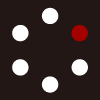
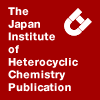
HETEROCYCLES
An International Journal for Reviews and Communications in Heterocyclic ChemistryWeb Edition ISSN: 1881-0942
Published online by The Japan Institute of Heterocyclic Chemistry
e-Journal
Full Text HTML
Received, 18th April, 2011, Accepted, 14th July, 2011, Published online, 21st July, 2011.
DOI: 10.3987/COM-11-12239
■ Synthetic Studies of Substituted Pyridine Aldehydes as Intermediates for the Synthesis of Toddaquinoline, Its Derivatives and Other Natural Products
Georgeta Serban,* Hitoshi Abe, and Yasuo Takeuchi
Pharmaceutical Chemistry Department, Faculty of Medicine and Pharmacy, University of Oradea, Nicolae Jiga 29, Oradea 410028, Romania
Abstract
The synthesis of substituted 2-bromopyridine aldehydes as intermediates in our planned approach to toddaquinoline, its derivatives and other natural products is reported. The DMF-formylation method of pyridine ring, the Vilsmeier-Haack procedure and the bromination of pyridine ring respectively, were studied in order to synthesize the above mentioned compounds. The successful methods (DMF-formylation and bromination of pyridine ring) provided the 2-bromo-4-formyl-5-tosyloxypyridine Ib and 5-benzyloxy-2,4,6-tribromo- nicotinaldehyde Ia under relatively mild conditions and in good yields.INTRODUCTION
Toddaquinoline, a benzo[h]quinoline alkaloid featuring a unique tetracyclic skeleton, was isolated in 1993 from the root bark of Toddalia asiatica, a constituent of many Asian folk medicines.1a,b In connection with our efforts to develop new and useful methods for the preparation of the Toddalia asiatica alkaloids2 and other natural products, our need for syntheses led us to prepare functionalized pyridine aldehydes type I, particularly molecules that bear bromine and hydroxyl functional groups.
RESULTS AND DISCUSSION
Taking into account the chemical literature concerning the pyridines being substituted with formyl or bromine group respectively, we focused on the possibilities of obtaining the substituted 2-bromopyridine aldehydes I and our synthetic approach is based on the four routes as shown in the retrosynthetic analysis (Scheme 1).
Pyridine ring formylation by DMF (route A)
Lithiation of aromatic and heteroaromatic compounds has been widely used to introduce various functional groups. This reaction is a very efficient functionalization method since electrophilic substitutions are often difficult on π-deficient heterocycles. 2-Bromopyridine aldehyde and its derivativesare valuable intermediates in heterocyclic chemistry. o-Bromopyridine aldehydes have been prepared by ortho-metalation of bromopyridines with lithium diisopropylamide LDA followed by electrophilic substitution with DMF.3a-c Based upon the fact that the ortho-metalation procedure studied previously in our laboratory2 is a useful route for the synthesis of various 2-bromopyridine aldehydes, our first strategy involved the pyridine ring formylation by DMF (route A). Subsequently the protection of hydroxyl group as benzyl,4 mesyl and tosyl,5 we started to investigate the reaction of 2-bromo-5-hydroxypyridine with LDA and DMF under various conditions.
Since in our previous studies the formylation of pyridine ring worked very well when 3 eq LDA/4 eq DMF/THF/-78 °C were used,2 in this case the first experiments using as starting materials 2-bromo-5-benzyloxypyridine 2 and 2-bromo-5-mesyloxypyridine 3 respectively, did not give the expected formylated products except the recovery of the starting materials in very high yields. Different experimental conditions still did not improve the result. Further we used 2-bromo-5-tosyloxypyridine 4 as starting material for the pyridine ring formylation by DMF (Scheme 2).
The formylation of 2-bromo-5-tosyloxypyridine 4 proved to be also troublesome in the above mentioned experimental conditions giving only the starting material in a very high yield (table 1, run 1). A higher reaction temperature (-80 °C→-40 °C) did not give a better result (run 2). By increasing the amount of deprotonation agent to 10 eq of LDA, the reaction proceeded in one case (run 3). When the reaction conditions of run 3 were applied again, the result could not be reproduced in the next experiments. Only when a different solvent (THF→ether) and higher amounts of deprotonation agent/formylation agent (10 eq LDA/4-8 eq DMF) were used, the formyl compound was obtained in a moderate to good yield (run 4-6).
The position of formyl group of the compound Ib was assigned by one- (1H-NMR, 13C-NMR) and two-dimensional experiments (HMBC), which showed the correlation of formyl hydrogen with C3, C4 and C5 of the pyridine ring meaning that deprotonation/formylation proceeded on the ortho-position of the functional group containing oxygen.6a-b
Pyridine ring formylation by Vilsmeier-Haack procedure (route B-C)
The Friedel-Crafts acylation and Vilsmeier formylation, which are important methods for the preparation of aryl ketones and aldehydes, fail in the pyridine series, making many 3-pyridine ketones and 3-pyridine carboxaldehydes difficult to prepare.7a In the last years, there have been developed synthesis of substituted pyridines via N-acyldihydropyridine or N-alkylpyridinium salts intermediates.7-e The 1,2- and 1,4-dihydropyridine have proven to be valuable intermediates for the preparation of interesting heterocyclic compounds. Meanwhile, 1,2-dihydropyridines that do not contain electron-withdrawing substituents on the ring are unstable, N-acyl-1,2-dihydropyridine are stabilized by the acyl group.8a,b The β-position of a 1-acyldihydropyridine is electron rich and susceptible to electrophilic attack. Shono9 described one example of β-formylation of 1-acyl-1,4-dihydropyridine and Comins reported the β-formylation of some substituted 1-(phenoxycarbonyl)-1,2-dihydropyridines via Vilsmeier-Haack reaction. The regiospecific β-formylation of 1-acyldihydropyridines has considerable potential for the synthesis of substituted 3-pyridine carboxaldehydes.7a,b
The preparation of compound Ia requires a method for the synthesis of N-acyl-6-bromo-3-hydroxy-1,2-dihydropyridine (route B). Comins synthesized the 3-substituted 1-(phenoxycarbonyl)-1,2-dihydropyridines using Fowler’s method by the reaction of several 3-substituted pyridines with phenyl chloroformate and sodium borohydride in methanol at -78 °C.7a Although Fowler and Comins have developed excellent procedures for the preparation of N-carboxyalkyl and N-carboxyphenyl 1,2-dihydropyridines, when these procedures were applied to 2-bromo-5-mesyloxypyridine 3 and 2-bromo-5-tosyloxypyridine 4 respectively, no reaction was found to occur and we recovered the unreacted starting material in every cases. The change of solvent (methanol→ether), higher amounts of reductive agent or acylation agent (concentration of sodium borohydride and phenyl chloroformate ranging from 1.3 eq and 1 eq, respectively to 2.5-3 eq) or varying the reaction temperature did not provide the desired N-acyl-6-bromo-3-hydroxy-1,2-dihydropyridine, the key intermediate for the formylation step (Scheme 3).
Further we studied the use of pyridinium salts as activated electrophilic substrates for reduction. The intermediate generated by the reduction of such salts can be reacted successfully with a range of different electrophiles (e.g. carbonyl compounds to give N-acyldihydropyridine).10 There are very few examples of 2-bromopyridine being N-alkylated using a suitable alkyl chain, the reluctance of 2-halopyridines to undergo alkylation with normal type of alkyl halides and tosylates being known.7d-e Thus, we decided to try the benzyl halides which have been used to synthesize N-benzylpyridinium salts in other examples11a,b and the N-alkylation was achieved in good yield when benzyl bromide was used (route C, Scheme 3). Having the 2-bromo-5-hydroxy-N-benzylpyridinium bromide 5 and 2-bromo-5-benzyloxy-N-benzylpyridinium bromide 6 in hands we focused on the reduction of the pyridinium salts. Earlier reports on the reduction of some pyridinium salts indicated that the selective formation of single dihydro isomer is difficult and dependent on temperature, pH and the nature of the reducing agent.12a,b Thus, the reduction of 2-bromo-5-hydroxy-N-benzylpyridinium bromide 5 by sodium borohydride, lithium aluminium hydride and sodium cyanoborohydride proved unsuccessful, in as much as many organic products very difficult to separate could be extracted from the reaction mixture.
With this background information, we carried out detailed studies on the reduction of 2-bromo-5-benzyloxy-N-benzylpyridinium bromide 6 by sodium dithionite in alkaline medium, sodium borohydride and tributyltin hydride. Working under various conditions, attempts to obtain 1,2- or 1,4-dihydropyridines were disappointing, the only compound obtained being the hydrolysis product 5-benzyloxy-N-benzylpyridine-2-one 7.
Selective sodium cyanoborohydride reduction in the nitrogen heterocyclic area and the conversion of certain pyridinium salts to the corresponding 1,2,5,6-tetrahydropyridines have been studied.13 The conversion of 1,2,5,6-tetrahydropyridines to dihydropyridines by bromination/double dehydrobromination with ethyl aluminium dichloride in HMPA under mild conditions is also known.11b Despite our efforts, the reduction of 6 by sodium cyanoborohydride in methanol at reflux or at room temperature gave the corresponding debrominated 1,2,5,6-tetrahydropyridine 8, not useful for bromination/double dehydrobromination step (Scheme 3).
Pyridine ring bromination (route D)
Our last approach for the preparation of compound Ia employed a bromination of the pyridine ring carrying the formyl group (route D, Scheme 4). The intermediate 5-benzyloxynicotinaldehyde 11, prepared from 3,5-dibromopyridine 9 by a method previously described,14a,b after acetalization with ethylene glycol in toluene15 yielded the corresponding 5-benzyloxynicotinaldehyde acetal 12. Since our experiments concerning the bromination of acetal 12 failed via electrophilic aromatic substitution (Br2/K2CO3, Br2/AcOH, benzyltrimethylammonium tribromide BTMABr3)16a-d and also via lithium-bromine exchange procedure (LDA/NBS, n-BuLi/NBS, n-BuLi/DBTCE dibromo tetrachloroethane, n-BuLi/LiDMAE/NBS, n-BuLi/LiDMAE/DBTCE),17a,b catalytic Pd/C hydrogenolysis of compound 12 in methanol18 gave debenzylated product 13 in a very good yield.
Out of the bromination reagents tried the best was benzyltrimethylammonium tribromide BTMABr3 which reacted with hydroxypyridine 13 in dichloromethane-methanol mixture. Various reaction conditions were experimented and good yields of the bromo compound 14 were obtained when a base (Et3N) was employed in order to neutralize a generating hydrogen bromide. Reaction of equimolar amounts of 3-hydroxypyridine 13 and bromine in the presence of a 10% aqueous solution of sodium hydroxide19a,b gave the brominated hydroxypyridine 14 only in low yield (15%). The results are summarized in the table 2.
In the next steps, the hydroxyl function was protected using the benzyl group4 which proved to be a good leaving group for deprotection method and the acetal group of 15 was removed by 10% HCl in THF20 to afford the 5-benzyloxy-2,4,6-tribromonicotinaldehyde Ia, a good intermediate for the synthesis of toddaquinoline and its derivatives.
In conclusion, we have developed highly practical and efficient syntheses of substituted 2-bromopyridine aldehydes Ia and Ib without using very expensive or dangerous reagents.
Due to reluctance of 2-bromopyridine for the introduction of substituents on its π-deficient aromatic ring, different synthetic pathways were studied: DMF-formylation method of pyridine ring, Vilsmeier-Haack formylation of N-acyl-1,2-dihydropyridine (starting from N-acyldihydropyridine or N-alkylpyridinium salts) and bromination of pyridine ring. The successful methods provided the 2-bromo-4-formyl-5-tosyloxypyridine Ib from 2-bromo-5-hydroxypyridine in 2 steps by DMF formylation method and 5-benzyloxy-2,4,6-tribromonicotinaldehyde Ia from 3,5-dibromopyridine in 7 steps by means of benzyltrimethylammonium tribromide under relatively mild conditions and in good yields. To the best of our knowledge, this is the first attempt of hydroxypyridines bromination using BTMABr3 as bromination reagent. The 2-bromopyridine aldehydes Ia and Ib are very good intermediates in the synthesis of toddaquinoline, its derivatives and other natural products.
EXPERIMENTAL
General: Melting points were measured on a micro-melting point hot-stage apparatus (Yanagimoto) and are uncorrected. The IR spectra were recorded using a JASCO FTIR-350 spectrophotometer. The 1H-NMR spectra in deuteriochloroform were recorded by a Mercury-300, VXR-500 or Varian Unity INOVA AS-600 spectrometer. The NMR spectral data are reported in parts per million downfield from the internal standard (tetramethylsilane, δ 0.0). The FAB-MS were obtained using a VG AutoSpec spectrometer with m-nitrobenzyl alcohol as the matrix. The elemental analysis was performed using a Yanaco MT-5 analyzer. Column chromatography was carried out with Merck silica gel (230-400 mesh). The TLC analysis was performed on Kieselgel 60 F254 (Merck) plates. All the experiments were carried out in an argon atmosphere, unless otherwise noted.
Substrates: 2-bromo-5-benzyloxypyridine 2,4 2-bromo-5-tosyloxypyridine 4, 2-bromo-4-formyl-5-tosyloxypyridine Ib,6b 3-bromo-5-benzyloxypyridine 10, and 3-formyl-5-benzyloxypyridine 1114a,b are known compounds.
2-Bromo-5-mesyloxypyridine (3). To a solution of 2-bromo-5-hydroxypyridine (1) (0.3 g, 1.72 mmol) in dry CH2Cl2 (20 mL), Et3N (1.19 mL, 8.62 mmol) was added. The mixture was cooled at 0 °C and MsCl (0.4 mL, 5.17 mmol) was added dropwise under stirring and then pyridine (0.15 mL, 1.72 mmol). The reaction mixture was stirred for 4 h at rt and then poured into ice water, neutralized with saturated aqueous solution of NaHCO3 and extracted with CH2Cl2. The solvent was removed under reduced pressure and the residue (0.52 g) was purified by column chromatography on silica gel. Elution with hexane-CH2Cl2 (2:1) gave the mesyloxypyridine 3 (0.433 g, 99%) as colourless prisms; mp 87.5-88.5 °C (Et2O). IR (KBr) cm-1: 1570, 1370. 1H-NMR (300 MHz, CDCl3) δ: 8.34 (dd, 1H, Py-H, J = 1.2; 2.1 Hz), 7.55 (d, 1H, Py-H, J = 1.2 Hz), 7.54 (d, 1H, Py-H, J = 2.1 Hz), 3.23 (s, 3H, CH3). LRMS (FAB, positive ion mode) m/z 252, 254 (M+H)+. Anal. Calcd for C6H6BrNO3S: C, 28.59; H, 2.40; N, 5.56. Found C, 28.52; H, 2.38; N, 5.47.
2-Bromo-4-formyl-5-tosyloxypyridine (Ib). To a LDA solution prepared from n-BuLi (hexane solution, 2 mL, 3.14 mmol) and i-Pr2NH (0.5 mL, 3.55 mmol) in Et2O (5 mL) at -80 °C for 1 h under Ar atmosphere, 2-bromo-5-tosyloxypyridine 4 (0.1 g, 0.304 mmol) solved in dry Et2O (8 mL) was added slowly and the mixture was stirred for 1.5-2 h at -80 °C. Dry DMF (0.2 mL, 2.57 mmol) was added drop by drop. After 1 h of stirring at -80 °C, the mixture was hydrolyzed with a 10% degassed aqueous solution of KH2PO4 (30 mL) and allowed to warm up to rt and extracted with Et2O. The combined organic layers were washed with brine, dried (Na2SO4), evaporated and purified by column chromatography on silica gel. Elution with hexane-Et2O (5:1) gave the formylated product Ib (0.057 g, 53%) as colorless prisms, mp 105-107 °C (hexane-Et2O). IR (KBr) cm-1: 1700, 1590, 1380, 1180. 1H-NMR (500 MHz, CDCl3) δ: 10.04 (s, 1H, CHO), 8.11 (s, 1H, Py-H6), 7.86 (s, 1H, Py-H3), 7.73 (d, 2H, Ar-H, J = 8 Hz), 7.39 (d, 2H, Ar-H, J = 8 Hz), 2.49 (s, 3H, CH3). 13C-NMR (150 MHz, CDCl3) δ: 184.98 (CHO), 147.25 (C, C-Ar), 146.53 (CH, C6-Py), 145.26 (C, C2-Py), 140.49 (C, C5-Py), 136.84 (C, C4-Py), 130.49 (CH, 2C-Ar), 130.26 (C, C-Ar), 128.62 (CH, 2C-Ar), 125.77 (CH, C3-Py), 21.82 (CH3). MS (FAB, positive ion mode) m/z 356, 358 (M+H)+. Anal. Calcd for C13H10BrNO4S: C, 43.84; H, 2.83; N, 3.93. Found: C, 44.19; H, 3.04; N, 3.79.
2-Bromo-5-hydroxy-N-benzylpyridinium bromide (5). To a solution of pyridine 1 (0.25 g, 1.43 mmol) in toluene (70 mL), BnBr (0.17 mL, 1.43 mmol) was added and the mixture was heated under reflux for 70 h. The solvent was removed under reduced pressure to furnish 2-bromo-5-hydroxy-N-benzylpyridinium bromide (5) (0.283 g, 56%) as white powder after washing with cold Et2O. mp 190-194 °C (EtOH). IR (KBr) cm-1: 3440, 3070, 1610, 1495, 1320. 1H-NMR (300 MHz, DMSO) δ: 12.18 (s, 1H, OH), 8.91 (d, 1H, Py-H, J = 2.8 Hz), 8.27 (d, 1H, Py-H, J = 9.1 Hz), 7.90 (dd, 1H, Py-H, J = 2.8; 9.1 Hz), 7.43-7.26 (m, 5H, Ph-H), 5.91 (s, 2H, CH2-Ph). LRMS (M-Br)+ 264, 266. Anal. Calcd for C12H11Br2NO: C, 41.77; H, 3.21; N, 4.06. Found: C, 42.03; H, 3.34; N 3.97.
2-Bromo-5-benzyloxy-N-benzylpyridinium bromide (6). To a solution of pyridine 2 (1 g, 3.78 mmol) in toluene (70 mL), BnBr (0.67 mL, 5.68 mmol) was added and the mixture was heated under reflux for 66 h. The solvent was removed and a liquid residue which crystallized in time was obtained. The crystals were filtered and washed with cold Et2O, giving the product 6 (0.982 g, 57%) as colourless cubic crystals. mp 142-144 °C (EtOH). IR (KBr) cm-1: 3060, 1615, 1510, 1260. 1H-NMR (300 MHz, DMSO) δ: 9.45 (d, 1H, Py-H, J = 3 Hz), 8.46 (d, 1H, Py-H, J = 9.3 Hz), 8.31 (dd, 1H, Py-H, J = 3; 9.3 Hz), 7.52-7.28 (m, 10H, Ph-H), 5.97 (s, 2H, N-CH2-Ph), 5.39 (s, 2H, O-CH2-Ph). LRMS (M-Br)+ 354, 356. Anal. Calcd for C19H17Br2NO: C, 52.44; H, 3.94; N, 3.22 Found C, 52.28; H, 4.01; N, 3.55.
3-Benzyloxy-N-benzyl-1,2,5,6-tetrahydropyridine (8). To a solution of N-benzylpyridinium bromide 6 (0.05g, 0.11 mmol) in dry MeOH (10 mL), NaBH3CN (0.014 g, 0.22 mmol) was added and the mixture was stirred for 22 h at rt. The mixture was poured into cold water, basified with saturated aqueous solution of NaHCO3 and extracted with AcOEt. The combined organic extracts were washed with brine, dried over anhydrous Na2SO4 and evaporated. The residue (0.05 g), purified by column chromatography on silica gel (hexane-AcOEt 15:1) gave the tetrahydropyridine 8 (0.021 g, 65%) as pale yellow oil. IR (NaCl) cm-1: 3050, 1680, 1500, 1450, 1220, 1180. 1H-NMR (300 MHz, CDCl3) δ: 7.39-7.28 (m, 10H, Ph-H), 4.79 (t, 1H, Py-H, J = 3.7 Hz), 4.72 (s, 2H, O-CH2-Ph), 3.66 (s, 2H, N-CH2-Ph), 3.03 (d, 2H, Py-H, J = 0.9 Hz), 2.60 (t, 2H, Py-H, J = 5.8 Hz ), 2.52-2.24 (m, 2H, Py-H). LRMS (FAB, positive ion mode) m/z 280 (M+H)+. HRMS (FAB) calcd for C19H22NO+: 280.1701, found 280.1708 (M+H)+.
5-Benzyloxypyridine-3-acetal (12). 3-Formyl-5-benzyloxypyridine (11) (0.27 g, 1.28 mmol) was dissolved in 25 mL of dry toluene. p-TsOH monohydrate (0.30 g, 1.6 mmol) was added and the mixture was stirred at 90 °C for 15 min. Ethylene glycol (0.35 mL, 6.29 mmol) was added and stirring was continued for 5 h at 110 °C. After the reaction was completed (TLC), the reaction mixture was cooled down, treated with saturated aqueous solution of NaHCO3 and extracted with CH2Cl2. The combined extracts were washed with brine, dried over anhydrous K2CO3 and evaporated. The yellow liquid residue (0.49 g) was purified by column chromatography on silica gel (hexane-AcOEt 2:1) to give the acetal 12 (0.297 g, 90%) as a colourless oil which crystallized in time as white prisms. mp 37-39 °C (crude). IR (KBr) cm-1: 2845, 1590, 1460, 1290, 970. 1H-NMR (300 MHz, CDCl3) δ: 8.38 (d, 1H, Py-H, J = 3 Hz), 8.32 (d, 1H, Py-H, J = 1.2 Hz), 7.45-7.32 (m, 6H, Ar-H, Py-H), 5.84 (s, 1H, CH-acetal), 5.11 (s, 2H, O-CH2-Ph), 4.13-4.01 (m, 4H, O-CH2-CH2-O). LRMS (FAB, positive ion mode) m/z 258 (M+H)+. HRMS (FAB) calcd for C15H16NO3+: 258.1130, found 258.1134 (M+H)+.
5-(1,3-Dioxolan-2-yl)pyridin-3-ol (13). A solution of pyridine 12 (0.61 g, 2.39 mmol) and 10% Pd on C (0.101 g) in MeOH (100 mL) was hydrogenated for 27 h at rt. The reaction mixture was filtered, washed through with MeOH and the filtrate was concentrated under reduced pressure to afford the compound 13 (0.393 g, 98%) as colourless prisms. mp 142-145 °C (CHCl3). IR (KBr) cm-1: 2845, 2810-2300, 1595, 1460, 1190, 970. 1H-NMR (300 MHz, CDCl3) δ: 8.23 (d, 1H, Py-H, J = 2.7 Hz), 8.21 (d, 1H, Py-H, J = 1.8 Hz), 7.39-7.38 (m, 1H, Py-H), 5.84 (s, 1H, CH-acetal), 4.13-4.08 (m, 2H, O-CH2-CH2-O), 4.06-4.01 (m, 2H, O-CH2-CH2-O). LRMS (FAB, positive ion mode) m/z 168 (M+H)+. Anal. Calcd for C8H9NO3: C, 57.48; H 5.43; N, 8.38; Found C, 57.14; H, 5.20; N, 8.27.
2,4,6-Tribromo-5-(1,3-dioxolan-2-yl)pyridin-3-ol (14). To a solution of pyridin-3-ol 13 (0.16 g, 0.99 mmol) in CH2Cl2-MeOH (20 mL-9 mL), dry Et3N (0.9 mL, 5.95 mmol) was added and the reaction mixture was stirred for a few minutes at -50 °C. After that BTMABr3 (1.54 g, 3.97 mmol) solved in 20 mL of CH2Cl2 was added dropwise. The temperature was increased from -50 °C to -35 °C during 3 h, after that 10% Na2S2O3 solution was added and the mixture was extracted with CH2Cl2. The combined organic extracts were washed with brine, dried (anhydrous K2CO3) and evaporated and the residue purified by column chromatography on silica gel (CH2Cl2-MeOH 50:1) gave the tribromopyridine 14 (0.273 g, 68%) as a white powder. mp 192.5-193.5 °C (CHCl3). IR (KBr) cm-1: 3400-3200, 2845, 1540, 1430, 1160, 960. 1H-NMR (300 MHz, CDCl3) δ: 6.25 (s, 1H, CH-acetal), 4.35-4.27 (m, 2H, O-CH2-CH2-O), 4.16-4.08 (m, 2H, O-CH2-CH2-O). 13C-NMR (125 MHz, CDCl3) δ: 144.64 (C), 128.58 (C), 127.21 (C), 125.72 (C), 119.04 (C), 100.45 (CH), 62.96 (2C, CH2). LRMS (FAB, positive ion mode) m/z 402, 404, 406, 408 (M+H)+. Anal. Calcd for C8H6Br3NO3: C, 23.79; H, 1.50; N, 3.47; Found C, 23.75; H, 1.44; N, 3.32.
3-Benzyloxy-2,4,6-tribromo-5-(1,3-dioxolan-2-yl)pyridine (15). To a solution of tribromopyridine 14 (0.68 g, 1.7 mmol) in dry DMF (70 mL) at 0 °C, anhydrous K2CO3 (0.47 g, 3.4 mmol) and a catalytic amount of KI (0.1 g) were added. The reaction mixture was stirred for 30 min at 0 °C and for another 30 min at rt, then was treated with BnBr (0.58 g, 3.4 mmol) for 6 h at rt. When the reaction was completed, cold water was added and the reaction mixture was extracted with CH2Cl2 and dried over anhydrous K2CO3. The solvent was removed under reduced pressure and the red liquid residue (1.52 g) was purified by column chromatography on silica gel (hexane-AcOEt 10:1) to give the benzyloxypyridine 15 (0.701 g, 84%) as colourless oil. IR (KBr) cm-1: 2860, 1520, 1420, 1220, 1030, 965. 1H-NMR (300 MHz, CDCl3) δ: 7.59-7.53 (m, 2H, Ar-H), 7.46-7.38 (m, 3H, Ar-H), 6.30 (s, 1H, CH-acetal), 5.05 (s, 2H, CH2Ph), 4.37-4.32 (m, 2H, O-CH2-CH2-O), 4.12-4.08 (m, 2H, O-CH2-CH2-O). LRMS (FAB, positive ion mode) m/z 492, 494, 496, 498 (M+H)+. HRMS (FAB) calcd for C15H13Br3NO3+: 495.8352, found 495.8385 (M+H)+.
5-Benzyloxy-2,4,6-tribromo-3-formylpyridine (Ia). To a solution of acetal 15 (0.74 g, 1.49 mmol) in THF (70 mL) was added 10% HCl (50 mL) and the reaction mixture was stirred for 3.5 h at 65 °C. After the reaction was completed (TLC), saturated aqueous solution of NaHCO3 was added and the mixture was extracted with AcOEt. The combined organic extracts were washed with brine, dried over anhydrous K2CO3 and evaporated under reduced pressure. The solid residue was purified by column chromatography on silica gel (hexane-AcOEt 20:1) to give the formylpyridine Ia (0.58 g, 86%) as colourless needles. mp 122.5-125 °C (Et2O-hexane). IR (KBr) cm-1: 1700, 1525, 1500, 1220, 1030. 1H-NMR (300 MHz, CDCl3) δ: 10.17 (s, 1H, CHO), 7.58-7.52 (m, 2H, Ar-H), 7.44-7.41 (m, 3H, Ar-H), 5.10 (s, 2H, CH2Ph). LRMS (FAB, positive ion mode) m/z 448, 450, 452, 454 (M+H)+. Anal. Calcd for C13H8Br3NO2: C, 34.70; H 1.79; N, 3.11. Found C, 34.93; H, 1.81; N, 3.07.
ACKNOWLEDGEMENTS
The authors are grateful to SC NMR Laboratory of Okayama University for the use of the facilities. We also wish to thank the Japan Society for the Promotion of Science (JSPS) for a postdoctoral fellowship for G.S. and financial support. Many thanks to Prof. I. Grosu, UBB Cluj-Napoca Romania, for his helpful suggestions and comments during the preparation of this paper.
References
1. (a) I. S. Chen, I. L. Tsai, S. J. Wu, W. S. Sheen, T. Ishikawa, and H. Ishii, Phytochemistry, 1993, 34, 1449; CrossRef (b) W. S. Kan, “Manual of Medicinal Plants in Taiwan”, vol. 2, National Research Institute of Chinese Medicine, Taiwan, 1970, p. 382.
2. G. Serban, Y. Shigeta, H. Nishioka, H. Abe, Y. Takeuchi, and T. Harayama, Heterocycles, 2007, 71, 1623. CrossRef
3. (a) P. Melnyk, J. Gasche, and C. Thal, Synth. Commun., 1993, 23, 2727; CrossRef (b) A. Numata, Y. Kondo, and T. Sakamoto, Synthesis, 1999, 306; CrossRef (c) F. Bracher, J. Heterocycl. Chem., 1993, 30, 157. CrossRef
4. E. Kuwano, L. Hassan, and Y. Sasama, JP Patent 10101647 A2, 1998.
5. P. Storck, A. M. Aubertin, and D. S. Grierson, Tetrahedron Lett., 2005, 46, 2919. CrossRef
6. (a) J. Clayden, N. Greeves, S. Warren, and P. Wothers, “Organic Chemistry”, Oxford University Press, Oxford, UK, 2001, pp. 214-215; (b) G. Serban, H. Abe, Y. Takeuchi, and T. Harayama, Heterocycles, 2008, 75, 2949. CrossRef
7. (a) D. L. Comins and J. J. Herrick, Heterocycles, 1987, 26, 2159; CrossRef (b) D. L. Comins and N. B. Mantlo, J. Org. Chem., 1986, 51, 5456; CrossRef (c) D. L. Comins and A. L. Williams, Org. Lett., 2001, 3, 3217; CrossRef (d) A. P. Dobbs, K. Jones, and K. T. Veal, Tetrahedron Lett., 1997, 38, 5383; CrossRef (e) M. L. Bennasar, C. Juan, and J. Bosch, Chem. Commun., 2000, 2459. CrossRef
8. (a) F. W. Fowler, J. Org. Chem., 1972, 37, 1321; CrossRef (b) M. J. Wyle and F. W. Fowler, J. Org. Chem., 1984, 49, 4025. CrossRef
9. T. Shono, Y. Matsumura, K. Tsubata, Y. Sugihara, S. Yamane, T. Kanazawa, and T. Aoki, J. Am. Chem. Soc., 1982, 104, 6697. CrossRef
10. T. J. Donohoe, D. J. Johnson, L. H. Mace, M. J. Bamford, and O. Ichihara, Org. Lett., 2005, 7, 435. CrossRef
11. (a) Y. Takeuchi, M. Hattori, H. Abe, and T. Harayama, Synthesis, 1999, 10, 1814; CrossRef (b) S. Raucher and R. F. Lawrence, Tetrahedron, 1983, 39, 3731. CrossRef
12. (a) G. Paglietti, P. Sanna, A. Nuvole, F. Soccolini, and R. M. Acheson, J. Chem. Res., 1983, 245; J. Chem. Res., 1983, 2326; (b) P. Sanna, A. Carta, and G. Paglietti, J. Chem. Res., 1992, 14; J. Chem. Res., 1992, 301.
13. R. O. Hutchins and N. R. Natale, Synthesis, 1979, 281. CrossRef
14. (a) D. C. Harrowven, M. I. T. Nunn, N. J. Blumire, and D. R. Fenwick, Tetrahedron Lett., 2000, 41, 6681; CrossRef (b) D. C. Harrowven, M. I. T. Nunn, N. J. Blumire, and D. R. Fenwick, Tetrahedron, 2001, 57, 4447. CrossRef
15. M. Lounasmaa, T. Tamminen, and R. Jokela, Heterocycles, 1985, 23, 1735. CrossRef
16. (a) J. Alcazar, J. M. Alonso, J. M. Bartolome, L. Iturrino, and E. Matesanz, Tetrahedron Lett., 2003, 44, 8983; CrossRef (b) T. M. Bargar, T. Wilson, and J. K. Daniel, J. Heterocycl. Chem., 1985, 22, 1583; CrossRef (c) S. Kajigaeshi, T. Kakinami, H. Tokiyama, T. Hirakawa, and T. Okamoto, Chem. Lett., 1987, 627; CrossRef (d) K. Umemura, K. Watanabe, K. Ono, M. Yamaura, and J. Yoshimura, Tetrahedron Lett., 1997, 38, 4811. CrossRef
17. (a) F. C. Fevrier, E. D. Smith, and D. L. Comins, Org. Lett., 2005, 7, 5457; CrossRef (b) B. Abarca, R. Ballesteros, G. Jones, and F. Mojarrad, Tetrahedron Lett., 1986, 27, 3543. CrossRef
18. D. R. Fenwick, A. T. Gillmore, and M. Y. Platts, Patent PCT Int. Appl., PIXXD2 WO 2006085199 A1, 2006.
19. (a) G. J. Clark and L. W. Deady, Aust. J. Chem., 1981, 34, 927; CrossRef (b) V. Koch and S. Schnatterer, Synthesis, 1990, 497. CrossRef
20. S. P. Chavan and R. Sivappa, Tetrahedron, 2004, 60, 9931. CrossRef