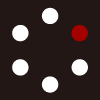
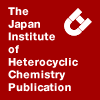
HETEROCYCLES
An International Journal for Reviews and Communications in Heterocyclic ChemistryWeb Edition ISSN: 1881-0942
Published online by The Japan Institute of Heterocyclic Chemistry
e-Journal
Full Text HTML
Received, 1st June, 2011, Accepted, 14th July, 2011, Published online, 21st July, 2011.
DOI: 10.3987/COM-11-12270
■ Aromatic Compounds from Cultured Lichen Mycobionts of Three Graphis Species
Yukiko Takenaka, Nobuo Hamada, and Takao Tanahashi*
Kobe Pharmaceutical University, 4-19-1 Motoyamakita-machi, Higashinada-ku, Kobe 658-8558, Japan
Abstract
Spore-derived mycobionts of three lichen species, Graphis apriens, G. handelii and G. awaensis, were cultivated on a malt-yeast extract medium supplemented with 10% sucrose and their metabolites were investigated. Two new isocoumarin derivatives 1 and 2 as well as a new thiophene derivative 3 were isolated along with six known compounds. Their structures were determined by spectroscopic methods.Lichens are distinct symbiotic entities composed of an algal or cyanobacterial photobiont and a fungal mycobiont. Lichen thalli are well known to produce a great variety of phenolic compounds with different structures and potential biological activities.1,2 Most of them are unique to lichens and termed as lichen substances. Aposymbiotically cultured mycobionts under stressed conditions also produce secondary metabolites but frequently biosynthesize different polyketides than those of the natural lichen.3—10 From our interest in the metabolic capability of the isolated lichen mycobionts, we have investigated the metabolites of the cultured mycobionts of the genera Graphis, Pyrenula3,4 and Lecanora.5 For the genus Graphis, our previous cultivation and chemical investigations were carried out on G. scripta, G. scripta var. pulverulenta, G. scripta var. serpentina, G. prunicola, G. cognata, G. rikuzensis and an unidentified Graphis sp., resulting in the isolation of 6H-dihydro[b,d]pyran-6-one derivatives,6,7 isocoumarins,8 chromones9 and phenyl ethers.10 In this paper, we report the cultivation of the spore-derived mycobionts of Graphis apriens, G. handelii and G. awaensis and the isolation and characterization of three new compounds 1—3 along with six known compounds from their cultures.
The polyspore-derived mycobionts of G. apriens, G. handelii and G. awaensis were cultured on conventional malt-yeast extract medium supplemented with 10% sucrose. After 6—10 months, the cultivated colonies were harvested and extracted with acetone. Purification of the extracts of G. apriens by a combination of preparative TLC and preparative HPLC afforded new compounds 1 and 2 together with six known compounds, 5-hydroxy-2,3-dimethyl-7-methoxychromone (4), 5-hydroxy-3-hydroxy- methyl-2-methyl-7-methoxychromone (5), 5-hydroxy-2-hydroxymethyl-3-methyl-7-methoxychromone (6), 6-methoxymellein (7), graphisquinone (8) and ergosterol (9). From the cultured mycobionts of G. handelii, a novel compound 3 was isolated along with 4, 7 and 8. The cultured mycobionts of G. awaensis also yielded 3, 4 and 7—9. Compounds 4—6 and 8 had already been reported as metabolites of the cultured mycobionts of Graphis sp.9,11 Compound 7, a phytoalexin produced by carrot roots,12 which had been obtained from the plant13 and fungal14 sources, was isolated from the cultured mycobionts for the first time. Ergosterol (9) has so far been isolated from the lichen Xanthoria parietina15 and the cultured lichen micobiont Baeomyces rufus (Huds.) Rebent.16
Compound 1, obtained as a colorless crystalline solid, had a molecular formula of C11H12O5 as established by HR-EIMS. The UV spectrum showed absorption maxima at 232, 268 and 332 nm, and the IR spectrum showed absorption bands at 3271, 1655 and 1643 cm-1, indicating the presence of aromatic chelated carbonyl and hydroxy groups. Its 1H NMR spectrum exhibited a broad singlet for a hydrogen-bonded hydroxyl group at δ 10.96, a singlet for a methoxy group at δ 3.93, a doublet for a methyl group at δ 1.53, and signals for a pair of methylene protons at δ 2.66 and 3.17 and an oxygenated methine proton at δ 4.64. These spectral features were similar to those of 6-methoxymellein (7). However, the 1H NMR spectrum of 1 exhibited only one broad singlet for an aromatic proton at δ 6.41 instead of two meta-coupled aromatic protons in 6-methoxymellein (7). These findings, together with its 13C NMR spectral data and its molecular formula, which was one oxygen atom more than that of 7, implied that compound 1 was a 5- or 7-hydroxylated derivative of 6-methoxymellein. The position of the substituents on the aromatic ring was confirmed by 2D NMR experiments (Figure 2). Significant HMBC correlations from the signal for a hydrogen-bonded hydroxyl group to an oxygenated carbon signal at δ 157.9 assignable to C-8 and an aromatic CH carbon (C-7) and the interactions from methylene protons to another oxygenated carbon signal at δ 134.3 were observed. The NOESY spectrum showed a cross-peak between the aromatic proton and the methoxy signal. These findings indicated that the hydroxy groups were substituted at C-5 and C-8 and the methoxy group located at C-6. This was further supported by comparison of its 13C-NMR spectral data with those of 6-methoxymellein (7) and 5,6-dihydroxymellein (10).14 Thus, the structure of 1 was determined as 5-hydroxy-6-methoxymellein. Chiral HPLC analysis of 1 demonstrated that the isolated compound was not a single isomer but an enantiomeric mixture in a similar case to that of 6-methoxymellein (7) isolated in this study and some isocoumarin derivatives from the cultured mycobionts.3 The CD spectrum of 1 showed a negative Cotton effect at 272 nm, indicating the major enantiomer to have 3R configuration, since (3R)-5,6-dihydroxymellein (10) was reported to show a negative Cotton effect at 273 nm.14 Thus, compound 1 was elucidated to be a mixture of (3R)- and (3S)-5-hydroxy-6-methoxymelleins in the ratio of 3:2.
Compound 2, C11H12O5, was isomeric with 1. Its 1H NMR spectrum exhibited a broad singlet for a hydrogen-bonded hydroxyl group at δ 11.22, a singlet for a methoxy group at δ 3.86, a doublet for a methyl group at δ 1.57 and signals for two aromatic protons at δ 6.49. These spectral features displayed its structural similarity to 6-methoxymellein (7). In the 1H NMR spectrum of 2, however, the signal for an oxygenated methine proton was observed at δ 4.51 (d, J=2.0 Hz) instead of signals for methylene protons. The marked difference between 2 and 7 was accounted for by the hydroxylation at C-4 in 2. This assumption was confirmed by a sequence of COSY correlations from the methyl signal to the oxygenated methine proton and HMBC correlation from the methyl signal to an oxygenated carbon signal at δ 67.6. The methoxy group was located at C-6 by the NOESY correlations between the methoxy signal and two aromatic proton signals (Figure 2). The relative configuration at C-3 and C-4 was determined to be cis by the comparison of the J3,4 value of 2 (2.0 Hz) with those of cis-4,6-dihydroxymellein (11) (2 Hz),17 cis-4-hydroxymellein (12) (2 Hz) and trans-4-hydroxymellein (13) (4 Hz).18 Chiral HPLC analysis of 2 showed two peaks in the ratio of 49:1, indicating that 2 was a mixture of enantiomers. The absolute configuration of the major enantiomer was estimated by comparison of its CD spectrum with that of (3R,4R)-cis-4-hydroxy-5-methylmellein (14), which showed a negative Cotton effect at 254 nm.19 Since a negative sign was found for the Cotton effect of 2 at 267 nm, (3R,4R)-isomer must be predominant in the enantiomeric mixture. Thus, compound 2 was elucidated to be a mixture of (3R,4R)- and (3S,4S)-4-hydroxy-6-methoxymellein with the ratio of 49:1.
Compound 3 was isolated as a red-orange crystalline. The HR-EIMS spectrum of 3 established the composition C11H10O4S. It showed UV maxima at 235, 297, 339 and 450 sh nm, and IR bands at 1670, 1649, 1605 and 1533 cm-1. Its 1H NMR spectrum exhibited signals for an aromatic methyl group at δ 2.56, two methoxy groups at δ 4.04 and 4.06 (each s) and an aromatic proton at δ 7.18. The 13C NMR spectrum of 3 showed one methyl carbon, two methoxy carbons, one sp2 CH carbon and seven sp2 quaternary carbons, two of which were carbonyl carbons (Table 1). These spectral features were very similar to those of graphisquinone (8),11 a metabolite of the culture. The significant HMBC correlations, together with COSY, NOESY and HMQC interactions, the quaternary carbon signals at δ 139.9, 149.8 and 139.2 and aromatic CH carbon signal at δ 124.1 were assigned to C-2, C-3a, C-7a and C-3, respectively (Figure 3). When the chemical shifts of these carbons were compared with those of the corresponding carbons in graphisquinone (8), C-2 and C-7a showed upfield shifts by -20.3 and -9.3 ppm, while C-3 and C-3a showed downfield shifts by +19.6 and +21.8 ppm, respectively. These findings demonstrated that the compound possessed a thiophene ring instead of a furan ring as seen in 8. Accordingly, compound 3 was formulated as shown and designated as thiographisquinone. It is of great interest that the cultured lichen mycobionts produced a unique metabolite with a thiophene ring.
EXPERIMENTAL
General Procedures. Melting points were measured on a Yanaco micro melting point apparatus and are not corrected. The UV spectra were recorded on a Shimadzu UV-240 spectrophotometer, the IR spectra on a Shimadzu FTIR-8200 infrared spectrophotometer and the CD spectra on a Shimadzu AVIV 62A DS. HR-EIMS were obtained with a Hitachi M-4100 mass spectrometer. The NMR experiments were performed with Varian VXR-500 and UNITY INOVA spectrometers with tetramethylsilane as an internal standard. Thin-layer chromatography was performed on pre-coated Kieselgel 60F254 plates (Merck), and spots were visualized under UV light. HPLC was performed using a Waters system (600E Multisolvent Delivery System, Lambda-Max Model 481 Absorbance Detector), and a LaChrom Elite (HITACHI L-7100, HITACHI Diode Array Detector L-7450). Preparative HPLC was carried ou on µBondasphere 5 µC18-100 Å with H2O-MeCN (2:3).
Plant Material. Specimens of Graphis apriens Müll. Arg. were collected from the bark of trees in Yase, Kyoto Prefecture, Japan (100 m alt.). The voucher specimen was identified by Prof. M. Nakanishi, Hiroshima University, Japan, and was deposited at Osaka City Institute of Public Health and Environmental Sciences with registration No. NH 988016. Specimens of Graphis handelii Zahlbr. (NH9933040) and Graphis awaensis Vain. (NH0012931) were collected from the bark of trees on Miyake Island (400 m alt.) and Hachijyo Island (250 m alt.), Tokyo Prefecture, Japan, respectively. The voucher specimens were identified by Prof. H. Miyawaki, Saga University, Japan.
Cultivation of Mycobionts. Mycobionts of Graphis sp. were obtained from the spores discharged from apothecia of a thallus, and were cultivated in 10 mL of modified MY10 medium (malt extract 10 g, yeast extract 4 g, sucrose 100 g, agar 15 g, H2O 1 L, pH 7) in test tubes at 18 ˚C in the dark.
Isolation of Metabolites from the Cultured Mycobionts of G. apriens. After cultivation for 6 months, the harvested colonies (106 test tubes, freeze-dried weight 61.2 g) were continuously extracted with acetone at rt, and the combined extracts were concentrated under reduced pressure to give a residue (122.3 mg). The residue was repeatedly subjected to preparative TLC with toluene-acetone (4:1) and preparative HPLC, giving rise to 1 (1.6 mg), 2 (3.0 mg), 4 (2.0 mg), 5 (2.6 mg), 6 (3.0 mg), 7 (1.5 mg), 8 (6.1 mg) and 9 (22.8 mg).
5-Hydroxy-6-methoxymellein (1): Enantiomeric mixture. Colorless crystalline solid. UV (EtOH) λmax nm (log ε): 232 (4.13), 268 (3.95), 332 (3.74). CD (EtOH) λmax nm (∆ε): 221 (+1.03), 241 (-3.16), 258 (-0.35), 272 (-1.28). IR (KBr) νmax cm-1: 3271, 1655, 1643. 1H-NMR (CDCl3): δ 1.53 (3H, d, J=7.0 Hz, H3-9), 2.66 (1H, ddd, J=17.0, 11.5, 0.5 Hz, H-4), 3.17 (1H, dd, J=17.0, 3.5 Hz, H-4), 3.93 (3H, s, 6-OCH3), 4.64 (1H, dqd, J=11.5, 7.0, 3.5 Hz, H-3), 5.23 (1H, br, 5-OH), 6.41 (1H, br s, H-7), 10.96 (1H, br s, 8-OH). HR-EIMS m/z: Calcd for C11H12O5 [M]+: 224.0685. Found: 224.0684.
4-Hydroxy-6-methoxymellein (2): Enantiomeric mixture. Colorless crystalline solid. UV (EtOH) λmax nm (log ε): 215 (4.32), 230 sh (4.04), 265 (4.01), 304 (3.72). CD (EtOH) λmax nm (∆ε): 212 (+6.30), 235 (-0.68), 244 (-0.16), 267 (-4.20), 305 (+0.65). IR (KBr) νmax cm-1: 3396, 1670, 1638. 1H-NMR (CDCl3): δ 1.57 (3H, d, J=6.5 Hz, H3-9), 3.86 (3H, s, 6-OCH3), 4.51 (1H, d, J=2.0 Hz, H-4), 4.65 (1H, qd, J=6.5, 2.0 Hz, H-3), 6.49 (2H, br s, H-5, 7), 11.22 (1H, br s, 8-OH). HR-EIMS m/z: Calcd for C11H12O5 [M]+: 224.0685. Found: 224.0704.
HPLC Analyses of 1 and 2. Compound 1 was analyzed by chiral HPLC [column, CHIRALCEL OB-H (4.6 i.d. × 250 mm, DAICEL CHEMICAL INDUSTRIES, LTD.); mobile phase, n-hexane-2-propanol (9:1); flow rate, 0.8 mL/min; detection, 254 nm] and demonstrated a major peak at 53 min and a minor one at 58 min (3:2). Compound 2 was subjected to chiral HPLC [column, CHIRALPAK AD-RH (4.6 i.d. × 150 mm, DAICEL CHEMICAL INDUSTRIES, LTD.); mobile phase, H2O-MeCN (7:3); flow rate, 0.6 mL/min; detection, 254 nm]. The minor peak eluted at 11 min and a major one at 13 min (1:49).
Isolation of Metabolites from the Cultured Mycobionts of G. handelii. After cultivation for 10 months, the harvested colonies (39 test tubes, freeze-dried weight 15.87 g) were continuously extracted with acetone at rt and the combined extracts were concentrated under reduced pressure to give a residue (218.5 mg). The residue was repeatedly subjected to preparative TLC (toluene-acetone, 4:1) and preparative HPLC, giving rise to 3 (0.5 mg), 4 (1.0 mg), 7 (0.7 mg) and 8 (4.0 mg).
Isolation of Metabolites from the Cultured Mycobionts of G. awaensis. After cultivation for 7 months, the harvested colonies (63 test tubes, freeze-dried weight 23.26 g) were continuously extracted with acetone at rt and the combined extracts were concentrated under reduced pressure to give a residue (354.7 mg). The residue was repeatedly purified by preparative TLC (toluene-acetone, 4:1) and preparative HPLC to yield 3 (0.9 mg), 4 (1.3 mg), 7 (0.7 mg), 8 (4.4 mg) and 9 (13.3 mg).
Thiographisquinone (3): Red-orange crystalline, mp 111-112 °C (MeOH). UV (EtOH) λmax nm (log ε): 235 (4.01), 297 (4.02), 339 (3.54), 450 sh (2.79). IR (KBr) νmax cm-1: 1670, 1649, 1605, 1533. 1H-NMR (CDCl3): δ 2.56 (3H, d, J=1.0 Hz, 2-CH3), 4.04 and 4.06 (each 3H, s, 2×OMe), 7.18 (1H, m, H-1). EIMS m/z (rel. int.): 238 (87) [M]+, 223 (65), 209 (19). HR-EIMS m/z: Calcd for C11H10O4S [M]+: 238.0300. Found: 238.0292, Calcd for C10H7O4S [M-CH3]+: 223.0092. Found: 223.0065.
ACKNOWLEDGEMENTS
We are grateful to Prof. M. Nakanishi and Prof. H. Miyawaki for identification of the voucher specimens. Thanks are also due to Dr. M. Sugiura (Kobe Pharmaceutical University) for 1H- and 13C-NMR spectra, and to Dr. K. Saiki (Kobe Pharmaceutical University) for MS spectra measurements. This research was financially supported in part by a Grant-in-Aid for Scientific Research (C) (No. 11672129) from the Ministry of Education, Sciences, Sports and Culture of Japan and Kobe Pharmaceutical University Collaboration Fund.
References
1. S. Huneck, in W. Herz, H. Falk, G. W. Kirby, R. E. Moore (eds): Progress in the Chemistry of Organic Natural Products, Vol. 81, pp.1-313, Springer Verlag, Wien, New York (2001).
2. V. Shukla, G. P. Joshi, and M. S. M. Rawat, Phyochem. Rev., 2010, 9, 303. CrossRef
3. Y. Takenaka, T. Tanahashi, N. Nagakura, A. Itoh, and N. Hamada, Phytochemistry, 2004, 65, 3119. CrossRef
4. Y. Takenaka, N. Nagakura, N. Hamada, and T. Tanahashi, Heterocycles, 2010, 81, 1931. CrossRef
5. Y. Takenaka, N. Nagakura, and T. Tanahashi, Z. Naturforsch., 2010, 65c, 637.
6. T. Tanahashi, M. Kuroishi, A. Kuwahara, N. Nagakura, and N. Hamada, Chem. Pharm. Bull., 1997, 45, 1183.
7. T. Tanahashi, Y. Takenaka, N. Nagakura, and N. Hamada, Phytochemistry, 2003, 62, 71. CrossRef
8. T. Tanahashi, Y. Takenaka, N. Nagakura, N. Hamada, and H. Miyawaki, Heterocycles, 2000, 53, 723. CrossRef
9. Y. Takenaka, T. Tanahashi, N. Nagakura, and N. Hamada, Heterocycles, 2000, 53, 1589. CrossRef
10. Y. Takenaka, T. Tanahashi, N. Nagakura, and N. Hamada, Chem. Pharm. Bull., 2003, 51, 794. CrossRef
11. H. Miyagawa, N. Hamada, M. Sato, and T. Ueno, Phytochemistry, 1994, 36, 1319. CrossRef
12. F. Kurosaki and A. Nishi, Phytochemistry, 1983, 22, 669. CrossRef
13. T. R. Govindachari, S. J. Patankar, and N. Viswanathan, Phytochemistry, 1971, 10, 1603. CrossRef
14. K. Krohn, R. Bahramsari, U. Flörke, K. Ludewig, C. Kliche-Spory, A. Michel, H.-J. Aust, S. Draeger, B. Schulz, and S. Antus, Phytochemistry, 1997, 45, 313. CrossRef
15. J. R. Lenton, L. J. Goad, and T. W. Goodwin, Phytochemistry, 1973, 12, 2249. CrossRef
16. B. Renner and E. Gerstner, Z. Pflanzenphysiol., 1982, 107, 47.
17. G. Assante, R. Locci, L. Camarda, L. Merlini, and G. Nasini, Phytochemistry, 1977, 16, 243. CrossRef
18. D. C. Aldridge, S. Galt, D. Giles, and W. B. Turner, J. Chem. Soc. (C), 1971, 1623. CrossRef
19. T. Okuno, S. Oikawa, T. Goto, K. Sawai, H. Shirahama, and T. Matsumoto, Agric. Biol. Chem., 1986, 50, 997. CrossRef