1). Anal. Calcd for C42H24Cl2N6O4.H2O: C, 65.89; H, 3.42; N, 10.98. Found: C, 65.90; H, 3.41; N, 10.59. A single crystal suitable for X-ray diffraction analysis was obtained from recrystallization from a mixture of toluene and chlorofom (1:3). Crystalographic data: C91H56Cl4N12O8 M = 1587.28, orthorhombic, Pbca, a = 7.8703(3) Å, b = 29.1424(11) Å, c = 32.8759(11) Å, α = 90.00°, β = 90.00°, γ = 90.00°, V = 7540.4(5) Å3 , Z = 4, μ(Mo-Kα) = 0.71073 mm-1, T= 173(2) K, The structure was solved and refined by SHELXL-97 in the WinGX package. Final residuals (503 parameters) R1 = 0.0866 for 6175 reflections with I > 2σ (I), and R1= 0.0975, wR2 = 0.2093. GoF = 1.155 for all 6894 data. CCDC 840018.
General Procedure for the Synthesis of Functionalized Oxacalix[2]m-terphenyl[2]triazines 6a and 6b. To a solution of 4 (0.15 g, 0.2 mmol) in THF (20 mL) at room temperature was added K2CO3 (finely ground) (0.11g, 0.8 mmol) and amine 5a or 5b (0.8 mmol). The resulting mixture was kept stirring for 8 h. The mixture was then filtered and solvent was removed using a rotary evaporator. The residue was chromatographed on a silica gel column (100-200) with a mixture of MeOH and acetone as the mobile phase to give pure product 6a or 6b.
6a (0. 14 g, 81%): a white solid; mp 222-223 °C; 1H NMR (300 MHz, DMSO) δ 7.85 (s, 2H), 7.68 (d, J = 8.5 Hz, 8H), 7.46 (d, J = 7.6 Hz, 4H), 7.37 – 7.30 (m, 2H), 7.26 (d, J = 8.4 Hz, 8H), 4.88 (t, J = 4.9 Hz, 4H), 3.71 (dd, J = 19.4, 4.8 Hz, 16H); 13C NMR (75 MHz, DMSO) δ 170.7, 167.6, 151.2, 139.4, 136.3, 129.4, 127.0, 125.2, 123.5, 121.7, 58.3, 50.5; IR (KBr) ν 3384, 1596, 1523, 1378, 1211, 1054 cm-1; MS [MALDI-TOF] m/z 885.5 [M + H+] (100), 886.5 (52), 887.5 (3). Anal. Calcd for C50H44N8O8.CH2Cl2: C, 63.16; H, 4.78; N, 11.55. Found: C, 63.16; H, 4.78; N, 11.45.
6b (0.165 g, 77%): a white solid; mp 197-198 °C; 1H NMR (300 MHz, CDCl3) δ 8.58 (d, J = 4.4 Hz, 4H), 7.68 (t, J = 8.1 Hz, 4H), 7.53 (s, 2H), 7.36 (d, J = 8.4 Hz, 12H), 7.32 – 7.25 (m, 6H), 7.24 – 7.16 (m, 4H), 7.04 (d, J = 8.5 Hz, 8H), 5.18 (s, 8H); 13C NMR (75 MHz, CDCl3) δ 172.0, 169.3, 156.7, 151.5, 149.5, 140.4, 137.9, 136.6, 129.0, 127.1, 125.6, 124.5, 122.3, 122.0, 121.9, 51.75; IR (KBr) ν 1592, 1517, 1381, 1212, 609 cm-1; MS [MALDI-TOF] m/z 1073.6 [M + H+] (100), 1074.6 (85), 1075.6 (25), 1076.6 (2), 1095.6 [M + Na+]. Anal. Calcd for C66H48N12O4: C, 73.87; H, 4.51; N, 15.66. Found: C, 73.70; H, 4.42; N, 15.60.
ACKNOWLEDGEMNT
We are grateful to the National Natural Science Foundation of China (20972161) and Ministry of Science and Technology, China (2011CB932501) for financial support. MMN thanks the Higher Education Commission of Pakistan for providing the Ph.D. scholarship.
References
1. For reviews: (a) M.-X. Wang, Chem. Commun., 2008, 4541; CrossRef (b) B. König and M. H. Fonseca, Eur. J. Inorg. Chem., 2000, 2303; (c) H. Tsue, K. Ishibashi, and R. Tamura, Top. Heterocycl. Chem., 2008, 17, 73; CrossRef (d) W. Maes and W. Dehaen, Chem. Soc. Rev., 2008, 37, 2393; CrossRef (e) N. Morohashi, F. Narumi, N. Iki, T. Hattori, and S. Miyano, Chem. Rev., 2006, 106, 5291; CrossRef (f) M.-X. Wang, Acc. Chem. Res., 2011, DOI: 10.1021/ar200108c. CrossRef
2. For a monograph: C. D. Gutsche, Calixarenes: An Introduction, The Royal Society of Chemistry, 2008.
3. (a) M.-X. Wang and H.-B. Yang, J. Am. Chem. Soc., 2004, 126, 15412; CrossRef (b) H.-Y. Gong, X.-H. Zhang, D.-X. Wang, H.-W. Ma, Q.-Y. Zheng, and M.-X. Wang, Chem. Eur. J., 2006, 12, 9262; CrossRef (c) Q.-Q. Wang, D.-X. Wang, Q.-Y. Zheng, and M.-X. Wang, Org. Lett., 2007, 9, 2847. CrossRef
4. (a) D.-X. Wang, Q.-Y. Zheng, Q.-Q. Wang, and M.-X. Wang, Angew. Chem. Int. Ed., 2008, 47, 7485; CrossRef (b) D.-X. Wang, Q.-Q. Wang, Y. Han, Y. Wang, Z.-T. Huang, and M.-X. Wang, Chem. Eur. J., 2010, 16, 13053. CrossRef
5. (a) H.-Y. Gong, Q.-Y. Zheng, X.-H. Zhang, D.-X. Wang, and M.-X. Wang, Org. Lett., 2006, 8, 4895; CrossRef (b) H.-B. Yang, D.-X. Wang, Q.-Q. Wang, and M.-X. Wang, J. Org. Chem., 2007, 72, 3757; CrossRef (c) E.-X. Zhang, D.-X. Wang, Q.-Y. Zheng, and M.-X. Wang, Org. Lett., 2008, 10, 2565; CrossRef (d) H.-Y. Gong, D.-X. Wang, Q.-Y. Zheng, and M.-X. Wang, Tetrahedron, 2009, 65, 87; CrossRef (e) M.-L. Ma, X.-Y. Li, and K. Wen, J. Am. Chem. Soc., 2009, 131, 8338; CrossRef (f) L.-X. Wang, L. Zhao, D.-X. Wang, and M.-X. Wang, J. Solid State Chem., 2010, 183, 3010; CrossRef (g) C.-Y. Gao, L. Zhao, and M.-X. Wang, J. Am. Chem. Soc., 2011, 133, 8448. CrossRef
6. (a) M.-X. Wang, X.-H. Zhang, and Q.-Y. Zheng, Angew. Chem. Int. Ed., 2004, 43, 838; CrossRef (b) S.-Q. Liu, D.-X. Wang, Q.-Y. Zheng, and M.-X. Wang, Chem. Commun., 2007, 3856; CrossRef (c) E.-X. Zhang, D.-X. Wang, Q.-Y. Zheng, and M.-X. Wang, Org. Lett., 2008, 10, 2565; CrossRef (d) M. M. Naseer, D.-X. Wang, L. Zhao, Z.-T. Huang, and M.-X. Wang, J. Org. Chem., 2011, 76, 1804. CrossRef
7. (a) H.-Y. Gong, D.-X. Wang, J.-F. Xiang, Q.-Y. Zheng, and M.-X. Wang, Chem. Eur. J., 2007, 13, 7791; CrossRef (b) Q.-Q. Wang, D.-X. Wang, H.-B. Yang, Z.-T. Huang, and M.-X. Wang, Chem. Eur. J., 2010, 16, 7265. CrossRef
8. For added examples: (a) W. Van Rossom, W. Maes, L. Kishore, M. Ovaere, L. Van Meervelt, and W. Dehaen, Org. Lett., 2008, 10, 585; CrossRef (b) L.-X. Wang, D.-X. Wang, Z.-T. Huang, and M.-X. Wang, J. Org. Chem., 2010, 75, 741; CrossRef (c) J.-C. Wu, D.-X. Wang, Z.-T. Huang, and M.-X. Wang, Tetrahedron Lett., 2009, 50, 7209; CrossRef (d) B. Yao, D.-X. Wang, and M.-X. Wang, J. Org. Chem., 2009, 74, 5361; CrossRef (e) B. Yao, D.-X. Wang, and M.-X. Wang, Chem. Commun., 2009, 2899; CrossRef (f) J.-C. Wu, D.-X. Wang, Z.-T. Huang, and M.-X. Wang, J. Org. Chem., 2010, 75, 8604; CrossRef (g) Y. Chen, D.-X. Wang, Z.-T. Huang, and M.-X. Wang, J. Org. Chem., 2010, 75, 3786; CrossRef (h) Y. Chen, D.-X. Wang, Z.-T. Huang, and M.-X. Wang, Chem. Commun., 2011, 47, 8112; CrossRef (i) W. Van Rossom, K. Robeyns, M. Ovaere, L. Van Meervelt, W. Dehaen, and W. Maes, Org. Lett., 2011, 13, 126; CrossRef (j) Y. Zhu, J. Yuan, Y. Li, M. Gao, L. Cao, J. Ding, and A.-X. Wu, Synlett, 2011, 52; CrossRef (k) H, Tsue, K. Ono, S. Tokita, K. Ishibashi, K. Matsui, H. Takahashi, K. Miyata, D. Takahashi, and R. Tamura, Org. Lett., 2011, 13, 490; CrossRef (l) J. L. Katz and B. A. Tschaen, Org. Lett., 2010, 12, 4300; CrossRef (m) C. Capici, G. Gattuso, A. Notti, M. F. Parisi, G. Bruno, F. Nicolo, and S. Pappalardo, Tetrahedron Lett., 2011, 52, 1351; CrossRef (n) W. Van Rossom, L. Kishore, K. Robeynes, L. Van Meervelt, W. Dehaen, and W. Maes, Eur. J. Org. Chem., 2010, 4122; CrossRef (o) S. Akagi, Y. Yasukawa, K. Kobayashi, and H. Konishi, Tetrahedron, 2009, 65, 9983; CrossRef (p) K. V. Lawson, A. C. Barton, and J. D. Spence, Org. Lett., 2009, 11, 895; CrossRef (q) M. Li, M.-L. Ma, X.-Y. Li, and K. Wen, Tetrahedron, 2009, 65, 4639; CrossRef (r) C.-F. Chen, Chem. Commun., 2011, 47, 1674 and references cited therein. CrossRef
9. J. L. Katz, B. J. Geller, and P. D. Foster, Chem. Commun., 2007, 1026. CrossRef
10. D. Shen, S. Diele, G. Pelzl, I. Wirth, and C. Tschierske, J. Mater. Chem., 1999, 9, 661. CrossRef