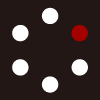
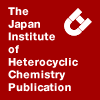
HETEROCYCLES
An International Journal for Reviews and Communications in Heterocyclic ChemistryWeb Edition ISSN: 1881-0942
Published online by The Japan Institute of Heterocyclic Chemistry
e-Journal
Full Text HTML
Received, 21st March, 2011, Accepted, 13th May, 2011, Published online, 30th May, 2011.
DOI: 10.3987/REV-11-707
■ Imidazolidin-4-ones: Their Syntheses and Applications
Timothy R. Blackmore* and Philip E. Thompson
Institute of Pharmaceutical Sciences, Monash University, 381 Royal Parade, Parkville, VIC 3052, Australia
Abstract
This review focuses on the synthesis and use of the imidazolidin-4-one ring in synthetic and medicinal chemistry studies. It has remained remarkably under-utilized as a motif in drug discovery despite its obvious similarity to the widely encountered lactam pyrrolidinone, its isomer imidazolin-2-one and its oxidation state variants, the hydantoins (imidazolidendiones), imidazolidines, and imidazoles, as well as fused bicyclic ring systems. The synthesis of imidazolidin-4-one is reported in only 3 journal articles and a search for the explicit use of it in synthesis resulted in just 5 patents, and no academic journals. The specific sub-structure motif is reported in 296 journal articles and 65 patents. The different methods reported for creating substituted analogues will be discussed, as well as the application of the moiety in medicinal chemistry projects and alternative uses of these analogues as organic catalysts and prodrugs.Early Reports:
The history of imidazolidin-4-one starts with investigations into the synthesis of diphenylhydantoin (phenytoin, Dilantin) described by Heinrich Biltz in 1908,1 and later found to have antiseizure effects.2 In the first reported synthesis of an imidazolidin-4-one, (Scheme 1), the phenytoin analogue, 5,5-diphenylthiohydantoin (1), was shown to react with sodium in amyl alcohol to produce 5,5-diphenylimidazolidin-4-one (2).3,4 Carrington et al. later reported that the same reduction of the thiocarbonyl intermediate (1) can also be achieved using Raney nickel.5 Biltz’s findings were later reinvestigated by Edward et al., while the key structures were in agreeance, there was a difference between the structures of some derivatives.6 Whalley et al. found that the desulfurization step could also yield a variety of stable intermediates or by-products including 5,5-diphenyl-2-hydroxy-4-imidazolidone (3) and 4,4-diphenyl-5-oxo-2-imidazoline (4).7 Such additional complexity may have limited the application of this route to imidazolidin-4-ones.
Synthesis of Imidazolidin-4-ones
Condensation of carbonyl compounds and aminoacetamide equivalents
One of the principle synthetic routes to imidazolidin-4-one derivatives incorporates a reaction between an aldehyde or ketone and an aminoacetamide. The unsubstituted imidazolidin-4-one itself can be produced this way, from simple starting materials (Scheme 2).8 Treatment of 2-(benzylamino)-acetamide (5) with formaldehyde gave 1-benzyl-3-(hydroxymethyl)imidazolidin-4-one (6). The hydroxymethyl group can be removed by careful distillation at low pressure, giving a 1-benzylimidazolidin-4-one (7). The benzyl group can then be hydrogenolysed to give the unsubstituted imidazolidin-4-one (8). This represents the simplest reported access to the title compound.
A study of imidazolidin-4-one cyclisation by Pascal et al. found that when formaldehyde is used to cyclize 2-(methylamino)propanamide (9) there are two products formed, the desired 1,5-dimethylimidazolidin-4-one (10) and the by-product 3-(hydroxymethyl)-1,5-dimethyl- imidazolidin-4-one (11) that exist in equilibrium (Scheme 3).9,10 While the hydroxy methyl may be removable in some cases, it can represent a significant obstacle to the usefulness of this approach.
Analogously, Harmon et al. had previously investigated the synthesis of 3-hydroxyimidazolidin-4-ones by condensing α-amino hydroxamic acids with aldehydes.11 For example, glycine hydroxamic acid (12) and formaldehyde combine to form 3-hydroxyimidazolidin-4-one (13) (Scheme 4), which was reported to be a ligand for the strychnine-insensitive glycine binding site of the NMDA receptor.12
The general reaction actually dates back to 1951 where Davis et al. reported the synthesis of 2,2-dimethylimidazolidin-4-one (Scheme 5). Aminoacetamide (14) and acetone were combined to form the Schiff base (15) which exists in equilibrium with the corresponding imino-oxazolidine (16). Rearrangement occurs to yield the more stable imidazolidin-4-one product (17). This product is still labile in basic or hot acidic solution, and is hydrolyzed back to aminoacetamide (14).13
Spiroimidazolidin-4-ones can be prepared by utilizing cyclic ketones (Scheme 6). Condensing cyclohexanone (18) with aminoacetonitrile (19) using sodium methoxide as a catalyst gives 1,4-diazaspiro[4.5]decan-2-one (20).13 This compound is more stable than dimethylimidazolidin-4-one (17). These examples suggest that using substituted ketones give cleaner or more stable products than using formaldehyde. A separate publication concluded that some of the reported compounds, specifically the 2-hydroxyimidazolidin-4-ones, actually existed as open chain tautomers.14
Khalaj et al. reported where a variety of 2-aminoacetamide analogues will cyclize with carbonyl compounds to imdazolidin-4-ones, but only by refluxing in methanol with a p-toluenesulfonic acid catalyst.15 Another report indicated that H-Y zeolite is also a useful catalyst, which has the advantage of being heterogeneous and can therefore be easily removed from the reaction by filtration.16 These reports show the scope of this reaction, proceeding with both aryl and alkyl derivatives on the acetamide and carbonyl reagents. A series of such compounds were patented for their anti-inflammatory and analgesic effects.17
Published methods exist for synthesizing a wide variety of diversely substituted imidazolidin-4-ones from a resin bound aminoacetamide. This presents an ideal opportunity for parallel synthesis of large libraries with significant diversity and this has been successfully demonstrated.
Rinnová et al. developed a solid phase synthesis of 1,2,5-trisubstituted imidazolidin-4-ones (27), that was used to synthesize an extensive series of analogues. Scheme 8 begins with reductive amination of a resin bound aminoacetamide (24), with benzaldehyde to give (25), which can then be cyclised with another aldehyde (26).
The resin cleavage step requires the use of hydrofluoric acid and produces diastereomeric products. This methodology was used to create a library of compound mixtures.18 A similar report using a modified synthetic scheme reported making a library of 180 different spiroimidazolidin-4-ones.19,20 An alternate method was published by Qin et al. including a solid phase synthesis of 1,2,5-trisubstituted imidazolidin-4-ones, that uses a photo cleavable linker, avoiding the hazards of using hydrofluoric acid.21
Cyclization by addition of Nitrogen
A less well documented synthetic approach exists where ammonia is inserted to cyclize a carbonyl containing compound. The Schiff base of an amino acid ester and an aldehyde can be cyclized to give 2,5 substituted imidazolidin-4-ones. The precursor and imidizolidin-4-ones are achieved in high yields, using mild conditions (Scheme 9).22 Amino acids esters can react with benzylidenemethylamine (31) to give tri-substituted imidazolidin-4-ones (Scheme 10).23 This is somewhat analogous to an intermolecular version of the Schiff base cyclisation above. Two molecules of acetone can be combined to yield a 2,2,5,5-tetramethylimidazolidin-4-one, through a reaction with sodium cyanide and ammonium chloride (Scheme 11).24 It has been supposed that this method would allow for large scale synthesis of relatively simple imidazolidin-4-ones.25
In another variation, treatment of α-haloacetamidobenzophenones (36) with hexamine yields 1,1’-methylenebis(3-(4-halophenyl)imidazolidin-4-ones) (37) which can be acid hydrolyzed to give corresponding imidazolidin-4-ones (38).26
Imidazolidin-4-one by ring expansion and contraction
Other general synthetic routes to imidazolidin-4-one and analogues rely on ring expansion reactions. Imidazolidin-4-one structures have been reported by ring expansion of precursor β-lactams. In another explicit synthesis of imidazolidin-4-one itself, the azetidin-3-one (39) is converted to an oxime and then mesylated to give 41. Beckmann rearrangement gives the carbamate which can be deprotected to yield the desired compound (43).27
Bird et al. described the conversion of β-lactam (44) to imidazolidin-4-one (45) (Scheme 14). The benzylacetamide function was shown to play some role in the transformation. This was shown by replacing it with p-methylbenzenesulfonamide and observing that it does not react in the same manner.28 This suggested that the reactions usefulness could be limited to only a smaller range of compounds.
Oxaziridine (46) reacts with substituted N-arylketenimine (47) and to produce a complex imidazolidin-4-one (48) (Scheme 15). The nature of this product was confirmed by several subsequent reactions. Acidic hydrolysis yielded anilide and benzaldehyde. Treatment with lithium aluminium hydride with an acidic workup gave an acyclic diamine and benzaldehyde, yet with a basic workup gave a 1,3-diazolidine.29 The reaction in Scheme 16 produced imidazolidin-4-ones (51) from nitrones (49) and isocyanides (50) in a conceptually similar manner.30
A number of examples where aziridine precursors have been converted to imidazolidin-4-ones exist in the literature. In Scheme 17 2-cyanoaziridine (52) interconverts to compound (53) which reacts with phenylisocyanate to form 4-imidazolidinone (54).31 A similar reaction is shown in Scheme 18, substituted 2-(alkoxycarbonyl)aziridines (55) ring open to give azomethine ylides (56) which can react with vinyl isocyanates and give rise to imidazolidin-4-ones (57). This shows that a variety of functional groups are tolerated in this transformation.32
Finally in Scheme 19 3-aryl-1-methylaziridine-2-carboxamides (59) convert to intermediates (58) and react with 1-aryl-2-bromoethenes to give functionalized imidazolidin-4-ones (60).33
A remarkably fast ring expansion occurs when adding LDA to a solution of 1-benzhydryl-2,4-dimethyl-1,2-diazetidin-3-one (61), where the N-methyl carbon is incorporated into the imidazolidin-4-one ring of 62 (Scheme 20).34 The suggested mechanism was supported by deuterium incorporation experiments.
In an attempt to synthesize a range of substituted β-lactam ring systems Shevtsov and coworkers discovered Scheme 21 where 1,2-dialkyldiaziridines (64) react with arylketenes (63) to give imidazolidin-4-ones (65).35
Imidazolin-4-ones have also been obtained by ring contraction. Photochemical irradiation of 3,6,6-trimethyl-5,6-dihydropyrazin-2(1H)-one (66) (450W mercury lamp) in aqueous solution gave 5-methylimidazolidin-4-one (67) (Scheme 22). The by-product of this reaction is acetone and a corresponding mechanism has been suggested, however the practical scope of this reaction is yet to be reported.36
Another interesting ring contraction (Scheme 23) to highly derivatized imidazolidin-4-ones (69) arises from the desulfurization of hexahydrotriazines (68), however this approach also yields hydantoins. A complex six step mechanism is proposed for this reaction.37
Other routes to imidazolin-4-ones
Several other routes have been taken to imidazolidin-4-one derivatives and are summarized here. First, 4-nitro-1-phenylazoles (70) treated with reducing aluminium give an intermediate oxime (71), and that converts to an imidazolidin-4-one (72) in fair yields. The versatility of this pathway has yet to be significantly investigated (Scheme 24).38
The Weinreb amide (73) in Scheme 25 reacts with potassium hexamethyldisilazide and cyclopentyl bromide to give a substituted imidazolidin-4-one (74). However the cyclisation reaction is dependent on the exact nature of the substituents, and can yield alternate 5-membered rings, therefore may not be useful in other synthetic schemes.39
As shown in Scheme 26, 2-vinylimidazolidin-4-ones (76) can be prepared from α-amino allenylamides (75). Microwave heating decreased the yield of imidazolidin-4-ones in favor of a 6-membered ring product.40 Use of a gold catalyst can greatly increase the yield of imidazolidin-4-ones in this reaction.41
The reaction in Scheme 27 of diethylzinc with a α-aldiminoester (77) yields a substituted imidazolidin-4-one (78) albeit as a side product of (79). The reaction is enantioselective but the imidazolidin-4-one was the minor product in all but one reported case.42 Perhaps this would be amenable to optimization.
Another method of combining an aminoacetamide and carbonyl containing compound is a three-component aza-Michael addition reaction (Scheme 28). The example below uses (S)-2-amino-N-alkyl-3-methylbutanamide (80), 4-nitrobenzaldehyde (81) and but-3-en-2-one (82) producing imidazolidin-4-ones (83) in a highly stereoselective manner (up to 50 : 1).43 The usefulness of chiral imidazolidin-4-ones as organic catalysts will be discussed further.
Imidazolidin-4-ones in synthesis
There are rather fewer reported reactions that use imidazolidin-4-ones as reactants reported in the literature. This may reflect the simplicity of making these compounds with the desired functionalities already in place or the difficulty of modifying them post-cyclization.
The facile nature of imidazolidin-4-one ring cyclisation and opening has been exploited in the synthesis of modified amino acids, via alkylation or substitution at an existing chiral center with stereocontrol.44
Glycinamide hydrochloride is converted to racemic 2-tert-butylimidazolidin-4-one by treatment with pivaldehyde followed by TFA. This racemic mix can be resolved by chiral HPLC or by two rounds of crystallization, the first with camphor sulfonic acid and then with N-acetyl-(R)-valine to give an enantiomeric ratio of greater than 99.5:0.5. The enantiopure imidazolidin-4-one (86) is protected with Boc and methylated to give (88). Activation with lithum diisopropylamide allows for substitution with an electrophile in a highly stereoselective manner. The monosubstituted imidazolidin-4-one (90) can then be deprotected, hydrolyzed without racemization. The resultant amino acid methyl ester is treated with benzylchloroformate to protect the amine, and for ease of purification. Alternatively a second round of activation, this time with N-butyl lithium and another stereoselective reaction with an electrophile gives di-substituted imidazolidin-4-one (92). Following deprotection and hydrolysis an α,α-disubstituted amino acid methyl ester is revealed (Scheme 29).45,46
In a truncated Scheme 30 (S)-1-benzoyl-2-(tert-butyl)-3-methyl-4-imidazolidinone (95) is a key precursor in the stereospecific synthesis of the antibiotic (+)-obafluorin (96).47 A related method has also been used in a pilot plant scale synthesis of a cell adhesion inhibitor BIRT-377 (97).48
Several other works that include imidazolidin-4-one in some way have been published, some examples of which will be examined here.
Scheme 31 shows substituted imidazolidin-4-ones (98) can undergo 4+4 cycloaddition reactions with tetrachloro-o-benzoquinone (99) to give complex tricyclic systems (100).49
Whilst looking for novel nitrogen containing analogues of imidazolidin-4-ones, Blass et al. found that imidazolidin-4-ones (101) undergo nitrosation (102) at the amine function as expected. Reduction to the corresponding amine was found to be more challenging, proceeding only in the presence of zinc dust and ammonium chloride, yielding 1-amino imidazolidin-4-one (103) (Scheme 32).50
An investigation into the optical properties and geometry of imidazolidin-4-ones derived from α-amino acids concluded that circular dichroism spectrometry is a useful method for analyzing such compounds.51 A series of NMR experiments were also undertaken in an effort to better characterize these substituted ring systems.52,53 Hydrolysis of the imidazolidin-4-one ring can be achieved by refluxing in 6 M hydrochloric acid.54 Some 2,2,5,5-substituted imidazolidin-4-ones can form stable nitroxide radicals, and can be used to stabilize synthetic polymers against light.55
There are a number of other medicinal chemistry projects that used imidazolidin-4-one in some way that will be discussed later.
As Organic Catalysts
Imidazolidin-4-ones make up a family of very useful organic catalysts generally referred to as iminium catalysts. They have a well understood activation mode that can be utilized in a number of different stereoselective reactions.56 MacMillan et al. first reported highly enantioselective organocatalysis of the Diels-Alder reaction Scheme 33. Finding that (S)-5-benzyl-2,2,3-trimethylimidazolidin-4-one (104) is an efficient asymmetric catalyst that can be used in place of older Lewis acid type catalysts.56
This catalytic strategy was also applied to a 1,3-dipolar cycloaddition reaction in Scheme 34 between a α,β-unsaturated aldehyde (106) and a nitrone (105) giving 108 with a high ee. This reaction was not viable with traditional metal catalysis, possibly due to metal chelation by the nitrone group, which is not an issue when using this organic catalyst (107).57
In Scheme 35, singly occupied molecular orbital activation catalysis allows for asymmetric functionalization alpha to a carbonyl group. This unique organocatalytic mechanism utilizes the same class of imidazolidin-4-one organic catalysts (109) as iminium catalysis but involves a radical cation.58,59
A solid phase equivalent of the iminium catalyst, used in the enantioselective Diels-Alder reaction, has been synthesized attached to a PEG polymer matrix. This allows the catalyst to function as it does in solution, and for easier catalyst recycling.60 It has also been shown that different solid supports (e.g. amide resin, silica) can be used to tune the activity of the catalyst.61-63
Imidazolidin-4-ones in Medicinal Chemistry
The prevalence of imidazolidin-4-one in medicinal chemistry journals and patents has risen significantly in recent years. This could be due to a number of reasons, including the need for pharmaceutical companies to constantly be moving into new chemical space, rising interest in structurally related compounds such as Rolipram (110) or simply that a small rise in interest promoted awareness and research in this motif.
The imidazolidin-4-one scaffold has appeared in a number of recent patents and medicinal chemistry journals for a wide variety of disease states. There have been patents filed for their use as CCR1 antagonists with possible anti-inflammatory effects (111),64 and as sodium channel inhibitors which are typically used to treat cardiac arrythmia (112).65 A series of spiroimidazolidin-4-ones (113) were patented by Pfizer as a treatment for diabetes related conditions.66 A short series of compounds utilizing an imidazolidin-4-one scaffold (114) were synthesized and tested for inhibitory activity at human leukocyte elastase for a possible treatment of emphysema.67 Simple N,N-dihaloimidazolidin-4-ones (115) have been patented for being biocidal at low concentrations.25
There is a similar variety of imidazolidin-4-one containing compounds that have been reported as BBB penetrating agents with various activities. One patent claimed a series of analogs as CNS penetrating agents (116).68 Another series has been patented as inhibitors of β-secretase for treatment for Alzheimer’s disease (117).69 Analogues of Spiperone (118) were originally patented in 1975 and have become useful as radiolabels for imaging dopamine receptor subtypes.70,71 In work related to the synthesis of an unsubstituted imidazolidin-4-one ring by Pfieffer et al. a patent has been filed for a series of nootropic compounds (119).72 A related paper by Pinza et al., utilizes imidazolidin-4-ones as an intermediate to get to a related series of bicyclic compounds that could also be used as cognition enhancers.73 This vast breadth of applications in medicinal chemistry highlights both the novelty and versatility of this motif.
Imidazolidin-4-ones can be labile to hydrolysis under physiological conditions; this has lead to several investigations into prodrugs containing this motif. A prime example is Hetacillin (Figure 2, 120), a prodrug of ampicillin which contains an imidazolidin-4-one ring that is cleaved in the body.74
Ethanol is metabolized to acetaldehyde, it was hypothesized that this could react with a metabolite of lidnocaine to form a stable imidazolidin-4-one species.75 The equivalent condensation with prilocaine (121) and formaldehyde to form an imidazolidin-4-one prodrug (122) was investigated along with the kinetics of its degradation (Scheme 36).76
An analogous study (Scheme 37) was conducted on imidazolidin-4-one prodrugs (124) of primaquine derivatives (123). Further studies found that these prodrugs were active anti-malarial agents in their own right.77,78 It was also found that formation of such prodrugs can occur in an enantioselective manner.79
To improve the metabolic stability of a peptide the amino terminus can be condensed with an aldehyde such as acetone and form an imidazolidin-4-one. This peptide motif has been reported to occur naturally. The open side chain of cyclopeptide alkaloid nummularine-B (125) was converted to an imidazolidin-4-one (126) by treatment with formaldehyde in ethanol (Scheme 38). This derivatization was used to confirm the existence of a natural equivalent peptide.80
In the presence of formaldehyde or acetaldehyde the N-terminus of glycopeptide antibiotic vancomycin will spontaneously convert to an imidazolidin-4-one. This modification is reversible but while in the cyclized state the drugs potency is significantly reduced.81 Similarly enkephalin peptides can resist metabolism by adding an imidazolidin-4-one ring into the peptide backbone. The peptide will be spontaneously hydrolyzed back to its native state in physiological conditions.82 Cyclizing the N-terminus with acetone increases the half life of leu-enkephalin from 6 minutes to 23.5 hours.82 The activity and stability of leu-enkephalin with imidazolidin-4-one rings installed at different positions have been studied.83
CONCLUSION
In this review, we have identified the surprising number of different synthetic approaches that can be taken to the synthesis of imidazolidin-4-one derivatives, and the variety of applications in which they can be exploited as reagents, catalysts, bioactive molecules and prodrugs.
While the imidazolin-4-one ring has in many respects been neglected as a functional moiety in medicinal chemistry, it has been appearing more often in recent times particularly in the patent literature. This would seem to be logical as the ring system has multiple points of substitution and within its own structure multiple means by which it could participate in interactions with macromolecular targets. As such simply substituted imidazolin-4-ones can be envisaged as excellent components of fragment-based screening libraries likely to possess high ligand efficiency as well as chemical novelty. The variety of synthetic approaches should see numerous new compounds and interesting applications of this class of heterocycle developed in the future.
References
1. H. Biltz, Berl. Dtsch. Chem. Gesamte., 1908, 41, 1379. CrossRef
2. T. J. Putnam and H. H. Merritt, Science, 1937, 85, 525. CrossRef
3. H. Biltz, Chem. Ber., 1909, 42, 1792. CrossRef
4. H. Biltz and K. Seydel, Liebigs Annalen., 1912, 391, 215. CrossRef
5. H. C. Carrington, C. H. Vasey, and W. S. Waring, J. Chem. Soc., 1953, 3105. CrossRef
6. J. T. Edward and I. Lantos, Can. J. Chem., 1967, 45, 1925. CrossRef
7. W. B. Whalley, E. L. Anderson, F. Dugan, J. W. Wilson, and G. E. Ullyot, J. Am. Chem. Soc., 1955, 77, 745. CrossRef
8. U. Pfeiffer, M. T. Riccaboni, R. Erba, and M. Pinza, Liebigs Annalen., 1988, 993. CrossRef
9. R. Pascal, M. Lasperas, J. Taillades, A. Commeyras, and A. Perez-Rubalcaba, Bull. Soc. Chim. Fr. II, 1984, 7, 329.
10. R. Pascal, J. Taillades, and A. Commeyras, Tetrahedron, 1978, 34, 2275. CrossRef
11. R. E. Harmon, V. L. Rizzo, and S. K. Gupta, J. Heterocycl. Chem., 1970, 7, 439. CrossRef
12. A. Cordi, J. M. Lacoste, V. Audinot, and M. Millan, Bioorg. Med. Chem. Lett., 1999, 9, 1409. CrossRef
13. A. C. Davis and A. L. Levy, J. Chem. Soc., 1951, 3479. CrossRef
14. E. Schipper and E. Chinery, J. Org. Chem., 1961, 26, 4480. CrossRef
15. A. Khalaj, R. D. Bazaz, and M. Shekarchi, Monatshe. Chem., 1997, 128, 395. CrossRef
16. M. A. Nooshabadi, K. Aghapoor, M. Bolourtchian, and M. M. Heravi, J. Chem. Res., Synop., 1999, 498. CrossRef
17. F. Kusuda, Y. Matsuo, and E. Seto, 1966, US3284447.
18. M. Rinnova, A. Vidal, A. Nefzi, and R. Houghten, J. Comb. Chem., 2002, 4, 209. CrossRef
19. L. Feliu, G. Subra, J. Martinez, and M. Amblard, J. Comb. Chem., 2003, 5, 356. CrossRef
20. P. Bedos, L. Feliu, J. Martinez, and M. Amblard, Tetrahedron Lett., 2003, 44, 4937. CrossRef
21. L.-Y. Qin, Tetrahedron Lett., 2009, 50, 419. CrossRef
22. P. G. Wiering and H. Steinberg, Recl. Trav. Chim. Pays-Bas, 1971, 90, 284. CrossRef
23. Y. G. Gololobov and L. I. Nesterova, Khim. Geterotsikl. Soedin., 1980, 706.
24. R. Anzai and K. Kikuchi, 2002, JP 2002265453.
25. S. D. Worley, T. Tsao, and D. E. Williams, 1991, WO 9110623.
26. M. Hannoun, M. Zinic, D. Kolbah, N. Blazevic, and F. Kajfez, J. Heterocycl. Chem., 1981, 18, 963. CrossRef
27. Y. Nitta, Heterocycles, 1986, 24, 25. CrossRef
28. C. W. Bird, Tetrahedron Lett., 1964, 609. CrossRef
29. N. Murai, M. Komatsu, Y. Ohshiro, and T. Agawa, J. Org. Chem., 1977, 42, 448. CrossRef
30. D. Moderhack, M. Lorke, and D. Schomburg, Liebigs Annalen., 1984, 1685. CrossRef
31. H. Benhaoua, F. Texier, and R. Carrie, Tetrahedron, 1986, 42, 2283. CrossRef
32. O. Mamoun and H. Benhaoua, Bull. Soc. Chim. Belg., 1994, 103, 753. CrossRef
33. J.-Y. Wang, Y. Hu, D.-X. Wang, J. Pan, Z.-T. Huang, and M.-X. Wang, Chem. Comm., 2009, 422. CrossRef
34. E. C. Taylor, R. J. Clemens, H. M. L. Davies, and N. F. Haley, J. Am. Chem. Soc., 1981, 103, 7659. CrossRef
35. A. V. Shevtsov, V. Y. Petukhova, Y. A. Strelenko, K. A. Lyssenko, I. V. Fedyanin, and N. N. Makhova, Mendeleev Commun., 2003, 13, 221. CrossRef
36. D. L. Kleyer and T. H. Koch, J. Org. Chem., 1982, 47, 3145. CrossRef
37. T. Sheradsky and N. Itzhak, J. Chem. Soc., Perkin. Trans. 1, 1989, 33. CrossRef
38. J. Suwinski and P. Wagner, Pol. J. Chem., 2000, 74, 1575.
39. C. L. Gibson, A. R. Kennedy, R. R. Morthala, J. A. Parkinson, and C. J. Suckling, Tetrahedron, 2008, 64, 7619. CrossRef
40. G. Broggini, S. Galli, M. Rigamonti, S. Sottocornola, and G. Zecchi, Tetrahedron Lett., 2009, 50, 1447. CrossRef
41. A. Manzo, Tetrahedron Lett., 2009, 50, 4696. CrossRef
42. S. Basra, M. W. Fennie, and M. C. Kozlowski, Org. Lett., 2006, 8, 2659. CrossRef
43. Z. Xu, T. Buechler, K. Wheeler, and H. Wang, Chem. Eur. J., 2010, 16, 2972. CrossRef
44. D. Seebach, D. D. Miller, S. Mueller, and T. Weber, Helv. Chim. Acta, 1985, 68, 949. CrossRef
45. M. Hoffmann, S. Blank, D. Seebach, E. Küsters, and E. Schmid, Chirality, 1998, 10, 217. CrossRef
46. D. Seebach and M. Hoffmann, Eur. J. Med. Chem., 1998, 1337. CrossRef
47. C. Lowe, Y. Pu, and J. C. Vederas, J. Org. Chem., 1992, 57, 10. CrossRef
48. N. K. Yee, L. J. Nummy, R. P. Frutos, J. J. Song, E. Napolitano, D. P. Byrne, P.-J. Jones, and V. Farina, Tetrahedron: Asymmetry, 2003, 14, 3495. CrossRef
49. W. Friedrichsen, W. D. Schroeer, and T. Debaerdemaeker, Liebigs Annalen., 1980, 1850. CrossRef
50. B. E. Blass, K. Coburn, N. Fairweather, A. Fluxe, S. Hodson, C. Jackson, J. Janusz, W. Lee, J. Ridgeway, R. White, and S. Wu, Tetrahedron Lett., 2006, 47, 7497. CrossRef
51. T. Polonski, Tetrahedron, 1985, 41, 611. CrossRef
52. T. Połoński, Org. Magn. Reson., 1984, 22, 176. CrossRef
53. C. Garcia-Martinez, H. Cervantes-Cuevas, and J. Escalante-Garcia, Chirality, 2003, 15, S74. CrossRef
54. D. Seebach, E. Juaristi, D. D. Miller, C. Schickli, and T. Weber, Helv. Chim. Acta, 1987, 70, 237. CrossRef
55. T. Toda, S. Morimura, E. Mori, H. Horiuchi, and K. Murayama, Bull. Chem. Soc. Jap., 1971, 44, 3445. CrossRef
56. K. A. Ahrendt, C. J. Borths, and D. W. C. Macmillan, J. Am. Chem. Soc., 2000, 122, 4243. CrossRef
57. W. S. Jen, J. J. M. Wiener, and D. W. C. Macmillan, J. Am. Chem. Soc., 2000, 122, 9874. CrossRef
58. T. D. Beeson, A. Mastracchio, J.-B. Hong, K. Ashton, and D. W. C. Macmillan, Science, 2007, 316, 582. CrossRef
59. H.-Y. Jang, J.-B. Hong, and D. W. C. Macmillan, J. Am. Chem. Soc., 2007, 129, 7004. CrossRef
60. M. Benaglia, G. Celentano, M. Cinquini, A. Puglisi, and F. Cozzi, Adv. Synth. Catal., 2002, 344, 149. CrossRef
61. S. A. Selkala, J. Tois, P. M. Pihko, and A. M. P. Koskinen, Adv. Synth. Catal., 2002, 344, 941. CrossRef
62. A. Puglisi, M. Benaglia, M. Cinquini, F. Cozzi, and G. Celentano, Eur. J. Org. Chem., 2004, 567. CrossRef
63. C. S. Pecinovsky, G. D. Nicodemus, and D. L. Gin, Chem. Mater., 2005, 17, 4889. CrossRef
64. P. Zhang, A. M. K. Pennell, L. Li, and E. J. Sullivan, 2008, US 7786157.
65. C. Cattaneo, R. Fariello, and R. Maj, 2009, EP2093218.
66. J. L. Belletire and R. Sarges, 1981, EP0028485B1.
67. L. Wei, Bioorg. Med. Chem., 2003, 11, 5149. CrossRef
68. B. Kenda, L. Turet, L. Quesnel, P. Michel, and A. Ates, 2008, WO 2008132142.
69. J. C. Barrow, K. E. Rittle, and P. L. Bondiskey, 2007, WO2007058862.
70. K. Sasajima, K. Ono, M. Nakao, I. Maruyama, M. Takayama, S. Katayama, J. Katsube, S. Inaba, and H. Yamamoto, 1975, JP 50005385A.
71. V. Bakthavachalam, N. Baindur, B. K. Madras, and J. L. Neumeyer, J. Med. Chem., 1991, 34, 3235. CrossRef
72. M. Pinza, U. Pfeiffer, C. Farina, and S. Banfi, 1987, EP0207681A2.
73. M. Pinza, C. Farina, A. Cerri, U. Pfeiffer, M. T. Riccaboni, S. Banfi, R. Biagetti, O. Pozzi, M. Magnani, and L. Dorigotti, J. Med. Chem., 1993, 36, 4214. CrossRef
74. G. A. Hardcastle, Jr., D. A. Johnson, C. A. Panetta, A. I. Scott, and S. A. Sutherland, J. Org. Chem., 1966, 31, 897. CrossRef
75. S. D. Nelson, G. D. Breck, and W. F. Trager, J. Med. Chem., 1973, 16, 1106. CrossRef
76. S. W. Larsen, M. Sidenius, M. Ankersen, and C. Larsen, Eur. J. Pharm. Sci., 2003, 20, 233. CrossRef
77. P. Gomes, M. J. Araújo, M. Rodrigues, N. Vale, Z. Azevedo, J. Iley, P. Chambel, J. Morais, and R. Moreira, Tetrahedron, 2004, 60, 5551. CrossRef
78. M. J. Araujo, J. Bom, R. Capela, C. Casimiro, P. Chambel, P. Gomes, J. Iley, F. Lopes, J. Morais, R. Moreira, O. E. De, R. V. Do, and N. Vale, J. Med. Chem., 2005, 48, 888. CrossRef
79. R. Ferraz, J. R. B. Gomes, O. E. De, R. Moreira, and P. Gomes, J. Org. Chem., 2007, 72, 4189. CrossRef
80. A. H. Shah, V. B. Pandey, G. Eckhardt, and R. Tschesche, Phytochemistry, 1985, 24, 2765. CrossRef
81. A. J. R. Heck, P. J. Bonnici, E. Breukink, D. Morris, and M. Wills, Chem. Eur. J., 2001, 7, 910. CrossRef
82. G. J. Rasmussen and H. Bundgaard, Int. J. Pharm., 1991, 76, 113. CrossRef
83. M. Rinnová, A. Nefzi, and R. A. Houghten, Bioorg. Med. Chem. Lett., 2002, 12, 3175. CrossRef