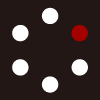
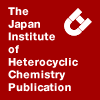
HETEROCYCLES
An International Journal for Reviews and Communications in Heterocyclic ChemistryWeb Edition ISSN: 1881-0942
Published online by The Japan Institute of Heterocyclic Chemistry
e-Journal
Full Text HTML
Received, 23rd June, 2011, Accepted, 1st August, 2011, Published online, 10th August, 2011.
DOI: 10.3987/COM-11-S(P)48
■ Enantioselective Cyclization Reactions of (Z)-N-Benzoyl-α-dehydro(1-naphthyl)alanine N’-Arylamides Initiated by Photoinduced Electron Transfer from Chiral Prolinol
Hiroyuki Yadai, Yuhki Sato, Tetsutaro Igarashi, and Tadamitsu Sakurai*
Material and Life Chemistry, Faculty of Engineering, Kanagawa University, 3-27-1 Rokkakubashi, Kanagawa-ku, Yokohama 221-8686, Japan
Abstract
An investigation was undertaken to explore substituent effects on the photoinduced electron transfer-initiated enantioselective cyclization of the title α-dehydronaphthylalanine N’-(2-hydroxyphenyl)amide [(Z)-1a], N’-(3-hydroxyphenyl)amide [(Z)-1b], N’-(4-hydroxyphenyl)amide [(Z)-1c], and N’-phenylamide [(Z)-1d] in 1,2-dichloroethane containing chiral N-methylprolinol or prolinol. 1H NMR spectral and HPLC chiral analyses of the target photoproducts, cis- and trans-4,5-dihydrooxazole isomers, confirmed that enantiomeric excess (ee) for these two isomers (estimated in the presence of the former prolinol) displayed a clear tendency to decrease in order of (Z)-1d > (Z)-1c > (Z)-1b > (Z)-1a. Hydrogen bonding and charge transfer interactions in radical ion pair and biradical intermediates were suggested to be key factors controlling ee, the value of which varied from 0 to 58%.INTRODUCTION
Sophisticated organic photochemistry has continued to contribute to the development of interesting asymmetric cyclization and cycloaddtion reactions, enabling the efficient synthesis of chiral and biologically active heterocyclic compounds, for the last two decades.1 Since photoinduced electron transfer (PET) reactions have already been found to give various heterocyclic compounds with high efficiencies,2 we embarked on a systematic study of PET-initiated reductive cyclization reactions of N-acyl-α-dehydroamino acid derivatives from both synthetic and mechanistic points of view. In the course of this systematic study we found that N-acyl-α-dehydroarylalaninamides and related arylalanine alkyl esters in their excited states readily undergo one-electron reduction in the presence of an aliphatic amine to eventually afford the corresponding 3,4-dihydroquinolinone and 4,5-dihydrooxazole derivatives with high selectivities, respectively.3,4 Extremely large photostability of these products that possess asymmetric carbons in the heterocyclic rings stimulated us to explore an unprecedented asymmetric cyclization of α-dehydroarylalaninamides and α-dehydroarylalanine alkyl esters, initiated by PET. As demonstrated in previous papers, our PET-initiated diastereoselective photocyclization proceeded efficiently to enable the construction of a chiral dihydroquinolinone or dihydrooxazole ring in a high diastereomeric excess (de), by introducing a bulky chiral auxiliary into the amide or the ester moiety of these derivatives.5,6
On the other hand, an attempt to develop the enantioselective photocyclization of N-benzoyl-α-dehydroarylalanine alkyl esters in the presence of chiral prolinol also proved successful, though enantiomeric excess (ee) for this asymmetric cyclization was not so high.6 A mechanistic analysis of the diastereoselective photocyclization of N-benzoyl-α-dehydroarylalanine alkyl esters revealed that asymmetry in this reaction is induced at the steps of the cycloaddition of a radical ion pair intermediate initially formed via PET as well as of the hydrogen shift in the subsequent biradical intermediate.6 In addition, hydrogen bonding and electrostatic interactions in these intermediates are proposed to be major factors controlling the observed asymmetric photoinduction. However, we found that π-face selective cyclization takes place to a negligible extent in any radical ion pair intermediates derived from the N-benzoyl-α-dehydroarylalanine alkyl ester/chiral prolinol systems.6 If we consider that this finding provides a good explanation for the less enantioselective photocyclization, we should improve the molecular design of the starting N-acyl-α-dehydroarylalanine derivative to elucidate the role of the chiral amine-derived radical cation in the cycloaddition of the above-mentioned radical ion pair intermediate and then to enhance enantioselectivity for the observed PET-initiated cyclization.
It was quite recently demonstrated that replacement of an alkoxycarbonyl group in the starting α-dehydronaphthylalanine alkyl ester by a bulky alkylaminocarbonyl group completely suppresses amine-catalyzed epimerization of alkylaminocarbonyl-substituted 4,5-dihydrooxazoles as photoproducts and, additionally, greatly enhances the π-face selectivity described above.7,8 Taking into account that phenolic hydroxy group may exert a large effect on hydrogen bonding and electrostatic interactions in the proposed intermediates, our attention in the present study was directed toward introducing a bulky hydroxyanilinocarbonyl functional group into the amide moiety of N-benzoyl-α-dehydronaphthylalaninamides. To elucidate the role of these interactions in a chiral amine radical cation/alaninamide radical anion pair and a biradical/chiral amine pair as key intermediates and also to devise an asymmetric photocyclization system proceeding in a high ee, substituent effects on the extent of asymmetric induction and the product composition in the PET-initiated photocyclization of α-dehydronaphthylalaninamide derivatives were investigated. For the purpose of investigating these substituent effects we adopted (Z)-N-benzoyl-α-dehydro(1-naphthyl)alanine N’-(hydroxy-substituted phenyl)amides [(Z)-1a–c] and (Z)-N-benzoyl-α-dehydro(1-naphthyl)alanine N’-phenylamide [(Z)-1d] as the starting naphthylalaninamides and (S)-pyrrolidine-2-methanol (S-PM), (R)-pyrrolidine-2-methanol (R-PM), and (S)-1-methylpyrrolidine-2-methanol (S-MPM) as chiral amines (Chart 1).
RESULTS AND DISCUSSION
The starting N-benzoyl-α-dehydronaphthylalanine N’-arylamides of the (Z)-configuration were prepared in higher than 60% yields by the ring-opening reactions of (Z)-4-(1-naphthylmethylene)-2-phenyl-5(4H)-oxazolone (5.0 mmol) with the corresponding arylamines (10 mmol) in refluxing tetrahydrofuran. To isolate the (Z)-1a-derived photoproducts and determine their structures, nitrogen-saturated 1,2-dichloroethane solutions of this α-dehydronaphthylalaninamide (4.0×10–3 mol dm–3, 10 mL×10) containing S-MPM (0.10 mol dm–3) were irradiated (at wavelengths longer than 280 nm from a 400 W high-pressure Hg lamp) in parallel using a merry-go-round-type irradiation equipment for 12 h at room temperature (conversion, 100%). The reaction mixture (obtained after S-MPM in the irradiated solutions was removed by treatment with hydrochloric acid) was subjected to preparative thin layer chromatography over silica gel. By this procedure we were able to isolate cis- and trans-4-(2-hydroxyanilinocarbonyl)-5-(1-naphthyl)-2-phenyl-4,5-dihydrooxazoles as their enantiomeric mixtures [cis-2a: (4R,5R)-2a + (4S,5S)-2a, 45% yield; trans-2a: (4S,5R)-2a + (4R,5S)-2a, 32% yield] having the vicinal coupling constants (J4,5) of 11.0 Hz and 6.5 Hz in DMSO-d6, respectively, along with a small amount of 3-(2-hydroxyanilinocarbonyl)-1-phenylbenzo[f]isoquinoline (3a, <1%), as shown in Scheme 1. The (E)-isomer of 1a was isolated independently from the reaction mixture, which was irradiated for
1 h under the same conditions, by similar workup. 1H NMR compositions of cis-2a, trans-2a, 3a, and (E)-1a were estimated prior to removing S-MPM and to isolating these dihydrooxazole isomers 2a. A normal-phase HPLC chiral analysis of cis-2a and trans-2a isolated showed that the former isomer gave two signals at retention times of 26.1 and 30.6 min and the latter isomer at 11.0 and 13.3 min, when the mixture of 2-propanol (5 vol%), chloroform (15 vol%), and n-hexane (80 vol%) was used as a mobile phase. The 2a-derived enantiomers exhibiting their HPLC signals at retention times of 26.1 and 11.0 min gave CD bands of positive sign at 225 nm, while CD bands of negative sign were observed for the other enantiomers showing their HPLC signals at 30.6 and 13.3 min. Our previous studies fully established that the sign of CD bands observed at 220–225 nm reflects absolute configuration of each enantiomer at 5-position on the dihydrooxazole ring6 and also (4S,5S)- and (4R,5R)-4-(N-substituted aminocarbonyl)-5-(1-naphthyl)-2-phenyl-4,5-dihydrooxazoles display circular CD bands of positive and negative signs at 225 nm, respectively.8 Thus, we were led to conclude that HPLC signals detected at 26.1 and 30.6 min are assigned to (4S,5S)-2a and (4R,5R)-2a, respectively and those observed at 11.0 and 13.3 min to (4R,5S)-2a and (4S,5R)-2a, respectively. The isolation and enantiomeric composition analysis of cis-2b–d and trans-2b–d were made in the same manner as described above [retention times of HPLC signals for the other enantiomers: (4S,5S)-2b = 68.3, (4R,5R)-2b = 107.0, (4R,5S)-2b = 30.7, (4S,5R)-2b = 46.4 min; (4S,5S)-2c = 51.4, (4R,5R)-2c = 79.3, (4R,5S)-2c = 30.6, (4S,5R)-2c = 42.2 min; (4S,5S)-2d = 35.7, (4R,5R)-2d = 26.7, (4R,5S)-2d = 6.9, (4S,5R)-2d = 15.3 min].
In Table 1 are collected data on the conversions of 1 and the 1H NMR compositions of (Z)-1, (E)-1, cis-2,
trans-2, and 3, obtained by irradiation of nitrogen-saturated 1,2-dichloroethane [(Z)-1a,d] or 1,2-dichloroethane-10 vol% methanol [(Z)-1b,c] solutions of (Z)-1 (4.0´10–3 mol dm–3) containing S-MPM (0.10 mol dm–3) at room temperature. In addition, substituent and chiral amine effects on the conversions of 1 and the HPLC compositions of the (4S,5S)- and (4R,5R)-enantiomers for cis-2 and the (4S,5R)- and (4R,5S)-enantiomers for trans-2 are summarized in Table 2 along with the ee values for these two isomers. The data collected in Table 1 show that there is a great difference in photoreactivity among the hydroxyphenyl-substituted alaninamide derivatives (Z)-1a–c in which the reactivity of (Z)-1b having the 3-hydroxyphenyl group is much higher than that of the other derivatives and also is comparable to that of the parent derivative (Z)-1d bearing the unsubstituted phenyl group. If we consider a strong hydrogen-bonding interaction between the basic nitrogen in S-MPM and the acidic hydroxy hydrogen in (Z)-1a and (Z)-1c, this interaction lowering the effective concentration of the chiral amine is very likely to be responsible for the decreased photoreactivity of these derivatives. As demonstrated in our previous papers,9 the presence of an electron-donating methoxy group at the meta position on the benzene ring of N-acyl-α-dehydrophenylalaninamides greatly accelerated their PET-initiated cyclization reactions and also affected their cyclization pathways. It is thus reasonable to interpret the enhanced photoreactivity of (Z)-1b as being due to the so-called ‘meta effect’ of the hydrogen-bonded hydroxy group in this derivative.10
Further inspection of the data in Table 1 reveals that while irradiation of (Z)-1b–c affords the cis-dihydrooxazole isomer in preference to the trans-isomer, these two isomers are formed in comparable
composition to each other from the photocyclization of the 2-hydroxyphenyl-substituted alaninamide derivative (Z)-1a. On the basis of a mechanism (for formation of 2) proposed in our previous studies,4,6 we are able to assume that the composition ratio of cis-2 to trans-2 is determined by the relative rate of hydrogen shifts in the re and si faces of the cyclized biradical intermediate III, as illustrated in Scheme 2. Because these hydrogen shifts result in a preferential formation of the thermodynamically less stable cis-dihydrooxazole isomer and, hence, are kinetically-controlled process, both of the 1-naphthyl and hydroxyanilinocarbonyl groups attached to the oxazole ring are considered to preferentially exist in either of the two faces to exert a large steric hindrance to hydrogen shift in the face. The composition ratios of cis-2b–d to trans-2b–d are consistent with the above kinetically-controlled process but the ratio of cis-2a to trans-2a is not. The fact that the relative composition of cis-2a is greatly decreased in the (Z)-1a-derived dihydrooxazole isomer suggests the occurrence of a steric hindrance to the hydrogen shift that affords the cis-dihydrooxazole isomer. Taking into account the ability of the NH nitrogen in III to form an intramolecular hydrogen bond with the phenolic hydroxy hydrogen of this intermediate, we propose that such a hydrogen bond should be formed so as to minimize a steric repulsion between the
hydrogen-bonded hydroxyphenyl group and the 1-naphthyl group and, hence, so as to enhance the relative rate of the hydrogen shift forming the trans-isomer, as illustrated in Figure 1.
On the other hand, substituent effects on the magnitude of ee for cis-2b–d and trans-2b–d estimated in the presence of S-MPM demonstrate that a greater ee is obtained for the latter isomer than for the former and, additionally, the PET-initiated cyclization of (Z)-1a affords the corresponding dihydrooxazole isomers in less than 1% ee (Table 2). While asymmetric induction in this cyclization process was not observed also in the presence of S-PM and R-PM, these secondary chiral amines induced the asymmetric cyclization of (Z)-1d affording the corresponding cis-isomer in higher ee than S-MPM (S-PM: 25% ee, R-PM: 58% ee, and S-MPM: 17% ee). As already described, asymmetry in the dihydrooxazole isomer is induced at the steps of both the transformation of the radical ion pair (E)-I into the cyclized radical ion pair II and the hydrogen shift in the biradical III (Scheme 2).6 Because configurational interconversions between IIA and IIB and also between IIIA and IIIB are very unlikely to occur during the irradiation, it is possible to estimate the rate ratio of Path A1 to Path A2 (A1/A2), (namely, the extent to which asymmetry in the former step is induced) based on the composition of each enantiomer for cis-2 and trans-2 (A1/A2 = composition ratio of IIIA to IIIB). S-MPM was chosen as a typical chiral amine and the A1/A2 was calculated from the ratio of the sum of compositions for (4S,5S)-2 and (4R,5S)-2 (IIIA) to that for (4R,5R)-2 and (4S,5R)-2 (IIIB). The 1H NMR and HPLC compositions of these enantiomers, collected in Tables 1 and 2, made it possible to determine the order of this A1/A2: (Z)-1a (A1/A2 = 1.0) < (Z)-1b (1.1) < (Z)-1c (1.2) < (Z)-1d (1.5).
Our previous studies suggested that π-face selectivity in the cyclization of (E)-I is closely associated not only with the existence of the chiral amine-derived radical cation at the site of this cyclization,6 but also with the preferential existence of the arylaminocarbonyl group in either the re or si face.8 Thus, the observed order of A1/A2 led us to propose that hydrogen bonding and charge transfer interactions in the radical ion pair (E)-I, shown in Figure 2, are both key factors that lower the π-face selectivity. In other words, this selectivity is decreased with increasing the planarity of the (E)-1-derived radical anion (caused by the above hydrogen bonding interaction) as well as the distance between the S-MPM-derived
radical cation and the site at which the cyclization forming II occurs. Additionally, no occurrence of the enantioselective photocyclization in (Z)-1a having the 2-hydroxyanilinocarbonyl group substantiates that rates for the hydrogen shifts in Paths B1 and B2 are approximately equal to those in Paths C1 and C2, respectively. This may be due to the participation of another intramolecular hydrogen bond depicted in Figure 1. In conclusion, on the basis of the unexpected finding that the secondary chiral prolinol R-PM enhances the π-face selectivity described above (A1/A2 = 2.2), we suggest that in addition to the structural improvement of PM, an increase in the steric bulkiness of the arylaminocarbonyl group in (Z)-1 enables our PET-initiated cyclization to proceed in an even greater ee.
EXPERIMENTAL
1H and 13C NMR spectra were taken with a JEOL JNM-A500 spectrometer. Chemical shifts were determined using tetramethylsilane as an internal standard. ESI-TOF mass spectra were measured with a JEOL JMS-T100LC AccuTOF mass spectrometer. Elemental analyses were performed on a Perkin-Elmer PE2400II CHNS/O analyzer. Circular dichroism spectra were recorded on a Nihonbunko J-820 spectropolarimeter. HPLC analysis was performed on a Shimadzu LC-10AT high-performance liquid chromatography system equipped with a Daicel CHIRALPAK IA column and a Shimadzu SPD-10A UV detector. Methanol and 1,2-dichloroethane were purified according to the standard procedures and freshly distilled prior to use.11 All other reagents including chiral amines were obtained from commercial sources and were of the highest grade available.
General procedure for the synthesis of (Z)-4-(1-naphthylmethylene)-2-phenyl-5(4H)-oxazolone
This oxazolone derivative was synthesized by the Knoevenagel-type condensation reaction between 1-naphthaldehyde and N-benzoylglycine in acetic anhydride containing sodium acetate, described in a previous paper.7 The NMR spectral data were consistent with those of the previously prepared oxazolone derivative.
General procedure for the synthesis of (Z)-N-benzoyl-α-dehydro(1-naphthyl)alanine N’-arylamides [(Z)-1a–d]
To a solution of (Z)-4-(1-naphthylmethylene)-2-phenyl-5(4H)-oxazolone (5.0 mmol) in 20 mL tetrahydrofuran was added aminophenol or aniline (10 mmol) and the resulting mixture was heated under reflux for 24 h. The mixture was concentrated to dryness under reduced pressure affording the solid residual, which was dissolved in 100 mL ethyl acetate. The ethyl acetate solution was washed with 2 mol dm–3 hydrochloric acid (50 mL´2) and then with water (50 mL), dried over sodium sulfate, and filtered. The crystalline solid obtained by removing the solvent under reduced pressure was recrystallized from ethyl acetate-hexane to give analytica-grade (Z)-1 in 60–84% yield.
(Z)-2-Benzoylamino-N-(2-hydroxyphenyl)-3-(1-naphthyl)-2-propenamide [(Z)-1a]: mp 180.0–180.5 °C; 1H NMR (500 MHz, DMSO-d6) δ = 6.85 (1H, dd, J = 7.4, 7.4 Hz), 6.86 (1H, d, J = 7.4 Hz), 6.96 (1H, dd, J = 7.4, 8.0 Hz ), 7.48 (2H, dd, J = 7.4, 8.0 Hz ), 7.48 (1H, dd, J = 8.0, 8.0 Hz), 7.56 (1H, dd, J = 7.4, 6.9 Hz), 7.57 (1H, dd, J = 7.4, 8.6 Hz ), 7.59 (1H, dd, J = 7.4, 8.0 Hz), 7.66 (1H, d, J = 6.9 Hz), 7.86 (2H, d, J = 7.4 Hz), 7.92 (1H, d, J = 8.6 Hz ), 7.97 (1H, d, J = 7.4 Hz), 8.03 (1H, s ), 8.07 (1H, d, J = 8.0 Hz), 8.14 (1H, d, J = 8.0 Hz), 9.17 (1H, s ), 9.96 (1H, s), 10.16 (1H, s); 13C NMR (125 MHz, DMSO-d6) δ = 115.3, 119.6, 120.7, 124.6, 124.8, 125.8, 126.53, 126.57, 126.63, 127.0, 128.0 (2C), 128.7, 128.8 (2C), 128.9, 129.3, 131.4, 131.5, 132.2, 132.3, 133.5, 133.7, 147.3, 162.9, 167.4. Anal. Calcd for C26H20N2O3: C, 76.45; H, 4.94; N 6.86. Found: C, 76.45; H, 4.79; N, 6.86.
(Z)-2-Benzoylamino-N-(3-hydroxyphenyl)-3-(1-naphthyl)-2-propenamide [(Z)-1b]: mp 217.5–219.0 °C; 1H NMR (500 MHz, DMSO-d6) δ = 6.50 (1H, d, J = 8.0 Hz), 7.11 (1H, dd, J = 8.0, 8.0 Hz), 7.17 (1H, d, J = 8.0 Hz), 7.39 (1H, s), 7.45 (2H, dd, J = 7.5, 8.0 Hz), 7.48 (1H, dd, J = 7.5, 7.5 Hz), 7.52–7.57 (3H, m), 7.64 (1H, s), 7.70 (1H, d, J = 7.0 Hz), 7.86 (2H, d, J = 7.5 Hz), 7.90 (1H, d, J = 9.0 Hz), 7.94–7.97 (1H, m), 8.12 (1H, m), 9.40 (1H, s), 9.94 (1H, s), 10.14 (1H, s); 13C NMR (125 MHz, DMSO-d6) δ = 107.3, 110.6, 111.0, 124.5, 125.0, 125.5, 126.1, 126.4, 126.6, 127.9 (2C), 128.2 (2C), 128.5, 128.6, 129.1, 131.1, 131.4, 131.7, 133.27, 133.28, 133.6, 140.4, 157.5, 164.0, 166.3. ESI-TOF-MS m/z calcd for C26H20N2O3: 431.1371 [M + Na]+. Found: 431.1367.
(Z)-2-Benzoylamino-N-(4-hydroxyphenyl)-3-(1-naphthyl)-2-propenamide [(Z)-1c]: mp 175.5–176.5 °C; 1H NMR (500 MHz, DMSO-d6) δ = 6.74 (2H, d, J = 8.5 Hz), 7.44 (2H, dd, J = 7.5, 8.0 Hz), 7.48 (1H, dd, J = 7.5, 7.5 Hz), 7.51–7.57 (5H, m), 7.67 (1H, s), 7.69 (1H, d, J = 8.5 Hz), 7.86 (2H, d, J = 8.0 Hz), 7.88 (1H, d, J = 8.0 Hz), 7.93–7.96 (1H, m), 8.10–8.13 (1H, m), 9.24 (1H, s), 9.88 (1H, s), 9.99 (1H, s); 13C NMR (125 MHz DMSO-d6) δ = 115.0 (2C), 122.2 (2C), 124.6, 125.1, 125.5, 126.1, 126.4, 126.5, 128.0 (2C), 128.3 (2C), 128.5, 128.6, 131.0, 131.2, 131.6, 131.7, 133.3 133.4, 133.8, 153.7, 163.6, 166.4 . Anal. Calcd for C26H20N2O3: C, 76.45; H, 4.94; N 6.86. Found: C, 76.38; H, 4.84; N, 6.96.
(Z)-2-Benzoylamino-3-(1-naphthyl)-N-phenyl-2-propenamide [(Z)-1d]: mp 223.0–224.5 °C; 1H NMR (500 MHz, DMSO-d6) δ = 7.09 (1H, dd, J = 7.5, 7.5 Hz), 7.35 (2H, dd, J = 7.5, 7.5 Hz), 7.45 (2H, dd, J = 7.5, 7.5 Hz), 7.50 (1H, dd, J = 7.5, 7.5 Hz), 7.52–7.57 (3H, m), 7.67 (1H, s), 7.70 (1H, d, J = 7.0 Hz), 7.79 (2H, d, J = 7.5 Hz), 7.87 (2H, d, J = 7.5 Hz), 7.90 (1H, d, J = 8.0 Hz), 7.95–7.97 (1H, m), 8.12–8.14 (1H, m), 9.96 (1H, s), 10.27 (1H, s); 13C NMR (125 MHz DMSO-d6) δ = 120.1 (2C), 123.4, 124.5, 125.0, 125.5, 126.1, 126.4, 126.5, 127.9 (2C), 128.2 (2C), 128.45, 128.51 (2C), 128.6, 131.1, 131.4, 131.7, 133.21, 133.24, 133.5, 139.3, 164.1, 166.3. Anal. Calcd for C26H20N2O2: C, 79.57; H, 5.14; N 7.14. Found: C, 79.60; H, 5.06; N, 7.08.
General procedure for the irradiation of (Z)-1a–d
To isolate and characterize the (Z)-1-derived photoproducts, nitrogen-saturated 1,2-dichloroethane [(Z)-1a,d] or 1,2-dichloroethane-10 vol% methanol [(Z)-1b,c] solutions of (Z)-1 (4.0´10–3 mol dm–3, 10 mL´10) containing S-MPM (0.10 mol dm–3), placed in Pyrex test tubes, were irradiated in parallel for 3 h [(Z)-1b,d] or 12 h [(Z)-1a,c] at wavelengths longer than 280 nm from a 400 W high-pressure Hg lamp (set in a Pyrex cooling jacket). Parallel irradiation of the solutions was carried out at rt on a merry-go-round-type irradiation equipment immersed into a water bath (RIKO model RH400-10W). To isolate (E)-1, the nitrogen-saturated solutions of (Z)-1 (4.0´10–3 mol dm–3, 10 mL´5) were irradiated for 1 h in the same way as above. After irradiation, an aliquot (10 mL) of the irradiated solutions was concentrated to dryness under reduced pressure giving the solid residual, which was dissolved in DMSO-d6 and subjected to 1H NMR spectral analysis. The remaining irradiated solutions (10 mL´9 or 10 mL´4) were combined, washed with 0.2 mol dm–3 hydrochloric acid (50 mL´2) and then with water (50 mL), dried over sodium sulfate, and filtered. The residue obtained by removing the solvent under reduced pressure was subjected to preparative thin layer chromatography over silica gel with the use of chloroform-methanol (20:1 v/v) as a developing solvent.
Moreover, to determine the absolute configuration and enantiomeric excess of the cis-2a–d- and trans-2a–d-derived enantiomers, the 4,5-dihydrooxazole isomers isolated were analyzed by high-performance liquid chromatographic apparatus equipped with a 4.6´250-mm Daicel CHIRALPAK IA column using the mixture of 2-propanol (5 vol%), chloroform (15 vol%), and n-hexane (80 vol%) as a mobile phase (detection wavelength, 250 nm; flow rate, 1.0 mL min–1). Repeated HPLC separation of the cis-2- and trans-2-derived enantiomers made it possible to isolate these enantiomers along with determination of their retention times and relative compositions. The absolute configuration of each enantiomer was determined by comparing its circular dichroism spectrum in methanol with that of (4S,5S)-4-(tert-butoxycarbonyl)-5-(1-naphthyl)-2-phenyl-4,5-dihydrooxazole, (4S,5S)- or (4R,5R)-4-(2-aminocarbonylpyrrolidin-1-ylcarbonyl)-5-(1-naphthyl)-2-phenyl-4,5-dihydrooxazole described in our previous papers.6,8
cis-4-(2-Hydroxyanilinocarbonyl)-5-(1-naphthyl)-2-phenyl-4,5-dihydrooxazole (cis-2a): 1H NMR (500 MHz, DMSO-d6) δ = 5.67 (1H, d, J = 11.0 Hz), 6.46 (1H, dd, J = 7.5, 7.5 Hz ), 6.71 (1H, d, J = 8.0 Hz), 6.76 (1H, dd, J = 7.5, 8.0 Hz), 6.96 (1H, d, J = 11.0 Hz ), 7.00 (1H, d, J = 8.0 Hz), 7.40 (1H, dd, J = 7.5, 8.0 Hz ), 7.45 (1H, d, J = 7.5 Hz), 7.52 (1H, dd, J = 8.0, 8.0 Hz), 7.56 (1H, dd, J = 7.5, 8.0 Hz ), 7.61 (2H, dd, J = 7.5, 7.5 Hz), 7.69 (1H, dd, J = 7.5, 7.5 Hz), 7.83 (1H, d, J = 8.0 Hz), 7.91 (1H, d, J = 7.5 Hz ), 8.11 (2H, d, J = 7.5 Hz), 8.18 (1H, d, J = 8.0 Hz ), 8.96 (1H, s), 9.74 (1H, s); 13C NMR (125 MHz, DMSO-d6) δ = 73.6, 80.3, 114.8, 118.7, 120.2, 123.2, 123.9, 124.2, 125.0, 125.3, 125.7, 126.1, 126.7, 128.3 (2C), 128.3, 128.4, 129.0 (2C), 130.4, 132.4, 132.76, 132.79, 146.8, 165.6, 166.2. ESI-TOF-MS m/z calcd for C26H20N2O3: 409.1552 [M + H]+. Found: 409.1591.
cis-4-(3-Hydroxyanilinocarbonyl)-5-(1-naphthyl)-2-phenyl-4,5-dihydrooxazole (cis-2b): 1H NMR (500 MHz, DMSO-d6) δ = 5.59 (1H, d, J = 10.5 Hz), 6.28 (1H, d, J = 8.0 Hz), 6.31 (1H, d, J = 8.0 Hz), 6.52 (1H, s), 6.81 (1H, dd, J = 8.0, 8.0 Hz), 6.86 (1H, d, J = 10.5 Hz), 7.45 (1H, dd, J = 7.5, 8.0 Hz), 7.49 (1H, dd, J = 7.0, 7.5 Hz), 7.56 (1H, dd, J = 7.5, 8.0 Hz), 7.59 (2H, dd, J = 7.5, 7.5 Hz), 7.62 (1H, d, J = 7.0 Hz), 7.66 (1H, dd, J = 7.5, 7.5 Hz), 7.80 (1H, d, J = 8.0 Hz), 7.86 (1H, d, J = 8.0 Hz), 8.10 (2H, d, J = 7.5 Hz), 8.11 (1H, d, J = 7.5 Hz), 9.18 (1H, s), 9.47 (1H, s). 13C NMR (125 MHz, DMSO-d6) δ = 73.8, 80.5, 107.0, 110.60, 110.62, 123.5, 123.7, 125.0, 125.6, 126.0, 127.2, 128.0, 128.32 (2C), 128.35, 128.78 (2C), 128.80, 129.8, 132.1, 132.6, 132.7, 138.8, 157.1, 164.9, 166.1. ESI-TOF-MS m/z calcd for C26H20N2O3: 409.1552 [M + H]+. Found: 409.1548.
cis-4-(4-Hydroxyanilinocarbonyl)-5-(1-naphthyl)-2-phenyl-4,5-dihydrooxazole (cis-2c): 1H NMR (500 MHz, DMSO-d6) δ = 5.53 (1H, d, J = 10.5 Hz), 6.40 (2H, d, J = 8.5 Hz), 6.54 (2H, d, J = 8.5 Hz), 6.86 (1H, d, J = 10.5 Hz), 7.45 (1H, dd, J = 7.0, 8.0 Hz), 7.50 (1H, dd, J = 7.5, 8.0 Hz), 7.55 (1H, dd, J = 8.0, 8.0 Hz), 7.57–7.61 (3H, m), 7.66 (1H, dd, J = 7.5, 8.5 Hz), 7.81 (1H, d, J = 8.0 Hz), 7.88 (1H, d, J = 7.5 Hz), 8.10 (2H, d, J = 8.0 Hz), 8.11 (1H, d, J = 7.0 Hz), 9.09 (1H, s), 9.28 (1H, s); 13C NMR (125 MHz, DMSO-d6) δ = 73.7, 80.5, 114.5 (2C), 122.2 (2C), 123.6, 123.8, 125.0, 125.6, 126.0, 127.2, 128.0, 128.29, 128.33 (2C), 128.7 (2C), 129.1, 129.9, 132.0, 132.7, 132.8, 153.6, 164.9, 165.8. ESI-TOF-MS m/z calcd for C26H20N2O3: 409.1552 [M + H]+. Found: 409.1576.
cis-4-Anilinocarbonyl-5-(1-naphthyl)-2-phenyl-4,5-dihydrooxazole (cis-2d): 1H NMR (500 MHz, DMSO-d6) δ = 5.61 (1H, d, J = 11.0 Hz), 6.85 (2H, d, J = 7.5 Hz), 6.87 (1H, dd, J = 7.5, 7.5 Hz), 6.89 (1H, d, J = 11.0 Hz), 7.03 (2H, dd, J = 7.5, 7.5 Hz), 7.45 (1H, dd, J = 7.5, 7.5 Hz), 7.48 (1H, d, J = 7.0 Hz), 7.56 (1H, dd, J = 7.0, 7.0 Hz), 7.59 (2H, dd, J = 7.5, 8.0 Hz), 7.62 (1H, d, J = 7.0 Hz), 7.67 (1H, dd, J = 7.5, 8.0 Hz), 7.79 (1H, d, J = 8.0 Hz), 7.85 (1H, d, J = 8.0 Hz), 8.11 (2H, d, J = 8.0 Hz), 8.12 (1H, dd, J = 8.0, 8.0 Hz), 9.59 (1H, s); 13C NMR (125 MHz, DMSO-d6) δ = 73.8, 80.5, 120.1 (2C), 123.5, 123.7, 125.0, 125.6, 126.0, 127.1, 128.0, 128.1 (2C), 128.28, 128.32 (2C), 128.8 (2C), 128.9, 129.8, 132.1, 132.5, 132.7, 137.6, 165.6, 166.2. ESI-TOF-MS m/z calcd for C26H20N2O2: 415.1423 [M + Na]+. Found: 415.1530.
trans-2a: 1H NMR (500 MHz, DMSO-d6) δ = 5.16 (1H, d, J = 6.5 Hz ), 6.74 (1H, d, J = 6.5 Hz ), 6.84 (1H, dd, J = 7.5, 8.0 Hz), 6.91 (1H, d, J = 8.0 Hz), 6.98 (1H, dd, J = 7.5, 8.0 Hz), 7.53–7.63 (5H, m), 7.64 (1H, dd, J = 7.0, 8.0 Hz), 7.68 (1H, dd, J = 7.5, 7.5 Hz), 7.98 (1H, dd, J = 8.0, 8.5 Hz), 8.03 (1H, d, J = 8.5 Hz ), 8.12 (2H, d, J= 7.0 Hz), 8.13 (1H, d, J = 8.0 Hz), 8.33 (1H, d, J = 6.5 Hz), 9.40 (1H, s), 10.10 (1H, s); 13C NMR (125 MHz, DMSO-d6)δ = 76.1, 81.0, 115.0, 119.1, 120.6, 122.8, 123.1, 124.6, 125.4, 125.8 126.1, 126.5, 126.7, 128.2 (2C), 128.87, 128.89, 129.0 (2C), 129.5, 132.5, 133.6, 135.0, 147.1, 164.2, 168.7. ESI-TOF-MS m/z calcd for C26H20N2O3: 409.1552 [M + H]+. Found: 409.1590.
trans-2b: 1H NMR (500 MHz, DMSO-d6) δ = 4.86 (1H, d, J = 6.5 Hz), 6.51 (1H, d, J = 8.0 Hz), 6.70 (1H, d, J = 6.5 Hz), 7.03 (1H, d, J = 8.0 Hz), 7.12 (1H, dd, J = 8.0, 8.0 Hz), 7.25 (1H, s), 7.55–7.61 (6H, m), 7.66 (1H, dd, J = 7.5, 8.0 Hz), 7.84–7.87 (1H, m), 7.98 (1H, m), 8.03–8.06 (1H, m), 8.08 (2H, d, J = 7.5 Hz), 9.47 (1H, s), 10.27 (1H, s); 13C NMR (125 MHz, DMSO-d6) δ = 78.0, 81.2, 107.2, 110.8, 111.6, 123.0, 123.3, 126.1, 126.8, 127.4, 128.8 (2C), 129.36, 129.39, 129.5 (2C), 129.6, 129.8, 130.0, 132.9, 134.1, 135.7, 140.1, 158.2, 164.3, 168.7. ESI-TOF-MS m/z calcd for C26H20N2O3: 409.1552 [M + H]+. Found: 409.1634.
trans-2c: 1H NMR (500 MHz, CDCl3) δ = 4.75 (1H, s), 4.93 (1H, d, J = 5.4 Hz), 6.80 (1H, d, J = 5.4 Hz), 6.82 (2H, d, J= 9.0 Hz), 7.44 (1H, dd, J = 7.2, 7.8 Hz), 7.47 (2H, d, J = 9.0 Hz), 7.48 (1H, dd, J = 7.2, 7.2 Hz), 7.52–7.56 (3H, m), 7.60–7.64 (2H, m), 7.85 (1H, d, J = 7.8 Hz), 7.91 (1H, d, J = 7.8 Hz), 8.20 (2H, d, J = 7.8 Hz), 8.35 (1H, s), 8.65 (1H, d, J = 7.8 Hz); 13C NMR (125 MHz, DMSO-d6) δ = 77.3, 80.7, 115.1 (2C), 121.3 (2C), 122.1, 122.6, 122.7, 126.1, 126.6, 126.8, 128.3 (2C), 128.4, 128.7, 128.8 (2C), 129.0, 130.1, 130.4, 132.2, 135.2, 153.8, 163.7, 167.6. ESI-TOF-MS m/z calcd for C26H20N2O3: 409.1552 [M + H]+. Found: 409.1577.
trans-2d: 1H NMR (500 MHz, DMSO-d6) δ = 4.89 (1H, d, J = 6.9 Hz), 6.72 (1H, d, J = 6.9 Hz), 7.12 (1H, dd, J = 7.5, 7.5 Hz), 7.36 (2H, dd, J = 7.5, 7.5 Hz), 7.55–7.61 (6H, m), 7.67 (1H, dd, J = 7.5, 7.5 Hz), 7.68 (2H, d, J = 7.5 Hz), 7.88–7.90 (1H, m), 7.96–8.00 (1H, m), 8.04–8.06 (1H, m), 8.09 (2H, d, J = 7.5 Hz), 10.41 (1H, s); 13C NMR (125 MHz DMSO-d6) δ = 77.4, 80.6, 119.6 (2C), 122.5, 122.8, 124.0, 125.6, 126.2, 126.5, 126.9, 128.3 (2C), 128.7, 128.87 (2C), 128.94 (2C), 129.1, 129.3, 132.3, 133.6, 135.1, 138.5, 163.9, 168.3. ESI-TOF-MS m/z calcd for C26H20N2O2: 393.1603 [M + H]+. Found: 393.1660.
3-(2-Hydroxyanilinocarbonyl)-1-phenylbenzo[f]isoquinoline (3a): 1H NMR (500 MHz, DMSO-d6)δ = 6.88 (1H, dd, J = 7.5, 7.5 Hz), 6.92 (1H, d, J = 8.0 Hz ), 6.98 (1H, dd, J = 7.5, 8.0 Hz ), 7.62–7.69 (3H, m ), 7.83 (2H, d, J = 7.5 Hz ), 7.89–7.91 (2H, m ), 8.00 (1H, d, J = 9.0 Hz), 8.14 (1H, d, J = 9.0 Hz), 8.15–8.17 (1H, m ), 8.50 (1H, d, J = 7.5 Hz ), 9.10–9.12 (1H, m), 9.46 (1H, s), 10.29 (1H, s ), 10.82 (1H, s); 13C NMR (125 MHz, DMSO-d6)δ = 114.3, 114.7, 119.1, 119.4, 123.7, 124.1, 124.2, 125.8, 126.4, 128.4, 128.6 (3C), 128.8, 129.1, 129.7, 130.1 (2C), 130.4, 132.9, 136.3, 138.7, 144.2, 146.5, 158.5, 161.5. ESI-TOF-MS m/z calcd for C26H18N2O2: 391.1447 [M + H]+. Found: 391.1395.
3-(3-Hydroxyanilinocarbonyl)-1-phenylbenzo[f]isoquinoline (3b): 1H NMR (500 MHz, DMSO-d6) δ = 6.56 (1H, d, J = 8.0 Hz), 7.17 (1H, dd, J = 8.0, 8.0 Hz), 7.25 (1H, d, J = 8.0 Hz), 7.49 (1H, s), 7.62–7.68 (3H, m), 7.87–7.91 (4H, m), 8.00 (1H, d, J = 9.0 Hz), 8.12 (1H, d, J = 9.0 Hz), 8.14–8.17 (1H, m), 9.09–9.12 (1H, m), 9.42 (1H, s), 9.52 (1H, s), 10.43 (1H, s); 13C NMR(125 MHz, DMSO-d6) δ = 107.3, 111.1, 111.2, 114.6, 123.7, 124.2, 125.7, 128.3, 128.5 (2C), 128.6, 128.8, 129.0, 129.5, 129.7, 130.3, 130.4 (2C), 132.8, 136.2, 138.6, 139.2, 144.7, 157.7, 158.5, 162.7. ESI-TOF-MS m/z calcd for C26H18N2O2: 391.1447 [M + H]+. Found: 391.1558.
(E)-1a: 1H NMR (500 MHz, DMSO-d6)δ = 6.71 (1H, dd, J = 7.5, 8.0 Hz), 6.72 (1H, d, J = 8.0 Hz), 6.90 (1H, dd, J = 7.5, 8.0 Hz), 7.424 (1H, s ), 7.425 (1H, dd, J = 7.5, 8.0 Hz), 7.49 (1H, d, J = 8.0 Hz), 7.53 (1H, d, J = 6.5 Hz), 7.55–7.66 (3H, m), 7.57 (2H, dd, J = 7.0, 8.0 Hz), 7.85 (1H, d, J = 8.0 Hz ), 7.95 (1H, d, J = 8.5 Hz ), 8.02 (2H, d, J = 7.0 Hz), 8.13 (1H, d, J = 8.0 Hz), 8.94 (1H, s), 9.24 (1H, s), 10.59 (1H, s); 13C NMR (125 MHz, DMSO-d6) δ = 115.9, 118.8, 119.5, 122.2, 125.1, 125.6, 126.1, 126.2, 126.6, 126.7, 126.9, 128.4 (2C), 128.6, 129.0 (2C), 129.1, 131.8, 132.0, 132.7, 133.7, 133.9, 134.9, 148.5, 163.7, 165.9. ESI-TOF-MS m/z calcd for C26H20N2O3: 431.1371 [M + Na]+. Found: 431.1347.
(E)-1b: 1H NMR (500 MHz, DMSO-d6) δ = 6.38 (1H, d, J = 7.8 Hz), 6.78 (1H, d, J = 8.4 Hz), 6.97 (1H, dd, J = 7.8, 8.4 Hz), 7.04 (1H, s), 7.37 (1H, dd, J = 7.8, 7.8 Hz), 7.39 (1H, s), 7.52–7.64 (6H, m), 7.79 (1H, d, J = 8.4 Hz), 7.92 (1H, d, J = 7.8 Hz), 8.01 (2H, d, J = 7.8 Hz), 8.13 (1H, d, J = 8.4 Hz), 9.26 (1H, s), 9.98 (1H, s), 10.48 (1H, s). 13C NMR(125 MHz, DMSO-d6) δ = 107.0, 110.4, 110.6, 115.7, 124.6, 125.4, 125.6, 125.9, 126.1, 127.6, 127.8 (2C), 128.3, 128.5 (2C), 129.0, 131.3, 131.8, 131.9, 133.1, 133.6, 135.0, 140.1, 157.3, 163.1, 164.9. ESI-TOF-MS m/z calcd for C26H20N2O3: 409.1552 [M + H]+. Found: 409.1563.
(E)-1c: 1H NMR (500 MHz, DMSO-d6) δ = 6.61 (2H, d, J = 9.0 Hz), 7.18 (2H, d, J = 9.0 Hz), 7.385 (1H, dd, J = 7.5, 8.0 Hz), 7.391 (1H, s), 7.53–7.64 (6H, m), 7.79 (1H, d, J = 8.0 Hz), 7.92 (1H, d, J = 8.5 Hz), 8.00 (2H, d, J = 7.5 Hz), 8.12 (1H, d, J = 8.5 Hz), 9.14 (1H, s), 9.79 (1H, s), 10.43 (1H, s). 13C NMR (125 MHz, DMSO-d6) δ = 114.8 (2C), 115.6, 121.7 (2C), 124.6, 125.4, 125.6, 125.9, 126.1, 127.4, 127.8 (2C), 128.3, 128.4 (2C), 130.6, 131.3, 131.8, 131.9, 133.1, 133.7,135.1, 153.5, 162.7, 164.9. ESI-TOF-MS m/z calcd for C26H20N2O3: 409.1552 [M + H]+. Found: 409.1615.
(E)-1d: 1H NMR (500 MHz, DMSO-d6) δ = 6.99 (1H, dd, J = 7.5, 7.5 Hz), 7.21 (2H, dd, J = 8.0, 8.0 Hz), 7.37 (1H, dd, J = 7.5, 8.0 Hz), 7.40 (1H, s), 7.42 (2H, d, J = 7.5 Hz), 7.53–7.63 (6H, m), 7.78 (1H, d, J = 8.0 Hz), 7.92 (1H, d, J = 7.5 Hz), 8.01 (2H, d, J = 7.5 Hz), 8.14 (1H, d, J = 8.5 Hz), 10.10 (1H, s), 10.51 (1H, s); 13C NMR (125 MHz, DMSO-d6) δ = 115.9, 119.8 (2C), 123.2, 124.6, 125.4, 125.6, 125.9, 126.1, 127.6, 127.8 (2C), 128.3, 128.4 (2C), 128.5 (2C), 131.3, 131.7, 131.9, 133.1, 133.6, 134.9, 139.1, 163.2, 164.9. ESI-TOF-MS m/z calcd for C26H20N2O2: 393.1603 [M + H]+. Found: 393.1642.
ACKNOWLEDGEMENTS
This research was partially supported by a “Scientific Frontier Research Project” from the Ministry of Education, Sports, Culture, Science and Technology, Japan.
References
1. a) S. R. L. Everitt and Y. Inoue, ‘Organic Molecular Photochemistry,’ ed. by V. Ramamurthy and K. S. Schanze, Marcel Dekker, New York, 1999, pp. 71–130; b) A. G. Griesbeck and U. J. Meierhenrich, Angew. Chem. Int. Ed., 2002, 41, 3147; CrossRef c) Y. Inoue, ‘Chiral Photochemistry,’ ed. by Y. Inoue and V. Ramamurthy, Marcel Dekker, New York, 2004, pp. 129–177; CrossRef d) N. Hoffmann and J.-P. Pete, ‘Chiral Photochemistry,’ ed. by Y. Inoue and V. Ramamurthy, Marcel Dekker, New York, 2004, pp. 179–233; CrossRef e) C. Müller and T. Bach, Aust. J. Chem., 2008, 61, 557; CrossRef f) N. Hoffmann, Chem. Rev., 2008, 108, 1052. CrossRef
2. a) ‘Synthetic Organic Photochemistry,’ ed. by W. M. Horspool, Plenum, New York, 1984; b) ‘Synthetic Organic Photochemistry,’ ed. by A. G. Griesbeck and J. Mattay, Marcel Dekker, New York, 2005; c) A. G. Griesbeck, N. Hoffmann, and K. Warzecha, Acc. Chem. Res., 2007, 40, 128. CrossRef
3. a) K. Maekawa, T. Igarashi, K. Kubo, and T. Sakurai, Tetrahedron, 2001, 57, 5515; CrossRef b) T. Motohashi, K. Maekawa, K. Kubo, T. Igarashi, and T. Sakurai, Heterocycles, 2002, 57, 269; CrossRef c) K. Maekawa, H. Kajiwara, Y. Iseya, T. Igarashi, and T. Sakurai, Heterocycles, 2003, 60, 637; CrossRef d) K. Maekawa, A. Shinozuka, M. Naito, T. Igarashi, and T. Sakurai, Tetrahedron, 2004, 60, 10293; CrossRef e) K. Maekawa, K. Fujita, K. Iizuka, T. Igarashi, and T. Sakurai, Heterocycles, 2005, 65, 117. CrossRef
4. a) K. Maekawa, T. Sasaki, K. Kubo, T. Igarashi, and T. Sakurai, Tetrahedron Lett., 2004, 45, 3663; CrossRef b) K. Maekawa, N. Hishikawa, K. Kubo, T. Igarashi, and T. Sakurai, Tetrahedron, 2007, 63, 11267. CrossRef
5. a) K. Maekawa, K. Kubo, T. Igarashi, and T. Sakurai, Heterocycles, 2002, 57, 1591; CrossRef b) K. Maekawa, K. Kubo, T. Igarashi, and T. Sakurai, Tetrahedron, 2004, 60, 1183; CrossRef c) K. Maekawa, K. Kubo, T. Igarashi, and T. Sakurai, Tetrahedron, 2005, 61, 11211. CrossRef
6. Y. Sasaki, H. Watanabe, T. Igarashi, and T. Sakurai, Heterocycles, 2011, 83, 1329. CrossRef
7. Y. Sato, A. Yoshida, T. Igarashi, and T. Sakurai, Heterocycles, 2010, 81, 997. CrossRef
8. Y. Sato, Y. Haruyama, T. Igarashi, and T. Sakurai, Heterocycles, 2010, 82, 603. CrossRef
9. a) K. Maekawa, H. Kajiwara, Y. Iseya, T. Igarashi, and T. Sakurai, Heterocycles, 2003, 60, 637; CrossRef b) K. Maekawa, K. Fujita, K. Iizuka, T. Igarashi, and T. Sakurai, Heterocycles, 2005, 65, 117; CrossRef c) Y. Yazawa, M. Suzuki, T. Igarashi, and T. Sakurai, Heterocycles, 2010, 80, 199. CrossRef
10. a) H. E. Zimmerman and V. R. Sandel, J. Am. Chem. Soc., 1963, 85, 915; CrossRef b) H. E. Zimmerman, J. Am. Chem. Soc., 1995, 117, 8988; CrossRef c) H. E. Zimmerman, J. Phys. Chem. A, 1998, 102, 5616. CrossRef
11. J. A. Riddick, W. B. Bunger, and T. K. Sakano, ‘Organic Solvents,’ 4th ed., Wiley, Chichester, 1986.