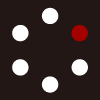
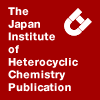
HETEROCYCLES
An International Journal for Reviews and Communications in Heterocyclic ChemistryWeb Edition ISSN: 1881-0942
Published online by The Japan Institute of Heterocyclic Chemistry
e-Journal
Full Text HTML
Received, 8th April, 2011, Accepted, 13th May, 2011, Published online, 24th May, 2011.
DOI: 10.3987/COM-11-12230
■ A Practical Synthesis of Novel Rho-Kinase Inhibitor, (S)-4-Fluoro-5-(2-methyl-1,4-diazepan-1-ylsulfonyl)isoquinoline
Noriaki Gomi, Tadaaki Ohgiya, Kimiyuki Shibuya,* Jyunji Katsuyama, Masayuki Masumoto, and Hitoshi Sakai
Pharmaceutical Division, Tokyo New Drug Research Laboratories, Kowa Co., Ltd., 2-17-43, Noguchicho, Higashimurayama, Tokyo 189-0022, Japan
Abstract
A practical synthesis of novel Rho-kinase inhibitor, (S)-4-fluoro-5-(2-methyl-1,4-diazepan-1-ylsulfonyl)isoquinoline hydrochloride dihydrate (1) was achieved in a pilot-scale production. We have demonstrated the regioselective chlorosulfonylation of 4-fluoroisoquinoline in an one-pot reaction to afford 4-fluoroisoquinoline-5-sulfonyl chloride and the asymmetric construction of the (S)-2-methyl-1,4-diazepane moiety as key steps.INTRODUCTION
A highly potent and selective Rho-kinase inhibitor, (S)-4-fluoro-5-(2-methyl-1,4-diazepan-1-ylsulfonyl)- isoquinoline hydrochloride dihydrate (1)1 has been developed from Fasudil (2).2,3 Both compounds have the same core structure of 5-(1,4-diazepan-1-ylsulfonyl)isoquinoline as shown in Figure 1. Fasudil has been recognized as a potent Rho-kinase inhibitor and clinically used for the treatment of cerebral vasospasm.2 Furthermore the recent studies revealed that 2 could enhance memory and improve the prognosis of Alzheimer’s patients,3a angina3b and pulmonary arterial hypertension.3c After exploring the chemical derivatives of 2, the incorporation of fluorine atom at C4 position of isoquinoline moiety and the chiral attachment of the methyl group at C2’ position of 1,4-diazepane moiety dramatically improved the pharmacological action. As can be seen, 1 showed much more potent and selective Rho-kinase inhibitory activity than Fasudil1a and would be potentially expected to cure the Cerebral Vascular Disorder.1b Especially, it is noteworthy that 1 can be effective on the ocular hypertension with intraocular pressure (IOP)-lowering activity and neuroprotection of retinal ganglion cells injured in glaucoma.1a
As part of our drug research and development plans, we required the practical synthesis of 1 in multi-kilogram scale and be easily scalable to commercial scale. However, there are two major issues. First, we needed to develop a regioselective chlorosulfonylation reaction at C5 position of 4-fluoroisoquinoline. Second, we had to guarantee the stereochemical purity in (S)-2-methyl-1,4- diazepane moiety. Herein we disclose the synthesis of 1 starting from 4-fluoroisoquinoline at pilot-scale.
RESULTS AND DISCUSSION
In our retrosynthetic analysis (Scheme 1), we set out to synthesize the intermediate 3 and verify that the absolute stereochemistry at the C2’ position would be retained to form the enantiomerically pure (S)-2-methyl-1,4-diazepane moiety. Intramolecular cyclization of the sulfonamide alcohol (3) would afford 1 by a Mitsunobu reaction. The precursor of 3 could be identified the combination of 4 and readily available (S)-2-aminopropanol by the disconnection bond between C3’ position and N4’ position. The sulfonamide
alcohol (4) can be easily prepared from 4-fluoroisoquinoline-5-sulfonyl chloride (5). An efficient chlorosulfonylation of 4-fluoroisoquinoline (6)4 is the most important issue to provide 5.
Although several methods for synthesis of 5 were previously reported, these syntheses were not amenable due to many steps, low yields, troublesome purifications and tedious reaction conditions (e.g., diazonium salt intermediates).5 Accordingly, we needed to establish the reproducible synthesis of 5 from 6 that is applicable for a large-scale production. In order to prepare 5, we investigated a variety of reaction condition on the regioselective sulfonylation of 6 and its efficient purification.
Some methods for the synthesis of arylsulfonychlorides from the corresponding aromatics have been achieved by stepwise sulfonylation and subsequent chlorination with phosphorus pentachloride, phosphorus oxychloride, thionyl chloride,6 or directly with chlorosulfuric acid,6a,7 or treatment with a combination of sulfinic acid and NCS.8 These methodologies have been already applied to some isoquinolines.6b,6c,7b
We attempted to transform 6 into 7 with a combination of concd H2SO4 and SO3 to produce fuming sulfuric acid in situ (Table 1). The initial conditions by applying 2.2 eq of concd H2SO4 and 8.6 eq of SO3 took 18 h at 30 °C to complete (Table 1, Entries 1, 2). While the quantity of concd H2SO4 accelerated the reaction (Entries 1 - 3), the low concentration of SO3 decreased the reaction rate and the regioselectivity between the C5 position and the C8 position (Entries 4, 5). The presence of ca. 2 eq of concd H2SO4 seemed to be favorable in the sulfonylation reaction conditions. Using a vast excess of SO3, the sulfonylation yield was improved for the desired 7 at 0 °C to 15 °C for 4 h (Entries 6 - 8). However, these conditions were unsuitable for a large-scale production, since the bulk amounts of concd H2SO4 make it difficult and expensive to neutralize for waste disposal compared to Entry 2. In addition, it was too difficult to stir the viscous reaction mixtures under these conditions. On the other hand, heating the reaction to 50 °C facilitated the stirring, but eroded the regioselectivity (Entry 9). We also investigated the influences of added Lewis Acids on the sulfonylation reaction in combination with concd H2SO4 and SO3 system. Unfortunately, the separate addition of 1 eq of MgCl2, AlCl3, ZnCl2, or H3BO3 decreased the yield of 7 (Entries 10 - 13). Half of equivalent of FeCl3 or AgNO3 had no impact on the yield of 7 (Entries 14, 15), but FeCl3 did afford the highest regioselectivity in the reaction. In the procedure on a large-scale production, the handling of 6 was found to be troublesome because of its low melting point.4
Then we investigated the HSO4– salt of 6 for easy handling in the sulfonylation reaction. Remarkably, we found that the HSO4– salt of 6 was very effective in the reaction providing high yield as shown in Entry 16 with decreased H2SO4 equivalents and good regioselectivity. As the result, we selected the best conditions as Entry 16 and Entry 2 as a second choice for the sulfonylation of 6.
Next, we developed a concise one-pot reaction for production of the sulfonyl chloride (5) via the corresponding sulfonic acid (7) prepared in a combination of concd H2SO4 and SO3 from 6 and which was subsequently exposed upon SOCl2 (Table 2). Our first attempt for a simple procedure was as follows. The 4-fluoroisoquinoline (6) in SOCl2 was treated with a mixture of concd H2SO4 and SO3. This reaction was failed and gave only a trace amount of 5 and 9 with the recovered 6 (63%) (Table 2, Entry 1). Accordingly, we conducted stepwise the sulfonylation of 6 with concd H2SO4 and SO3 and subsequently the chlorination of the prepared 7 and 8 with SOCl2 in one-pot reaction (Entry 2). The similar result was obtained by application of HSO4– salt of 6 (Entry 3). The ratio of 7 and 8 was not changed in the chlorination process. Satisfactory results were obtained to afford extra pure 5 in 41% and 39% yield after crystallization as the HCl salt respectively. Fortunately, the 8-sulfonyl chloride (9) as by-product can be easily removed by filtration in the mother liquid during the crystallization procedure.
Thus obtained sulfonyl chloride 5 in one-pot reaction9 was further utilized in the synthesis of our Rho-kinase inhibitor (1) as depicted in Scheme 2. Sulfonylamidation of 5 with (S)-2-aminopropanol gave the corresponding sulfonylamide (4) in 84% yield. Mesylation of 4 led to a mixture of the mesylate (10) and the aziridine intermediate (11).10 N-Alkylation was carried out in situ, the mixture of 10 and 11 was directly treated with 3-aminopropanol to afford the desired 3 in a high yield. Two reaction pathways can be postulated for the nucleophilic attack on Site A or Site B of the aziridine. The ratio of the isomer 3b to 3a was greatly influenced by the reaction temperature. The desired addition (attacked from site A) was controlled by carrying out the reaction at low temperature.11 However, we had to remove 3b to the minimum at this stage. In order to avoid using the column chromatography separation especially in a large-scale production, we attempted to purify 3a by way of a crystalline oxalate salt. As expected, the oxalate salt of 3a was obtained as colorless crystals in 73% yield from 4. Thus we succeeded in minimizing the amount of the contaminating isomer 3b (attack from site B) to 0.3%.
After basifying of the oxalate salt of 3a to afford the free amine (3) (Scheme 3), the intramolecular cyclization was conducted by treating 3 under Mitsunobu conditions.12 The reaction proceeded smoothly to provide the desired product (12) in 90% yield (95% purity by HPLC). Finally, the purification of 1 was accomplished by the crystallization as the HCl salt in 73% yield over two steps (>99.9% ee). The crystalline 1 was sufficiently pure for GMP production and clinical use.
In conclusion, we have achieved the practical synthesis of novel Rho-kinase inhibitor, (S)-4-fluoro-5-(2-methyl-1,4-diazepan-1-ylsulfonyl)isoquinoline hydrochloride dihydrate (1) starting from 4-fluoroisoquinoline in a pilot-scale production. We developed and reported the concise one-pot chlorosulfonylation of 4-fluoroisoquinoline and the regioselective construction of the chiral 1,4-diazepane by a Mitsunobu reaction intramolecular cyclization as the key steps. Application of 1 for the treatment of ocular hypertension is currently under study.
EXPERIMENTAL
General. All reactions were carried out under a N2 atmosphere unless otherwise noted. Optical rotations were measured on a JASCO P-2200 polarimeter with sodium (D line) lamp. IR spectra were recorded on a Thermo Nicolet 370 FT-IR spectrometer. 1H-NMR spectra and 13C-NMR spectra were obtained on a JEOL JNM-AL 400 MHz spectrometer using CDCl3 or DMSO-d6 solvents with tetramethylsilane as internal standard unless otherwise noted. Mass spectra were obtained on a JEOL MS-BU20 mass spectrometer. Elemental analyses (C, H, N) were performed by Yanaco MT-5. Elemental analyses (Cl) were performed by Kyoto Electronics Manufacturing AT-510. Elemental analyses (S, F) were performed by Nippon Dionex ICS-1500. Melting points were determined in open glass capillaries on a Buchi B-545 melting point apparatus. The HPLC analyses were performed using Hitachi D-7000 equipment. The optical purity was determined by HPLC analysis (CHIRALPAK AD, Daicel Chemical Industries Limited).
4-Fluoroisoquinoline sulfate (6a)
To a solution of 4-fluoroisoquinoline (6)4 (9.40 kg, 63.9 mol) in acetone (28 kg) was carefully and gradually added concd H2SO4 (6.58 kg, 67.1 mol) at 0 – 10 °C, and the mixture was stirred at the same temperature for 2 h. The precipitated crystals were filtered, washed with acetone (22 kg), and dried under reduced pressure to yield 6a (15.5 kg, 99%) as a colorless crystalline solid.: mp 166-167 °C; IR (KBr) 1659, 1617, 1601, 1558, 1506, 1407 cm-1; 1H-NMR (DMSO-d6) δ: 8.09 (1H, t, J = 7.9 Hz), 8.25 (1H, t, J = 7.9 Hz), 8.31 (1H, d, J = 7.9 Hz), 8.60 (1H, d, J = 7.9 Hz), 8.87 (1H, d, J = 3.7 Hz), 9.74 (1H, s), 12.18 (2H, s); 13C-NMR (DMSO-d6) δ: 119.7 (J = 3.3 Hz), 122.2 (J = 32.3 Hz), 127.8 (J = 14.9 Hz), 128.8 (J = 5.0 Hz), 129.8, 131.0, 135.6, 146.5 (J = 4.1 Hz), 155.8 (J = 256.6 Hz); FABMS m/z 148 (M+H–H2SO4)+; Anal. Calcd for C9H8FNO4S: C, 44.08; H, 3.29; F, 7.75; N, 5.71. Found: C, 43.90; H, 3.31; F, 7.81; N, 5.73.
4-Fluoroisoquinoline-5-sulfonyl chloride hydrochloride (5)
To liquid sulfur trioxide (47.7 kg, 596 mol) was gradually added 6a (15.8 kg, 64.4 mol) at 30 °C for 3 h and the resulting mixture was stirred at the same temperature for 16 h. To this reaction mixture was gradually added SOCl2 (42.2 kg, 355 mol) at the same temperature for 3 h. The mixture was heated with stirring to 70 °C for 4h and allowed to cool to 20 °C. The reaction mixture was slowly added to a mixture of water (208 kg), NaCl (48 kg), ice (200 kg) and CH2Cl2 (320 kg) at a temperature of less than 5 °C. The organic layer was partitioned. The aqueous layer was extracted with CH2Cl2 (3 x 126 kg). The combined organic layers were washed with saturated brine (85 kg), NaHCO3 (9 kg) in water (117 kg) and saturated brine (93 kg) and dried over anhydrous Na2SO4 (24 kg). Na2SO4 was filtered, washed with additional CH2Cl2 (210 kg) and combined. To this solution was slowly added 4 M HCl/EtOAc (18 kg) at 35 °C and the resulting mixture was stirred at 35 °C for 1 h. The precipitated crystals were filtered, washed with CH2Cl2 (63 kg) and dried under reduced pressure to yield 5 (7.04 kg, 39%) as a colorless crystalline solid. The crystalline solids contained a trace amount of the C8-isomer (9) which was determined by HPLC (Ratio of 5 and 9 was 99.64:0.36).: mp 200-201 °C (decomp); IR (KCl) 2993, 2303, 2086, 1654, 1495, 1375, 1184 cm-1; 1H-NMR (DMSO-d6) δ: 7.94 (1H, t, J = 7.8 Hz), 8.45-8.49 (1H, m), 8.67 (1H, dd, J = 7.8, 1.2 Hz), 8.77 (1H, d, J = 5.4 Hz), 9.62 (1H, s), 14.80 (1H, br s); 13C-NMR (DMSO-d6) δ: 124.2 (J = 13.4 Hz), 127.3 (J = 32.4 Hz), 129.2, 130.8, 130.9 (J = 3.8 Hz), 133.8, 142.4 (J = 5.7 Hz), 147.5 (J = 4.8 Hz), 155.0 (J = 268.0 Hz); 1H-NMR (CDCl3, as free base) δ: 7.84 (1H, t, J = 7.8 Hz), 8.40-8.44 (1H, m), 8.71 (1H, d, J = 4.4 Hz), 8.72 (1H, dd, J = 7.8, 1.5 Hz), 9.25 (1H, s); 13C-NMR (CDCl3, as free base) δ: 121.1 (J = 13.2 Hz), 126.6 (J = 1.7 Hz), 130.6, 134.5 (J = 28.1 Hz), 134.9, 135.7 (J = 1.7 Hz), 138.0 (J = 4.1 Hz), 149.2 (J = 5.8 Hz), 152.0 (J = 265.7 Hz); FABMS m/z 246 (M(as 35Cl)+H–HCl)+; Anal. Calcd for C9H6Cl2FNO2S: C, 38.32; H, 2.14; Cl, 25.13; F, 6.73; N, 4.96; S, 11.37. Found: C, 38.26; H, 2.09; Cl, 25.14; F, 6.58; N, 4.95; S, 11.34. Data for the intermediate (7): 1H-NMR (DMSO-d6) δ: 6.93 (1H, br s), 7.76 (1H, t, J = 7.8 Hz), 8.23-8.29 (1H, m), 8.49 (1H, dd, J = 7.8, 1.0 Hz), 8.52 (1H, d, J = 5.2 Hz), 9.28 (1H, s). Data for the intermediate (8): 1H-NMR (DMSO-d6) δ: 7.20 (1H, br s), 8.10 (1H, t, J = 7.8 Hz), 8.26 (2H, d, J = 7.8 Hz), 8.82 (1H, d, J = 3.4 Hz), 10.08 (1H, s).
(S)-4-Fluoro-N-(1-hydroxypropan-2-yl)isoquinoline-5-sulfonamide (4)
To a solution of (S)-2-aminopropanol (2.08 kg, 27.7 mol) in CH2Cl2 (92 kg) was added Et3N (6.30 kg, 62.3 mol) and the solution was cooled below –10 °C. To this mixture was gradually added 5 (7.04 kg, 25.0 mol) at –10 °C over 1 h. The resulting mixture was stirred at the same temperature for 30 min and allowed to stir at 20 °C for 18 h. After completion of the reaction, the mixture was diluted with additional CH2Cl2 (99 kg). The organic layer was washed with water (3 x 12 kg) and concentrated under reduced pressure. The residue was dissolved in IPA (36 kg) at 74 °C. The solution was allowed to cool to 25 °C and crystals were formed. The mixture was further cooled with stirring at 5 °C for 1 h. The precipitated crystals were filtered, washed with IPA (11 kg) and dried under reduced pressure at 40 °C to yield 4 (5.92 kg, 84%) as an off-white crystalline solid.: mp 149-151 °C; [α]19D –18.36 (c 0.54, CHCl3); IR (KBr) 3397, 3329, 3092, 1492, 1329, 1159, 1054 cm-1; 1H-NMR (CDCl3) δ: 1.06 (3H, d, J = 6.3 Hz), 1.78 (1H, t, J = 5.1 Hz), 3.43-3.59 (3H, m), 5.34 (1H, t, J = 7.1 Hz), 7.77 (1H, t, J = 7.8 Hz), 8.23-8.28 (1H, m), 8.60 (1H, d, J = 5.4 Hz), 8.68 (1H, dd, J = 7.8, 1.2 Hz), 9.19 (1H, s); 13C-NMR (CDCl3) δ: 18.0, 51.8, 66.0, 121.8 (J = 12.4 Hz), 127.1, 130.8 (J = 2.5 Hz), 132.9, 133.0 (J = 29.8 Hz), 134.1 (J = 4.1 Hz), 134.9, 149.6 (J = 4.1 Hz), 152.8 (J = 259.9 Hz); FABMS m/z 285 (M+H)+; Anal. Calcd for C12H13FN2O3S: C, 50.69; H, 4.61; F, 6.68; N, 9.85; S, 11.28. Found: C, 50.75; H, 4.49; F, 6.38; N, 9.93; S, 11.30.
(S)-4-Fluoro-N-[1-(3-hydroxypropylamino)propan-2-yl]isoquinoline-5-sulfonamide oxalate (3a)
To a solution of 4 (5.92 kg, 20.8 mol) in THF (65.1 kg) was added Et3N (3.16 kg, 31.2 mmol) at 20 °C. To the mixture was gradually added MsCl (2.50 kg, 21.8 mol) at –10 °C for 35 min. The reaction mixture was stirred at 0 °C for 30 min and then 3-aminopropanol (6.26 kg, 83.3 mol) was added. The reaction mixture was allowed to warm to 25 °C and stirred for 20 h. After completion of the reaction, the reaction mixture was concentrated under reduced pressure. The residue was diluted with CH2Cl2 (170 kg) and NaHCO3 (1.00 kg) in water (12.0 kg). The organic layer was partitioned and concentrated under reduced pressure. The residue was dissolved in IPA (107 kg) and water (7.10 kg). To this solution was gradually added oxalic acid dihydrate (2.62 kg, 20.8 mol) in the solution of IPA (26.4 kg) and water (1.80 kg) at 70 °C for 35 min. The reaction mixture was allowed to cool to 25 °C and crystals were formed. The precipitated crystals were filtered, washed with IPA (23.3 kg) and dried under reduced pressure at 40 °C to yield 3a (6.55 kg, 73%) as a colorless crystalline solid. The crystalline solids contained a trace amount of 3b which was determined by HPLC (0.3%).: mp 176-177 °C; [α]19D –42.29 (c 0.54, DMSO); IR (KBr) 3287, 3080, 1717, 1589, 1332, 1164 cm-1; 1H-NMR (DMSO-d6) δ: 0.89 (3H, d, J = 6.6 Hz), 1.66-1.77 (2H, m), 2.89-3.01 (4H, m), 3.46 (2H, t, J = 6.0 Hz), 3.59-3.69 (1H, m), 7.94 (1H, t, J = 7.8 Hz), 8.57 (2H, d, J = 7.8 Hz), 8.74 (1H, d, J = 4.9 Hz), 9.39 (1H, s); 13C-NMR (DMSO-d6) δ: 18.3, 28.5, 45.3, 46.7, 51.5, 58.2, 120.8 (J = 13.2 Hz), 127.6, 130.6 (J = 1.7 Hz), 132.5 (J = 28.1 Hz), 133.3, 134.1 (2C), 149.9 (J = 5.0 Hz), 152.1 (J = 263.2 Hz), 164.5 (2C); FABMS m/z 342 (M+H–(CO2H)2)+; Anal. Calcd for C17H22FN3O7S: C, 47.33; H, 5.14; F, 4.40; N, 9.74; S, 7.43. Found: C, 47.37; H, 4.99; F, 4.26; N, 9.84; S, 7.37.
(S)-4-Fluoro-5-(2-methyl-1,4-diazepan-1-ylsulfonyl)isoquinoline hydrochloride dihydrate (1)
To a solution of 3a (6.55 kg, 15.2 mol) in CH2Cl2 (85.2 kg) was added a solution of K2CO3 (5.00 kg, 36.2 mol) in water (11.1 kg) at 20 °C. The reaction mixture was stirred at the same temperature for 1 h. The organic layer was partitioned and the aqueous layer was extracted with CH2Cl2 (2 x 43.9 kg). The combined organic layers were concentrated under reduced pressure. The residue was dissolved in toluene (5.24 kg) and THF (5.90 kg). The solution was azeotropically evaporated to remove water under reduced pressure. The residue was dissolved in THF (93.7 kg). To this solution was added Ph3P (5.12 kg, 19.5 mol) and a solution of DIAD (95%, 4.10 kg, 19.3 mol) in toluene (5.90 kg) at 20 °C. The resulting mixture was stirred at 25 °C for 30 min and then concentrated under reduced pressure. The residue was dissolved in CH2Cl2 (41.9 kg). To this mixture was added 1.05 M HCl solution (34.7 kg) at 10 °C. The aqueous layer was partitioned, washed with CH2Cl2 (46.5 kg) and stirred with activated carbon (0.33 kg) at 25 °C for 1 h. The mixture was filtered to remove the activated carbon. The activated carbon was washed with water (10.0 kg). The combined aqueous layers were cooled to 10 °C and neutralized with 8.3 M sodium hydroxide solution (7.86 kg) until pH>8 and then extracted with CH2Cl2 (3 x 21.0 kg). The combined organic layers were washed with water (13.0 kg) and concentrated under reduced pressure. The residue was dissolved in acetone (31.2 kg). To this solution was gradually added concd HCl (1.52 kg, 15.0 mol) at 10 °C. The reaction mixture was stirred at 15 °C for 20 h and then cooled to 5 °C and stirred at the same temperature for 2h. The precipitated crystals were filtered, washed with acetone (8.80 kg) and dried under reduced pressure to yield 1 (4.41 kg, 73%, >99.9% ee) as a white crystalline solid.: mp 258-259 °C (decomp); [α]20D –8.82 (c 1.00, H2O); IR (KCl) 3406, 2983, 2763, 1588, 1324, 1146, 1129 cm-1; 1H-NMR (DMSO-d6) δ: 1.20 (3H, d, J = 6.6 Hz), 1.98-2.07 (2H, m), 3.06-3.16 (1H, m), 3.22-3.31 (2H, m), 3.35 (4H, s), 3.44 (1H, dd, J = 14.1, 4.4 Hz), 3.59-3.74 (2H, m), 4.37-4.47 (1H, m), 7.93 (1H, t, J = 7.8 Hz), 8.32 (1H, d, J = 7.8 Hz), 8.54-8.60 (1H, m), 8.72 (1H, d, J = 4.9 Hz), 9.39 (1H, s), 9.51 (2H, br s); 13C-NMR (DMSO-d6) δ: 16.6, 26.8, 42.9, 45.5, 50.3, 50.9, 120.9 (J = 12.4 Hz), 127.5, 130.7 (J = 1.7 Hz), 132.2, 132.5 (J = 27.3 Hz), 133.2 (J = 5.0 Hz), 133.3, 149.8 (J = 5.0 Hz), 152.0 (J = 264.0 Hz); FABMS m/z 324 (M+H–HCl–2H2O)+, Anal. Calcd for C15H23ClFN3O4S: C, 45.51; H, 5.86; Cl, 8.96; F, 4.80; N, 10.61. Found: C, 45.44; H, 5.65; Cl, 8.87; F, 4.68; N, 10.78.
ACKNOWLEDGEMENTS
We are grateful to Dr. H. Hidaka, CSO, of D. Western Therapeutics Institute for promotion study. We thank Dr. S. Tanabe, Director, for his encouragement and support and also Dr. K. Mizuno, Manager, for pharmacology suggestions at Tokyo New Drug Research Laboratories, Kowa Co., Ltd. We also wish to thank Dr. Kevin Swiss, Director, Kowa Research Institute, for his help in preparing the manuscript.
References
1. a) A similar pharmacological effect was obtained in the related compounds, see: K. Mizuno, T. Koide, Y. Fujieda, J. Mori, S. Kondo, J. Matsumoto, Y. Hattori, M. Nishio, and H. Hidaka, ARVO 2007; b) S. Tahara and H. Shimokawa, Yakugaku Zasshi, 2007, 127, 501. CrossRef
2. a) T. Asano, I. Ikegaki, S. Satoh, Y. Suzuki, M. Shibuya, M. Takayasu, and H. Hidaka, J. Pharmacol. Exp. Ther., 1987, 241, 1033; b) M. Shibuya and Y. Suzuki, BRAIN and NERVE, 1993, 45, 819; c) M. Shibuya, T. Asano, and Y. Sasaki, Acta. Neurochir. Suppl., 2001, 77, 201; d) E. Hu and D. Lee, Expert Opin. Ther. Targets, 2005, 9, 715; CrossRef e) R. Stavenger, Annu. Rep. Med. Chem., 2008, 43, 87. CrossRef
3. a) M. J. Huentelman, D. A. Stephan, J. Talboom, J. J. Corneveaux, D. M. Reiman, J. D. Gerber, C. A. Barnes, G. E. Alexander, E. M. Reiman, and H. A. Bimonte-Nelson, Behav. Neurosci., 2009, 123, 218; CrossRef b) Y. Fukumoto, M. Mohri, K. Inokuchi, A. Ito, Y. Hirakawa, A. Masumoto, Y. Hiraoka, A. Takeshita, and H. Shimokawa, J. Cardiovasc. Pharmacol., 2007, 49, 117; CrossRef c) H. Fujita, Y. Fukumoto, K. Saji, K. Sugimura, J. Demachi, J. Nawata, and H. Shimokawa, Heart, 2010, 25, 144.
4. A. Roe and C. E. Teague, Jr., J. Am. Chem. Soc., 1951, 73, 687. CrossRef
5. a) H. Hidaka, A. Matsuura, and T. Matsuzaki, World Patent WO97028130, 1997; b) H. Hidaka and A. Matsuura, World Patent WO99020620, 1999; c) H. Hidaka and H. Muramatsu, World Patent WO99054306, 1999; d) J. L. Peglion, A. Dessinges, C. Poitevin, J. P. Vilaine, N. Villeneuve, C. Thollon, and M. P. Bourguignon, European Patent EP1122254, 2001.
6. a) H. T. Clarke, G. S. Babcock, and T. F. Murray, Org. Synth., 1941, Coll. Vol. 1, 84; b) C. Gerard, L. C. McPhail, A. Marfat, N. P. Stimler-Gerard, D. A. Bass, and C. E. McCall, J. Clin. Invest., 1986, 77, 61; CrossRef c) A. Morikawa, T. Sone, and T. Asano, J. Med. Chem., 1989, 32, 42. CrossRef
7. a) M. S. Morgan and L. H. Cretcher, J. Am. Chem. Soc., 1948, 70, 375; CrossRef b) J. Y. Q. Lai, Y. Ferguson, and M. Jones, Synth. Commun., 2003, 33, 3427. CrossRef
8. M. Furukawa, M. Nishikawa, Y. Inaba, Y. Noguchi, T. Okawara, and T. Hitoshi, Chem. Pharm. Bull., 1981, 29, 623.
9. We investigated the other chlorosulfonylation conditions as follows. Attempts of the direct chlorosulfonylation of 6 with chlorosulfonic acid gave 5 as a minor product (13%) and the isomer (9) as the major product (24%) (by HPLC analysis). The separate use of the other strong acids such as ClSO3H, CF3SO3H, or Nafion NR-50 instead of concd H2SO4 decreased the regioselectivity compared to the combination of H2SO4/SO3 and SOCl2. The direct electrophilic reaction of SO2Cl2 in the separate presence of Lewis acids such as ClSO3H, AlCl3, or Nafion NR-50 was unsuccessful and gave complex mixtures.
10. a) I. J. Krauss and J. L. Leighton, Org. Lett., 2003, 5, 3201; CrossRef b) C. A. Olsen, C. Christensen, B. Nielsen, M. M. Farah, M. Witt, R. P. Clausen, J. L. Kristensen, H. Franzyk, and J. W. Jaroszewski, Org. Lett., 2006, 8, 3371; CrossRef c) F. Crestey, M. Witt, K. Frydenvang, D. Stærk, J. W. Jaroszewski, and H. Franzyk, J. Org. Chem., 2008, 73, 3566; CrossRef d) J. A. Groeper, J. B. Eaglesa, and S. R. Hitchcock, Tetrahedron:Asymmetry, 2009, 20, 1969; CrossRef e) As for the aziridine intermediate (11), we could not determine the accurate ratio of 10 and 11 by HPLC. Because the arylsulfony-activated aziridine (11) was very unstable due to the presence of isoquinoline moiety on molecule, compared with other aziridines.10c Data for the isolated 11: 1H-NMR (CDCl3) δ: 1.34 (3H, d, J = 5.4 Hz), 2.21 (1H, d, J = 4.4 Hz), 2.96 (1H, d, J = 6.8 Hz), 3.13-3.23 (1H, m), 7.77 (1H, t, J = 7.8 Hz), 8.29 (1H, d, J = 7.8 Hz), 8.61 (1H, d, J = 4.4 Hz), 8.67 (1H, d, J = 7.8 Hz), 9.18 (1H, s).
11. The isomer 3b was detected at various amounts in the following reaction conditions: 2.9% (at 0 °C and warmed to 25 °C for 12 h), 4.4% (at 20 °C for 12 h) and 5.4% (at 40 °C for 6 h). Although the complete suppression to produce 3b was too difficult, we finally succeeded in diminishing the yield of 3b from 2.9% to 0.3%. The methyl group at C1’ position of aziridine ring serves as steric hindrance to control the nucleophilic attack of 3-aminopropanol toward site A rather than site B.
12. J. R. Henry, L. R. Marcin, M. C. Mcintosh, P. M. Scola, G. D. Harris, Jr., and S. M. Weinreb, Tetrahedron Lett., 1989, 30, 5709. CrossRef