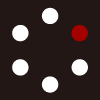
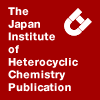
HETEROCYCLES
An International Journal for Reviews and Communications in Heterocyclic ChemistryWeb Edition ISSN: 1881-0942
Published online by The Japan Institute of Heterocyclic Chemistry
e-Journal
Full Text HTML
Received, 10th May, 2011, Accepted, 27th June, 2011, Published online, 8th July, 2011.
DOI: 10.3987/COM-11-S(P)20
■ Ni- and Cu-Catalyzed Coupling Reactions Using 2-(4,5-Dihydro-1H-imidazo-2-yl)phenol as a Versatile Phosphine-Free Ligand
Satoshi Haneda, Kazuhiko Sudo, and Masahiko Hayashi*
Department of Chemistry , Graduate School of Science, Kobe University, 1-1 Rokkodai-cho, Nada-ku, Kobe 657-8501, Japan
Abstract
We have disclosed 2-(4,5-dihydro-1H-imidazo-2-yl)phenol (1) worked efficiently as a ligand in Ni-catalyzed Suzuki-Miyaura coupling reactions and Cu-catalyzed N-cyclopropylation of indoles using cyclopropylboronic acid.INTRODUCTION
In 1995, Percec and his co-workers first reported Ni-catalyzed Suzuki-Miyaura coupling of aryl mesylates with arylboronic acid using 10 mol% of NiCl2(dppf) (dppf = 1,1’-bis(diphenylphosphino)ferrocene) in the presence of K3PO4 in dioxane at 100 °C. They used Zn to generate Ni(0) in situ from NiCl2(dppf).1 In 1996, Miyaura et al. reported the use of 4 eq. of n-BuLi instead of Zn as a reducing reagent (eq. 1).2
Indolese revealed the Suzuki-Miyaura coupling of aryl chlorides with arylboronic acids proceeded using NiCl2(dppf) in the presence of K3PO4 in dioxane without any reducing reagents in 1997.3 Miyaura also, reported the system of NiCl2(PPh3)2/2PPh3/K3PO4.n-H2O/toluene in Suzuki—Miyaura coupling.4-6
As for phosphine-free ligand, Leadbeater first reported nitrogen based Ni-catalyzed coupling reactions using NiCl2(NEt)3 and NiCl2(bpy) complexes as a catalyst.7 We also reported nitrogen-based, especially, including imidazole or imidazoline ligand—PdCl2 catalyzed Mizoroki-Heck and Suzuki-Miyaura coupling reactions.8
Here we report the utility of 2-(4,5-dihydro-1H-imidazo-2-yl)phenol 1 as a useful ligand in Ni- and Cu-catalyzed coupling reaction using boronic acid as a coupling partner.
RESULTS AND DISCUSSION
We first examined the reaction of Ni-catalyzed reaction of 4-methylbromobenzene with phenylboronic acid. The nature of the ligand is summarized in Table 1. Only ligand 1 exhibited the high performance to give the coupling product in high yield (72% and 92% by the use of NiCl2 and Ni(cod)2 as a catalyst precursor, respectively). In Mizoroki-Heck reaction, other ligand or catalyst, for example, ligand 5—PdCl2 complex showed high catalytic activity,8a however, in the present Ni-catalyzed reaction, catalytic activity of ligand 5—PdCl2 complex was proved to be quite low. After screening of the metal source, ligand, solvent, base, and reaction temperature, we optimized the reaction condition as follows: 3 mol% of NiCl2 in the presence of 3 mol% of ligand, in the presence of 2 eq. of K3PO4 as a base in dioxane at 95 °C for 24 h. The reaction of 2-bromonaphthalene with phenylboronic acid gave the coupling product in 49% yield (eq. 2). In this case, Ni(OAc)2•4H2O exhibited higher reactivity than NiCl2 and Ni(cod)2 as a Ni source.
Then we examined Cu-catalyzed N-cyclopropylation of indoles using ligand 1. The direct introduction of cyclopropyl group is one of the important problems for the synthesis pharmacuitical compounds such as Nevirapine, Ciprofloxacin and Sparfloxacin. To the best of our knowledge, there are two reports of N-cyclopropylation of indoles. In 2007, Gagnon and his co-workers reported the N-cyclopropylation of indoles using tricyclopropylbismus reagents promoted by 1.5 eq. Cu(OAc)2 and 3 eq. pyridine to give the cyclopropylation products in 31—98% yield.9 Tsuritani and his co-workers reported the use of cyclopropylboronic acid in the presence of 0.1 eq. or 1 eq. of Cu(OAc)2, 3 eq. DMAP, 1 eq. NaHMDS. In both of catalytic and stoichiometric cases, the yields range from low to good or high, that is, <5—72% yield (0.1 eq.) and 15—93% yield (1 eq.), respectively.10
Based on the above reported background, we examined the utility of 2-(4,5-dihydro-1H-imidazo- 2-yl)phenol 1 as a ligand in the reaction of substituted indoles and cyclopropylboronic acid catalyzed by Cu(OAc)2. After screening of a variety of reaction factors such as Cu prepursors, solvent, base and temperature, we found the combination of Cu(OAc)2, ligand 1, toluene as a solvent, pyridine as a base 95 °C was the best choice. By using this system, cycloproplated product of indole was obtained in 41% yield. It should be mentioned that the ligand 1 also gave the best result in this reaction among the ligands we examined such as bipyridyl, and 9,10-phenanthroline. Then, we examined the reaction of substituted indoles with cyclopropylboronic acid. The indole having nitro group at 5 and 6 position gave the products in 62% and 63% yield, respectively.
As for the reaction mechanism, Tromp and his co-workers examined the reaction of Cu(II)-catalyzed arylation of imidazoles and phenylboronic acid using multiple and time-resolved spectroscopic techniques such as EDXFAFS, UV-vis, EPR, and NMR.11 Based on their proposed mechanism, we adopted disproportionation mechanism as shown in Scheme 1. Cu-alkoxide will form the dimeric species of Cu(II), which disproportionated Cu(I) and Cu(III), then, the latter will catalyze the cyclopropanation of indole with cyclopropylboronic acid. The resulting Cu(I) will be oxidized to Cu(III) by O2.
Phthalimide is more reactive than indole. Under the reaction condition, that is, 10 mol% of Cu(OAc)2 and 10 mol% of ligand 1 in the presence of 3 equiv. of pyridine in toluene afforded the cyclopropyl product in 83% yield (eq. 3).
CONCLUSION
We have revealed that 2-(4,5-dihydro-1H-imidazo-2-yl)phenol worked efficiently in Ni-catalyzed Suzuki-Miyaura reaction and Cu-catalyzed N-cyclopropylation of indoles using cyclopropylboronic acid.
EXPERIMENTAL
General: IR spectra were measured on a PERKIN ELMER FT-IR Spectrometer SPECTRUM 1000. 1H and 13C NMR spectra (400.0 and 100.6 MHz, respectively) were recorded on a JEOL JNM-LA 400 using Me4Si as the internal standard in CDCl3. Elemental analyses were performed with a Yanaco CHN Corder MT-5. Mass spectra were measured on a Thermo Quest LCQ DECA XPPlus. Preparative column chromatography was carried out on Fuji Silysia BW-820MH or YMC∗GEL Silica (6nm I-40-63 um). Filtrations were carried out using nacalai tesque Celite®500 (grain size 1.5 µm) or nacalai tesque Hyflo Super-Cel® (grain size 7 µm). Thin-layer chromatography (TLC) was carried out on Merck 25 TLC aluminum Sheets silica gel 60 F254 (layer thickness 0.2 mm). As for Ni-catalyzed coupling product, the spectra of these compounds were consistent with the authentic sample of commercially available 4-phenyltoluene and 2-phenylnaphthalene.
1-Cyclopropylindole10
Rf = 0.80 (hexane:EtOAc =3:1). Pale yellow oil. IR (neat): νmax (cm-1); 1511, 1464, 1316, 1024, 742, 420. 1H NMR (400 MHz, CDCl3): δ 7.62 (d, J = 8.0 Hz, 1H), 7.60 (d, J = 8.0 Hz, 1H), 7.20 (t, J = 8.0 Hz, 1H), 7.12—7.09 (m, 2H), 6.41 (d, 1H, J = 3.2 Hz), 3.3—3.4 (m, 1H), 1.2—1.0 (m, 4H). 13C NMR (100.6 MHz, CDCl3) δ 137.14, 128.69, 127.96, 121.44, 120.81, 119.66, 110.25, 101.01, 26.86, 6.13.
1-Cyclopropyl-5-nitroindole10
Rf = 0.45 (hexane: EtOAc =3:1). Yellow oil. IR (KBr): νmax (cm-1); 3040, 1514, 1476, 1330, 1278, 1060, 1033, 888, 814, 745, 710, 422. 1H NMR (400 MHz, CDCl3): δ 8.56 (d, J = 2.4 Hz, 1H), 8.13 (dd, J = 2.4 Hz, 8.8 Hz, 1H), 7.58 (d, J = 8.8 Hz, 1H), 7.29 (d, J = 3.4 Hz, 1H), 6.35 (d, J = 3.4 Hz, 1H), 3.3—3.2 (m, 1H), 1.1—1.0 (m, 4H). 13C NMR (100.6 MHz, CDCl3) δ 131.26, 118.09, 117.28, 110.12, 103.75, 27.36, 6.42.
1-Cyclopropyl-6-nitroindole
Rf = 0.63 (hexane: EtOAc =3:1). Yellow oil. IR (KBr): νmax (cm-1); 1587, 1511, 1493, 1467, 1326, 1283, 1055, 1027, 869, 824, 764, 731. 1H NMR (400 MHz, CDCl3): δ 8.50 (s, 1H), 8.01 (d, J = 7.2 Hz, 1H), 7.40 (d, J = 8.8 Hz, 1H), 6.52 (d, J = 3.2 Hz, 1H), 3.5—3.4 (m, 1H), 1.2—1.0 (m, 4H). 13C NMR (100.6 MHz, CDCl3) δ 133.80, 120.72, 115.33, 107.33, 102.13, 27.27, 6.38. Anal. Calcd for C11H10N2O2: C, 65.34,; H, 4.98; N, 13.85. Found: C, 65.03; H, 5.08; N, 13.48.
1-Cyclopropyl-5-bromoindole12
Rf = 0.76 (hexane: EtOAc =3:1). Organge oil. IR (neat): νmax (cm-1); 3092, 3011, 2923, 1506, 1467, 1382, 1277, 1231, 1027, 795. 1H NMR (400 MHz, CDCl3): δ 8.52 (d, J = 2.0 Hz, 1H), 8.10 (m, 1H), 7.55 (d, J = 8.8 Hz, 1H), 7.27 (dd, J = 2.0 Hz, 8.8 Hz, 1H), 6.59 (d, J = 3.6 Hz, 1H), 3.4—3.3 (m, 1H), 1.1—1.2 (m, 4H). 13C NMR (100.6 MHz, CDCl3) δ 136.05, 130.28, 129.09, 124.22, 123.16, 112.94, 111.63, 100.56, 26.91, 6.12.
1-Cyclopropyl-5-chloroindole10
Rf = 0.75 (hexane: EtOAc =3:1). Pale yellow liquid. IR (neat): νmax (cm-1); 3011, 1464, 1385, 1277, 1231, 1062, 1026, 907, 870, 796, 780, 755, 720, 438. 1H NMR (400 MHz, CDCl3): δ 7.55 (d, J = 2.0 Hz, 1H), 7.45 (d, J = 8.4 Hz, 1H), 7.2—7.1 (m, 2H), 6.35 (d, J = 3.2 Hz, 1H), 3.3—3.2 (m, 1H), 1.1—1.0 (m, 4H). 13C NMR (100.6 MHz, CDCl3) δ 135.82, 129.62, 129.25, 125.36, 121.71, 120.12, 111.64, 100.66.
1-Cyclopropyl-5-methylindole10
Rf = 0.83 (hexane: EtOAc =3:1). Pale yellow liquid. IR (neat): νmax (cm-1); 3013, 1713, 1486, 1386, 1293, 1240, 1227, 1025, 871, 793, 761, 760. 1H NMR (400 MHz, CDCl3): δ 7.5—7.4 (m, 2H), 7.1—7.0 (m, 2H), 6.33 (d, J = 4.0 Hz, 1H), 3.3—3.2 (m, 1H), 2.45 (s, 3H), 1.1—1.0 (m, 4H). 13C NMR (100.6 MHz, CDCl3) δ 135.84, 128.87, 128.00, 123.06, 120.50, 109.92, 100.47, 26.88, 21.36, 6.11.
N-Cyclopropylphthalimide12
Rf = 0.51 (hexane: EtOAc =3:1). White solid (mp 135—136 °C, lit.12 136 °C). IR (KBr): νmax (cm-1); 3025, 1765, 1713, 1700, 1610, 1401, 1140, 946, 720. 1H NMR (400 MHz, CDCl3): δ 7.83 (m, 2H), 7.71 (m, 2H), 2.72 (m, 1H), 1.1—1.0 (m, 4H). 13C NMR (100.6 MHz, CDCl3) δ 133.99, 131.81, 123.14, 20.94, 5.20.
References
1. V. Perec, J.-Y. Bae, and D. H. Hill, J. Org. Chem., 1995, 60, 1060. CrossRef
2. S. Saito, M. Sakai, and N. Miyaura, Tetrahedron Lett., 1996, 37, 2993. CrossRef
3. A. F. Indolese, Tetrahedron Lett., 1997, 38, 3513. CrossRef
4. S. Saito, S. Ohtani, and N. Miyaura, J. Org. Chem., 1997, 62, 8024. CrossRef
5. K. Inada and N. Miyaura, Tetrahedron, 2000, 56, 8657. CrossRef
6. For other phosphine ligand Ni-catalyzed Suzuki-Miyaura type reaction: (a) J.-C. Galland, M. Savignac, and J.-P. Genêt, Tetrahedron Lett., 1999, 40, 2323; CrossRef (b) K. Inada and N. Miyaura, Tetrahedron, 2000, 56, 8657; CrossRef (c) B. H. Lipshutz, J. A. Sclafani, and P. A. Blomgren, Tetrahedron, 2000, 56, 2139; CrossRef (d) V. Percec, G. M. Golding, J. Smidrkal, and O. Weichold, J. Org. Chem,. 2004, 69, 3447; CrossRef (e) Z.-Y. Tang and Q.-S. Hu, J. Am. Chem. Soc., 2004, 126, 3058; CrossRef (f) J.-R. Zhou and G. C. Fu, J. Am. Chem. Soc., 2004, 126, 1340; CrossRef (g) Z.-Y. Tang, S. Spinella, and Q.-S. Hu. Tetrahedron Lett., 2006, 47, 2427; CrossRef (h) Z.-Y.Tang and Q.-S. Hu, J. Org. Chem., 2006, 71, 2167; CrossRef (i) C.-C. Lee, W.-C. Ke, K.-T. Chan, C.-L. Lai, C.-H. Hu, and H. M. Lee, Chem. Eur. J., 2007, 13, 582; CrossRef (j) C. Chen and L.-M. Yang, Tetrahedron Lett., 2007, 48, 2427; CrossRef (k) L. Zhou, Q. Miao, R. He, X. Feng, and M. Bao, Tetrahedron Lett., 2007, 48, 7899. CrossRef
7. N. E. Leadbeater and S. M. Resouly, Tetrahedron, 1999, 55, 11889. CrossRef
8. (a) S. Haneda, Z. Gan, K. Eda, and M. Hayashi, Organometallics, 2007, 26, 6551; CrossRef (b) S. Haneda, C. Ueba, K. Eda, and M. Hayashi, Adv. Synth. Catal., 2007, 349, 833; CrossRef (c) K. Kawamura, S. Haneda, A. Gan, K. Eda, and M. Hayashi, Organometallics, 2008, 27, 3748. CrossRef
9. A. Gagnon, M. St-Onge, K. Little, M. Duplessis, and F. Barabé, J. Am. Chem. Soc., 2007, 129, 44. CrossRef
10. T. Tsuritani, N. A. Strotman, Y. Yamamoto, M. Kawasaki, N. Yasuda, and T. Mase, Org. Lett., 2008, 10, 1653. CrossRef
11. M. Tromp, G. P. F. Strijdonck, S. S. Berkel, A. Hoogenband, M. C. Feiters, B. Bruin, S. G. Fiddy, A. J. Eerden, J. A. Bokhoven, P. W. N. M. Leeuwen, and D. C. Koningsberger, Organometallics, 2010, 29, 3085. CrossRef
12. S. Bénard, L. Neuvile, and J. Zhu, J. Org. Chem., 2008, 73, 6441. CrossRef