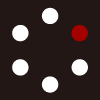
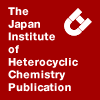
HETEROCYCLES
An International Journal for Reviews and Communications in Heterocyclic ChemistryWeb Edition ISSN: 1881-0942
Published online by The Japan Institute of Heterocyclic Chemistry
e-Journal
Full Text HTML
Received, 12th May, 2011, Accepted, 29th August, 2011, Published online, 5th September, 2011.
DOI: 10.3987/COM-11-12258
■ A Molecular Orbital Calculation Study on Ring-Opening Reactions of Fluorine-Containing 3,4-Dihydro-2H-pyrans with Amines and Thiols
Norio Ota, Yasuhiro Kamitori,* Yusuke Tamai, Guo Feng, Mizuki Hatakenaka, and Etsuji Okada*
Department of Chemical Science and Engineering, Graduate School of Engineering, Kobe University, 1-1 Rokkodai-cho, Nada-ku, Kobe 657-8501, Japan
Abstract
The reactions of 5-trifluoroacetyl-6-trifluoromethyl-3,4-dihydro-2H-pyran (1a) with various amines and sodium thiolates gave the corresponding 4,4-bistrifluoroacetyl-1,3-butadienyl amines (2b) and sulfides (2c), respectively, in good yields. Our DFT calculations suggested that the elimination of isobutanol from 1a and the subsequent electrocyclic ring-opening reaction on intermediate (4a) would proceed to afford 4,4-bistrifluoroacetyl-1,3-butadienyl ether (2a). Moreover, subsequent nucleophilic O-N and O-S exchange reactions on 2a which gave 2b and 2c, respectively, were also predicted.INTRODUCTION
It is well-known that various kinds of fluorine-containing heterocycles are assembled into new chemical entities given that these compounds are widely recognized as important organic materials that exhibit bioactive functions in modern drug discovery and development.1-4 In the course of our continuous researches concerning syntheses and reactions of novel fluorine-containing heterocycles, we found that 2-ethoxy-4-isobutoxy-6-trifluoromethyl-3,4-dihydro-2H-pyran (1a) which could be easily obtained by hetero Diels-Alder reaction of 1,1,1,5,5,5-hexafluoro-3-(isobutoxyallylidene)pentane-2,4-dione with ethyl vinyl ether,5 reacted with various amines and sodium thiolates under very mild conditions to give the corresponding 4,4-bistrifluoroacetyl-1,3-butadienyl amines (2b)6 and sulfides (2c),7 respectively, in good yields (Scheme 1). Both dienes, 2b and 2c, are versatile building blocks to access a variety of novel fluorine-containing heterocycles. Hence, these ring-opening reactions depicted in Scheme 1 are assumed to be the key steps to construct a wide range of novel fluorine-containing heterocyclic systems.
Recently, we reported a similar ring-opening reaction of 1a with aromatic compounds in refluxing trifluoroacetic acid affording 4,4-bis(trifluoroacetyl)-1,3-butadienylated aromatic compounds (2d) selectively.8 Formation of 2d was reasonably explained by the mechanism including pyrylium (3) as a key intermediate which was afforded by the protonation on alkoxy oxygens of 1a under strongly acidic conditions (Figure 1).9 In contrast to this, it has been considered that 3 is not an inferred intermediate in the present reaction of 1a giving 2b and 2c due to the strongly basic conditions.
Here we wish to report the molecular orbital calculation study for these ring-opening reactions of dihydropyran (1a) with amines and thiols. Probable pathways from 1a to the dienes, 2b and 2c, were examined on the basis of DFT calculations to clarify the mechanism of this reaction. Moreover, the contrastive reactivity of non-fluorinated dihydropyran (1aa’) with amines under the similar conditions resulted in the complete recovery of 1aa’6 was also elucidated (Figure 1).
RESULTS AND DISCUSSION
The present reaction of dihydropyran (1a) providing dienes is assumed to propose two routes as shown in Scheme 2. In Path A, direct nucleophilic substitution by amines and thiols at C-2 of 1a or elimination-addition processes via 4H-pyran (5) gives the corresponding intermediates, 1b and 1c, respectively. The following elimination of isobutanol from 1b and 1c, and subsequent electrocyclic ring-opening reaction on 2H-pyrans (4b,c) affords the dienes, 2b and 2c, respectively. On the other hand, a preceding elimination of isobutanol from 1a gives 2H-pyran (4a), which undergoes electrocyclic ring-opening to afford butadienyl ether (2a) in Path B. The nucleophilic O-N and O-S exchange reactions occur at α-position of 2a successively to afford 2b and 2c, respectively.
First, we carried out structural optimization of the substrate, 1a, and the intermediate, 5, using RB3LYP/6-31G* as for the Path A. The results are summarized in Figure 2 where the lengths of C-C bond comprising the each pyran rings of 1a and 5 are indicated together with their energy values. The frontier electron densities of LUMO (frN) on some ring carbons of 1a and 5, and the LUMO levels of these two pyrans are also described in Figure 2. Extremely small frN (0.011) at C-2 less than that (0.061) at C-4 on 1a indicates that nucleophiles hardly attack 1a at C-2. As for the energy profiles of the substitution at C-4 and C-2 of 1a, which are giving 1b’, 1c’, and 1ba, 1ca, respectively, the results of model calculations employing dimethylamine and methanethiol are shownin Scheme 3.10
All of these substitutions are predicted to be endothermic processes demanding external energy exhibited in parenthesis. These energy values suggest the probability of substitutions at C-4 in addition to C-2 and, especially in the case of the reaction of 1a with methanethiol, the C-4 substitution giving 1c’ proceeds preferentially over the C-2 substitution affording 1ca, even if the direct substitution at C-2 on 1a affording 1ca is possible. However the products corresponding to 1b’, 1c’ and any products derived from them could not be detected in the crude materials of the reaction of 1a with amines and thiols. Hence, the above results indicate that the direct substitution on 1a giving 1b and 1c would be disallowed.
Two kinds of courses are regarded as tentative dealcoholization step of 1a. One is the ethanol elimination affording 4H-pyran (5) and the other is the isobutanol elimination affording 2H-pyran (4a). To clarify which type of the elimination occurred preferentially, we also carried out calculations as for 4a (Figure 3).
The results indicate that the elimination reactions from 1a to 5 and 4a take place endothermically to absorb 27.3 kcal/mol and 19.3 kcal/mol, respectively. Thus the energy difference of ca. 8 kcal/mol between these two processes suggests that the elimination of isobutanol from 1a giving 2H-pyran (4a) would proceed exclusively. Moreover, frN no more than 0.022 at C-6 on 5 (Figure 2) predicts that nucleophilic addition to 5 affording 1b and 1c hardly occurs even if 5 formed to some extent. Therefore, elimination-addition route via 5 is also considered to be impossible process. Based on the above results, we can conclude that the reactions of 1a giving 2b and 2c do not proceed along Path A.
Next, an examination about Path B should be discussed. As mentioned in the preceding investigation for Path A, the first elimination step from dihydropyran (1a) to 2H-pyran (4a) would occur
predominantly instead of the forming of 5. In order to elucidate the second step, we estimated the transition state structure (TS4a) and its energy are illustrated together with the optimized structures of butadienyl ether (2a) and 2H-pyran (4a) with their energies. Additionally, the steric structures of 4a, TS4a and 2a also provided on the results of IRC calculations of transition state (TS4a) obviously shows the formation of trans-isomer of 2a (s-cis form) via TS4a (Figure 4).11 According to these data, activation energy of this ring-opening is estimated to be 12.2 kcal/mol, and it is also predicted that this ring-opening to 2a (s-trans as a final form) takes place exothermically to release 0.2 kcal/mol. This activation energy is regarded as relatively low, which suggests that this ring-opening reaction would occur smoothly under the very mild conditions as shown in Scheme 1.
The subsequent substitution from butadienyl ether (2a) to the corresponding amine (2b) and sulfide (2c) are assumed to be easily achievable since frN on C-α of 2a is calculated as 0.425 which is enough large value to promote the nucleophilic attack of amines and thiols to that position. Additionally, the analogous successful substitution (S-N exchange reaction) on ethyl butadienyl sulfide (2c’)12 under the mild conditions is also consistent with an implication of the calculational study on this simple processes from 2a to 2b and 2c (Scheme 4).13 Consequently, Path B is concluded to be a reasonable mechanism for the present reaction of dihydropyran (1a) giving the corresponding butadienyl amines (2b) and sulfides (2c).
Lastly, we also examined by conducting calculations as to the non-fluorinated dihydropyrans (1aa’,ab’), 2H-pyran (4a’), transition state (TS4a’), and butadienyl ether (2a’) to emphasize the uniqueness about the reaction of the fluorinated dihydropyran (1a) (Figure 5). The both elimination reactions on 1aa’ and 1ab’ affording 4a’ were estimated to be endothermic processes with 17.4 kcal/mol and 17.7 kcal/mol, respectively. Thus these eliminations are assumed to proceed readily similar to the corresponding step of 1a (19.3 kcal/mol).14 However, the required energy of activation for the step of the ring-opening of 2H-pyran (4a’) giving butadienyl ether (2a’) is calculated as 16.8 kcal/mol, which is ca. 4 kcal/mol higher than the corresponding reaction of 4a. Moreover, 2a’ was calculated to be 4.0 kcal/mol less stable than 4a’. In spite of the adoption of the same conditions which promotes the successful conversion of 1a to 2b, these unfavorable factors would be believed to be the significant reasons for the failure on the reaction of 1aa’ with amines.
CONCLUSION
On the basis of our DFT calculation results, we could have presented the reasonable mechanism for the ring-opening on the reaction of dihydropyran (1a) affording the corresponding butadienyl amines (2b) and sulfides (2c). Our study shows that the elimination of isobutanol from 1a and the subsequent electrocyclic ring-opening on intermediate (4a) give 4,4-bistrifluoroacetyl-1,3-butadienyl ether (2a) initially, and the following nucleophilic O-N and O-S exchange reactions on α-carbon of 2a afford 2b and 2c, respectively. In contrast, the endothermic ring-opening of 4a’ to 2a’ requires relatively high activation energy, which would be the important factor why non-fluorinated dihydropyran (1aa’) did not afford the corresponding butadienyl amines.
Based on the novel knowledge given by a series of our studies regarding 1a, further works are under progress on the syntheses of novel fluorine-containing heterocycles, especially, using butadienyl amines (2b) and sulfides (2c) as building blocks.
COMPUTATIONAL METHODS
All calculations employed in this paper were accomplished using the computer programs packages PC SPARTAN 02 and PC SPARTAN 04.15 All calculations for geometrical optimizations were performed with the 6-31G* basis set at B3LYP16 level. The starting geometries employed for all optimizations were resulted from molecular mechanics using SYBYL17 force field and subsequent semi-empirical PM318 optimizations. The calculations for transition state geometries and their energies were also taken with the 6-31G* basis set at B3LYP level.
References
1. R. Filler and Y. Kobayashi, ‘Biomedicinal Aspects of Fluorine Chemistry,’ Kodansha & Elsevier Biomedical, Tokyo, 1982.
2. R. Filler, ‘Organofluorine Chemicals and Their Industrial Applications,’ Ellis Horwood, London, 1979.
3. J. T. Welch, Tetrahedron, 1987, 43, 3123. CrossRef
4. R. Filler, Y. Kobayashi, and L. M. Yagupolskii, ‘Organofluorine Compounds in Medicinal Chemistry and Biomedical Applications,’ Elsevier, Amsterdam, 1993.
5. M. Hojo, R. Masuda, and E. Okada, Synthesis, 1990, 347. CrossRef
6. M. Hojo, R. Masuda, and E. Okada, Synthesis, 1990, 425. CrossRef
7. M. Hojo, R. Masuda, and E. Okada, Synthesis, 1991, 46. CrossRef
8. N. Ota, E. Okada, A. Sonoda, N. Muro, D. Shibata, and M. Médebielle, Heterocycles, 2008, 76, 215. CrossRef
9. N. Ota, Y. Kamitori, E. Nishiguchi, M. Ishii, and E. Okada, Heterocycles, 2011, 82, 1337. CrossRef
10. The energies of 1ba, 1b’, 1ca, and 1c’ were calculated as followings; 1ba: -1424.36316 au; 1b’: -1345.73141 au; 1ca: -1727.89121 au; 1c’: -1649.26950 au.
11. Since the ring-opening process from 4a to 2a (s-cis form) and the rotation process from 2a (s-cis form) to 2a (s-trans form) are independent to each other, IRC calculation on TS4a could not reproduce the latter rotation process.
12. Frontier electron density (frN) at α-position of this compound was calculated as 0.456.
13. M. Hojo, R. Masuda, and E. Okada, Chem. Lett., 1990, 2095. CrossRef
14. Similar to the case of 1a, the preceding substitutions at C-2 of 1aa’ and 1ab’ (Path A type) were predicted to be disallowed [frN at C-2 are 0.023 (1aa’) and 0.022 (1ab’), and the 2H-pyran (4a’) formation is ca. 7 kcal/mol less endothermic than the corresponding 4H-pyran formation].
15. Wavefunction, Inc.
16. A. D. Becke, J. Chem. Phys., 1993, 98, 5648. CrossRef
17. M. Clark, R. D. Cramer III, and N. van Opdensch, J. Computational Chem., 1989, 10, 982. CrossRef
18. J. J. P. Stewart, J. Computational Chem., 1989, 10, 209. CrossRef