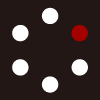
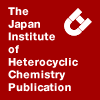
HETEROCYCLES
An International Journal for Reviews and Communications in Heterocyclic ChemistryWeb Edition ISSN: 1881-0942
Published online by The Japan Institute of Heterocyclic Chemistry
e-Journal
Full Text HTML
Received, 14th June, 2011, Accepted, 5th August, 2011, Published online, 8th August, 2011.
DOI: 10.3987/COM-11-12281
■ The Glassy Mesomorphism of Unsymmetrical Bipedal Liquid Crystals Derived from Tetrathiafulvalene and Cholesterol
Ningjuan Zheng, Han Wang, Ruibin Hou, and Bingzhu Yin*
Key Laboratory of Natural Resources of Changbai Mountain & Functional Molecules, Ministry of Education, Yanbian University, Yanji 133002, China
Abstract
1,3-dithiole-2-ones attached two cholesteryl through two ω-thioalkanoyloxy spacer of varying length were easily transformed to the appropriate TTF-based unsymmetrical bipedal by cross-coupling reaction in net triethyl phosphite. All of tetrathiafulvalene-based bipedals exhibit mesogenic phases in a wide temperature region, no crystallization but vitrifying to form glassy mesogens during cooling from the isotropic melt.Tetrathiafulvalene (TTF) derivatives have played a pivotal role in the development of organic materials for optoelectronic application due to their excellent electron-donating properties.1 The transport properties of these materials are clearly dependent on the molecular architecture in the solid state and so a wide variety of substituents have been introduced at the periphery of the TTF core in order to achieve a suitable solid-state organization.2 In this respect, a possible approach is based on the preparation of mesogenic compounds. In particular, glassy liquid crystals (GLCs) hold a fast and good orientation, which can be readily processed into macroscopically ordered solid films.3 Although a considerable number of TTF derivatives have so far been synthesized, there are only a few reports describing TTF derivatives with mesomorphic properties4 and consequently, it is not yet possible to establish structure-property relationships for this family of compounds. Very recently, we reported that the symmetrical tetrapedals derived from TTF and cholesterol exhibited smectic and/or hexagonal columnar phasese, which depended on the length of spacers.5 In order to create new TTF-based mesomorphic states, a series of cholesteryl with various alkyl chain lengths was introduced onto one side of the TTF core. Here, we report the synthesis and mesomorphic properties of TTF-based unsymmetrical bipedals 3a-3f containing two cholesteryls, together with the electron donating property of TTF central core.
The structures and synthetic route of TTF-based bipedals were shown in Scheme 1. The starting 3-dithiole-2-thiones (1a-1f) attached two cholesteryl through two ω-thioalkanoyloxy spacer of varying length were easily transformed to the appropriate ketones (2a-2f) using conventional methods in high yields. The cross-coupling reaction of 2a-2f with 3-dithiole-2-thione yielded the TTF-based unsymmetric bipedals (3a-3f) in net triethyl phosphate at 120 ºC.
The phase sequences and phase structures of 3a-3f were investigated by polarized-light optical microscopy (POM), differential scanning calorimetry (DSC), and small-angle X-ray scatterings (SAXS). All the synthesized bipedals exhibit mesophases in a wide temperature range including room temperature and no crystallization but vitrifying to form glassy mesogen during cooling from the isotropic melt. The 3a-3e exhibited only one mesophase in a wide temperature region. Only 3f with the longest spacer showed two phase transitions in heating as well as in cooling cycle (Table 1). For example, 3c (n=4) exhibited a liquid crystalline phase at a melting state (34.1 ºC), which was transformed to an isotropic phase at 80.5 ºC (Figure 1a). On slow cooling of 3c from the isotropic liquid to liquid crystalline phase at 77.7 ºC, a sanded texture was observed by POM experiment, which was transformed to glassy state at ca. 27.5 ºC during cooling (Figure 2a). The SAXS of 3c measured at cooling to 40 ºC displayed three sharp reflections with d spacings of 519, 260, and 170 nm, which were in the ratio of 3:2:1 and agreed well with (100), (200), and (300) reflections of a lamellar packing structure (Figure1b). Considering the layer thickness (5.19 nm) obtained from the X-ray diffraction pattern is much larger than the estimated molecular length (2.94 nm by CPK model) and the S…S interaction between TTF segments, bimolecule arrangement in lamellar structure is expected, in which TTF segments interdigitated to fill the space. The small-angle X-ray diffraction patterns of 3a, 3b and 3d also displayed similar Bragg diffraction peaks corresponding to the long spacing or layer reflections in the small-angle region and thus also index to a lamellar structure. Considering the similarity of the optical textures with those of 3a-3b and 3d-3e could also
be indexed to lamellar arrangement. The compound 3f (n=7) with the longest spacer is unique in that it displays two mesophases at 22.3 °C and 57.1 °C, followed by transformation to an isotropic phase at 74.7 °C (Figure 1a). Upon cooling from the isotropic liquid, a mosaic texture was observed by POM experiment (Figure 2c). To identify the detailed phase structure, SAXS studies were performed but no useful peak in the lower Bragg angle region was observed at high temperature region (60-70 °C). Considering the phase sequences and optical texture, it probably is a columnar phase. The longer spacer is favorable to a columnar structure packing because it probably provide a bigger disk to avoid the crowding between cholesteryl groups.5 On further cooling to 62.1 °C, the columnar phase gradually transformed to another mesophase, the typical sanded texture appeared and its disordered systems induce vitrification rather than crystallization during cooling (Figure 2b). The X-ray diffraction pattern of 3f measured at cooling to 40 °C displays three sharp reflections corresponding to spacing of 5.88, 2.94, and 1.96 nm in the small-angle region (Figure 1b). They are in the ratio of 3:2:1 and could be indexed as (100), (200), and (300) reflections of a lamellar packing structure. Considering the layer thickness (5.88 nm) obtained from the X-ray diffraction pattern, bimolecule arrangement in lamellar structure is also expected.
To evaluate electron donating property of TTF-based bipedal 3a-3f, the cyclic voltammetry (CV) measurements were performed in a dry CH2Cl2 solution of Bu4NBF4 (0.1 M) with a scan rate of 100 mV s-1 at room temperature (Table 1 and Figure 3a). The bipedal 3a-3f showed two reversible single-electron oxidation peaks at ~0.49 and ~0.95 V, corresponding to the formation of radical cations and dications of TTF, respectively, indicating two sequential reversible one-electron transfer steps. The oxidation potentials of 3a-3f were higher than those of TTF probably due to the existence of electron-withdrawing ester linkages in the distance.
Therefore, as the methylene spacer lengthened (from n = 2 to n = 5), the halfwave potential was anodic shifted in a regular manner.
To investigate the potential of these structures to act as a conducting architecture, chemical oxidation of 3f in a CH2Cl2 solution (10-5 M) was conducted with stepwise addition of I2. Upon stepwise addition of I2 (0.5→2.0 equiv) to a CH2Cl2 solutions of 3f, three new CT absorption bands appeared at 364, 482 and 734 nm (Figure 3b). The absorptions at 364 and 482 nm was assigned to an intramolecular electron transfer of radical cation, 3f +•, while the absorption band at 734 nm was due to an intermolecular electron transfer of the π-dimer of 3f dications.7
In summary, a series of bipedal liquid crystals based on TTF and cholesterol was synthesized by cross-coupling reaction of 1,2-dithiol-2-ones bearing two cholesteryl side chains with 1,2-dithiol-2-thione. All of the TTF-based bipedals exhibit mesophases in a wide temperature range including room temperature and no crystallization but vitrifying to form glassy mesogen during cooling from the isotropic melt. These liquid crystals, in combination with the glassy mesogen and excellent electron-donating properties of TTF, may provide new opportunities in the development of soft materials.
EXPERIMENTAL
NMR spectra were recorded in CDCl3 with a Bruker AV-300 Spectrometer (300 MHz for 1H and 75 MHz for 13C) and chemical shifts were referenced relative to tetramethylsilane (δH/δC = 0). MALDI-TOF-MS was performed on a Shimadzu Axima CFRTM Plus using a 1,8,9-anthracenetriol (DITH) matrix. IR spectra were recorded on a Shimadzu FT-IR Prestige-21 instrument (KBr pressed disc method). UV-vis spectra were recorded on a Hitachi U-3010 spectrophotometer in CH2Cl2 (10-5 M). Cyclic voltammetric studies were carried out on a Potentiostat/Galvanostat 273A instrument in CH2Cl2 (10-3 M) and 0.1 M Bu4PF6 as the supporting electrolyte. Counter and Working electrodes were made of Pt and Glass-Carbon, respectively, and the reference electrode was calomel electrode (SCE). A Perkin-Elmer Pyris Diamond differential scanning calorimeter was used to determine the thermal transitions, the heating and cooling rates were controlled to 10 °C/min. An Olympus BX51-P optical polarized microscope (40×) was used to observe the thermal transitions and to analyze the anisotropic texture. X-ray scattering measurements were performed in transmission mode with Philips PW 1700 X-ray diffractometer. Compounds 1a-1f were synthesized according to our literature method.5
Typical synthetic procedure for 2
Mercuric acetate (9.81 mmol) was added in one portion to suspension of 1 (3.27 mmol) in a mixture of CHCl3 (60 mL) and glacial acetic acid (20 mL), causing the initially yellow solution to change to white within 2 min. The resulting white suspension was stirred at room temperature for 5 h, whereupon the white precipitate was filtered and washed thoroughly with CH2Cl2. The combined organic phase was washed with saturated aqueous NaHCO3 solution, H2O and dried over MgSO4. Evaporation of the solvent in vacuum and the residue was purified by column chromatography (silica gel, CH3Cl) to give 2.
2a. White slimy solid, Yield: 90%, mp 26.99 °C (by DSC). 1H NMR (CDCl3): δ 0.68-2.33 (m, 86H, aliphatic and cholesteric protons are overlapped), 2.66 (t, J = 7.4 Hz, 4H), 3.01 (t, J = 7.4 Hz, 4H), 4.56-4.73 (m, 2H), 5.39 (brs, 2H); FT-IR (KBr, cm-1): 2947 (C-H), 1734 (C=O, ester), 1672 (C=O), 1190 (C-O). Anal. Calcd for C63H98O5S4: C, 71.14; H, 9.29. Found: C, 70.80; H, 9.57.
2b. White slimy solid, Yield: 94%, mp 19.53 °C (by DSC). 1H NMR (CDCl3): δ 0.68-2.31 (m, 90H, aliphatic and cholesteric protons are overlapped), 2.46 (t, J = 7.4 Hz, 4H), 2.91 (t, J = 7.4 Hz, 4H), 4.56-4.73 (m, 2H), 5.38-5.40 (m, 2H); FT-IR (KBr, cm-1): 2949 (C-H), 1732 (C=O, ester), 1678 (C=O), 1171 (C-O). Anal. Calcd for C65H102O5S4: C, 71.51; H, 9.42. Found: C, 71.42; H, 9.75.
2c. White slimy solid, Yield: 92%, mp 10.89 °C (by DSC). 1H NMR (300 MHz, CDCl3): δ 0.67-2.34 (m, 98H, aliphatic and cholesteric protons are overlapped), 0.86 (s, 6H), 2.89 (t, J = 7.0 Hz, 4H), 4.58-4.76 (m, 2H), 5.38-5.46 (m, 2H); FT-IR (KBr, cm-1): 2947 (C-H), 1730 (C=O, ester), 1668(C=O), 1171 (C-O). Anal. Calcd for C67H106O5S4: C, 71.86; H, 9.54. Found: C, 71.60; H, 9.55.
2d. White slimy solid, Yield: 87%, mp 8.03 °C (by DSC). 1H NMR (300 MHz, CDCl3): δ 0.68-2.38 (m, 102H, aliphatic and cholesteric protons are overlapped), 2.89 (t, J = 7.2 Hz, 4H), 4.55-4.75 (m, 2H), 5.38-5.42 (brs, 2H); FT-IR (KBr, cm-1): 2947 (C-H), 1732 (C=O, ester), 1668(C=O), 1171 (C-O). Anal. Calcd for C69H110O5S4: C, 72.20; H, 9.66. Found: C, 71.91; H, 9.35.
2e. White slimy solid, Yield: 90%, mp 6.03 °C (by DSC). 1H NMR (300 MHz, CDCl3): δ 0.67-2.32 (m, 106H, aliphatic and cholesteric protons are overlapped), 2.83 (t, J = 7.2 Hz, 4H), 4.59-4.62 (m, 2H), 5.36-5.37 (m, 2H); FT-IR (KBr, cm-1): 2936 (C-H), 1732 (C=O, ester), 1670 (C=O), 1171 (C-O). Anal. Calcd for C71H114O5S4: C, 72.52; H, 9.77. Found: C, 72.41; H, 10.02.
2f. White slimy solid (Yield: 95%), mp 4.10 °C (by DSC). 1H NMR (CDCl3): δ 0.68-2.40 (m, 110H, aliphatic and cholesteric protons are overlapped), 2.84 (t, J = 7.2 Hz, 4H), 4.52-4.64 (m, 2H), 5.36-5.40 (m, 2H); FT-IR (KBr, cm-1): 2949 (C-H), 1732 (C=O, ester), 1668 (C=O), 1172 (C-O); Anal. Calcd for C73H118O5S4: C, 72.82; H, 9.88. Found: C, 73.00; H, 9.56.
Typical synthetic procedure for 3
2 (0.5 mmol) and 1,3-dithiol-2-thione (0.5 mmol) was added to P(OEt)3 (5 mL) and the suspension was heated to 120 °C, causing dissolution within 1 min, leaving a yellow reaction mixture. The mixture was stirred for 5 h at 120 °C, cooled to room temperature. Addition of MeOH gave a yellow solid, which was filtered, washed with MeOH, and dried in vacuo. The products were purified by column chromatography (silica gel, CH2Cl2:PE = 3:1; v/v) to give a yellow solid. The solid was recrystallized from CH2Cl2/n-Hexane gave 3.
3a. Yellow solid (Yield: 43%). 1H NMR (300 MHz, CDCl3): δ 0.68-2.11 (m, 82H, aliphatic and cholesteric protons are overlapped), 2.33 (d, J = 7.4 Hz, 4H), 2.66 (t, J = 7.1 Hz, 4H), 3.06 (t, J = 7.4 Hz, 4H), 4.64 (m, 2H), 5.38 (brs, 2H), 6.33 (s, 2H); MS (EI): m/z (%) 1149 (M+, 100); FT-IR (KBr, cm-1): 2943 (C-H), 1730 (C=O), 1179 (C-O); Anal. Calcd for C66H100O4S6: C, 68.94; H, 8.77. Found: C, 68.71; H, 9.06.
3b. Yellow solid (Yield: 44%). 1H NMR (300 MHz, CDCl3): δ 0.68-2.08 (m, 86H, aliphatic and cholesteric protons are overlapped), 2.32 (d, J = 7.45 Hz, 4H), 2.45 (t, J = 7.2 Hz, 4H), 2.87 (t, J = 7.4 Hz, 4H), 4.62 (m, 2H), 5.38 (brs, 2H), 6.31 (s, 2H); MS (EI): m/z (%) = 1177 (M+, 100); FT-IR (KBr, cm-1): 2936 (C-H), 1732 (C=O), 1172 (C-O). Anal. Calcd for C68H104O4S6: C, 69.34; H, 8.90. Found: C, 69.50; H, 8.66.
3c. Yellow solid (Yield: 43%). 1H NMR (300 MHz, CDCl3): δ 0.69-2.31 (m, 100H, aliphatic and cholesteric protons are overlapped), 2.83 (t, J = 7.0 Hz, 4H), 4.62 (m, 2H), 5.39 (brs, 2H), 6.31 (s, 2H); MS (EI): m/z (%) = 1205 (M+, 100); FT-IR (KBr, cm-1): 2947 (C-H), 1730 (C=O), 1169 (C-O). Anal. Calcd for C70H108O4S6: C, 69.71; H, 9.03. Found: C, 69.90; H, 9.01.
3d. Yellow solid (Yield: 42%). 1H NMR (300 MHz, CDCl3): δ 0.68-2.32 (m, 102H, aliphatic and cholesteric protons are overlapped), 2.81 (t, J = 7.2 Hz, 4H), 4.62 (m, 2H), 5.38 (brs, 2H), 6.32 (s, 2H); MS (EI): m/z (%) = 1233 (M+, 100). FT-IR (KBr, cm-1): 2945 (C-H), 1734 (C=O), 1170 (C-O). Anal. Calcd for C72H112O4S6: C, 70.08; H, 9.15. Found: C, 69.89; H, 9.34.
3e. Yellow solid (Yield: 51%). 1H NMR (300 MHz, CDCl3): δ 0.67-2.32 (m, 106H, aliphatic and cholesteric protons are overlapped), 2.81 (t, J = 7.2 Hz, 4H), 4.59-4.62 (m, 2H), 5.36-5.38 (m, 2H), 6.32 (s, 2H); MS (EI): m/z (%) = 1260 (M+, 100). FT-IR (KBr, cm-1): 2936 (C-H), 1732 (C=O), 1171 (C-O). Anal. Calcd for C74H116O4S6: C, 70.42; H, 9.26. Found: C, 70.30; H, 9.36.
3f. Yellow solid (Yield: 46%). 1H NMR (300 MHz, CDCl3): δ 0.68-2.32 (m, 110H, aliphatic and cholesteric protons are overlapped), 2.84 (t, J = 7.2 Hz, 4H), 4.62 (m, 2H), 5.37 (brs, 2H), 6.32 (s, 2H); MS (EI): m/z (%) = 1289 (M+, 100). FT-IR (KBr, cm-1): 2930 (C-H), 1736 (C=O), 1175 (C-O). Anal. Calcd for C76H120O4S6: C, 70.75; H, 9.38. Found: C, 70.50; H, 9.54.
ACKNOWLEDGEMENTS
This work was supported by the National Science Foundation of China (No. 21062022) and the Specialized Research Fund for the Doctoral Program of Higher Education (Grant No. 20102201110001).
References
1. (a) M. R. Bryce, Chem. Soc. Rev., 1991, 20, 355; CrossRef (b) M. R. Bryce, J. Mater. Chem., 1995, 5, 1481; CrossRef (c) J. W. Goodby, I. M. Saez, S. J. Cowling, V. Gortz, M. Draper, A. W. Hall, S. Sia, G. Cosquer, S.-E. Lee, and E. P. Raynes, Angew. Chem. Int. Ed., 2008, 47, 2754. CrossRef
2. R. Andreu, J. Barberá, J. Garín, J. Orduna, J. L. Serrano, T. Sierra, P. Leriche, M. Sallé, A. Riou, M. Jubault, and A. J. Gorgues, Mater. Chem., 1998, 8, 881. CrossRef
3. H. P. Chen, D. Katsis, J. C. Mastrangelo, S. H. Chen, and S. D. Jacobs, Adv. Mater., 2000, 12, 1283. CrossRef
4. (a) M. Katsuhara, I. Aoyagi, H. Nakajima, T. Mori, T. Kambayashi, M. Ofuji, Y. Takanishi, K. Ishikawa, H. Takezoe, and H. Hosono, Synth. Met., 2005, 149, 219; CrossRef (b) X. Gao, W. Wu, Y. Liu, S. Jiao, W. Qiu, G. Yu, L. Wang, and D. Zhu, J. Mater. Chem., 2007, 17, 736; CrossRef (c) R. A. Bissell, N. Boden, R. J. Bushby, C. W. G. Fishwick, E. Holland, B. Movaghar, R. J. Bushby, and G. Ungar, Chem. Commun., 1998, 113; CrossRef (d) A. González, J. I. Segura, and N. Martín, Tetrahedron Lett., 2000, 41, 3083; CrossRef (e) R. Andreu, J. Garín, J. Orduna, J. Barberá, J. L. Serrano, T. Sierra, M. Sallé, and A. Gorgues, Tetrahedron, 1998, 54, 3895; CrossRef (f) L. Wang, K.-U. Jeong, and M.-H. Lee, J. Mater. Chem., 2008, 18, 2657; CrossRef (g) I. C. Pintre, J. L. Serrano, M. Blanca, C. L. Folcia, J. Etxebarria, F. Goc, D. B. Amabilino, J. Puigmartí-Luis, and E. Gomar-Nadald, Chem. Commun., 2008, 2523; CrossRef (h) J. Martínez-Perdiguero, I. Alonso, J. Ortega, C. L. Folcia, J. Etxebarria, I. Pintre, M. B. Ros, and D. B. Amabilino, Phys. Rev. E, 2008, 77, 020701; CrossRef (i) I. Alonso, J. Martínez-Perdiguero, C. L. Folcia, J. Etxebarria, J. Ortega, I. C. Pintre, and M. B. Ros, Phys. Rev. E, 2008, 78, 041701. CrossRef
5. R. B. Hou, Z. G. Huang, K. L. Zhong, L.-Y. Jin, and B. Z. Yin, Tetrahedron, 2011, 67, 1238. CrossRef
6. D. J. Green, J. Chem. Soc., Chem. Commun., 1977, 6, 161. CrossRef
7. (a) L. Huchet, S. Akoudad, E. Levillain, and J. Roncali, J. Phys. Chem., B, 1998, 102, 777; CrossRef (b) H. Spanggaard, J. Prehn, M. B. Nielsen, E. Levillain, M. Allain, and J. Becher, J. Am. Chem. Soc., 2000, 122, 9486. CrossRef