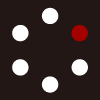
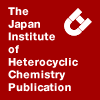
HETEROCYCLES
An International Journal for Reviews and Communications in Heterocyclic ChemistryWeb Edition ISSN: 1881-0942
Published online by The Japan Institute of Heterocyclic Chemistry
e-Journal
Full Text HTML
Received, 15th June, 2011, Accepted, 20th July, 2011, Published online, 28th July, 2011.
DOI: 10.3987/COM-11-12284
■ Synthesis of Hetarenoindanone Based on Selenium Dioxide-Promoted Direct Intramolecular Cyclization
Youlai Zhang, Hiroki Tanimoto, Yasuhiro Nishiyama, Tsumoru Morimoto, and Kiyomi Kakiuchi*
Graduate School of Materials Science, Nara Institute of Science and Technology, 8916-5 Takayama, Ikoma, Nara 630-0192, Japan
Abstract
An alternative and simple method to prepare hetarenoindanone via intramolecular cyclization has been studied. This reaction represents the first example of an intramolecular Friedel-Crafts reaction-oxidation cascade promoted by selenium dioxide by way of Riley allylic oxidation.In recent years, indanone derivatives have been of considerable interest because of their important photosensitizing properties,1 biomedical applications,2 and use as key synthetic intermediates.3 Several methods are known for the synthesis of indanone derivatives. The useful syntheses include classical Friedel-Crafts ring closures of biarylcarboxylic acids and derivatives,4 direct intramolecular arylation of aldehydes with IPy2BF4/HBF4,5 palladium-catalyzed cyclocarbonylation containing a halogen (Br or I),6 intramolecular cyclization of 2-hetarylbenzoic acids and their derivatives under various conditions,7 intramolecular [4+2] cycloaddition reactions of conjugated enynes,8 and oxidation of fluorenes with t-butyl hydroperoxide over Ruthenium-exchanged Montmorillonite K10.9 However, to our knowledge, there has been no report on direct intramolecular cyclization promoted by selenium dioxide (SeO2). In this paper, we disclose the first example of an intramolecular Friedel-Crafts reaction-oxidation cascade promoted by SeO2 to give hetarenoindanone 1 quantitatively.
Recently, we have developed the new photolabile protecting group (PLPG) 2 based on the thiochromene skeleton.10 The novel PLPG 2 was synthesized from thiophenol and ethyl acetoacetate as shown in Scheme 1. In the synthetic route, SeO2 oxidation of 2-methyl-3-phenyl thiochromone 3 afforded the corresponding aldehyde 5 in somewhat low yield followed by reduction of the crude 5 to produce alcohol 4. To clarify the reason for the low yield of the SeO2 oxidation, we examined the reaction in detail to isolate an unexpected formation of indeno[2,1-b]thiochromene-6,11-dione 1 as a byproduct together with the desired 5 (Scheme 1). The structure of 1 was assigned by spectroscopic data and confirmed by X-ray crystallographic analysis as shown in Figure 1.11
It is well known that allylic and benzylic oxidation of methyl groups by selenium dioxide affords aldehydes, but to the best of our knowledge, no example has been reported about the use of selenium dioxide as a promoter of cyclization reactions through carbon–carbon bond formation. Therefore, several oxidation conditions were attempted (Table 1).
In the initial attempt, this oxidation reaction was carried out in chlorobenzene with 1.2 eq. SeO2 at 100 °C, but no reaction was observed (entry 1). When the temperature was raised to 130 °C, aldehyde 5 was isolated after 60 h as a sole product (25% yield, entry 2). Then increasing the concentration to 0.033 mol/L and prolonging reaction time to 115 h caused the increase of the yield of 5 to 39%. At the same time, hetarenoindanone 1 was obtained in 46% yield (entry 3). When the reaction was carried out in o-dichlorobenzene (ODCB) with 2.4 eq. SeO2 at 150 °C, after 7h, the best yield of 5 was obtained (61%, entry 4). It should be noted that, for the longer reaction time, the yield of aldehyde 5 decreased and that of hetarenoindanone 1 increased (entries 4, 5 and 6). Finally, we optimized the reaction in 1.0 mol/L concentration for 3 h to give 1 quantitatively (entry 7).
From these data, it can be hypothesized that hetarenoindanone 1 is produced through aldehyde 5. It is well known that the oxidation reaction of 3 to aldehyde 5 follows Riley selenium dioxide oxidation,12 but the cyclization process was still unclear. Thus, our interest was focused on the detail from aldehyde 5 to hetarenoindanone 1 and the following experiments were designed (Table 2).
First, the reaction was carried out in the absence of SeO2 to give no product (entry 1). This result suggested that thermal energy did not induce cyclization. To our surprise, neither reaction with SeO2 afforded 1 (entry 2). However, when H2O was added (entry 3), the cyclization occurred smoothly. This indicated that the selenous acid generated from SeO2 and H2O works as an acid to promote cyclization. In the case of transformation from 3, H2O is provided during Riley allylic oxidation according to the known mechanism through the intermediates a, b, and c as shown in Scheme 2.13 A mechanistic proposal to account for the formation of hetarenoindanone 1 is also summarized in Scheme 2. The selenous acid promptes intramolecular Friedel-Crafts reaction via the intermediate d to generate the tetracyclic core e. Then aromatization and the subsequent oxidation of the resulting benzyl alcohol f gives the dione 1.
In summary, we developed the quantitative and cascade formation of hetarenoindanone 1 from 2-methyl thiochromone derivative 3 by way of selenium dioxide oxidation. Intramolecular Friedel-Crafts reaction followed by oxidation could be accomplished by selenious acid which is generated from SeO2 and H2O by Riley allylic oxidation.
EXPERIMENTAL
All reactions were conducted in oven-dried (135 °C) glassware under an inert atmosphere of dry nitrogen. The progress of reactions was monitored by silica gel thin layer chromatography (TLC) plates (mesh size 60Å, MERCK). Products were purified by flash column chromatography (FCC) on 40-63 μm silica gel 60 (MERCK). Proton nuclear magnetic resonance spectra (1H NMR) were recorded on JEOL JNM-ECP 500 (500 MHz). Chemical shifts are reported in ppm relative to tetramethylsilane (TMS) as the internal standard. Data is reported as follows: chemical shift, integration, coupling constants (Hz), multiplicity (s = singlet, d = doublet, t = triplet, q = quartet, sept = septet, br = broad, m = multiplet). Carbon nuclear magnetic resonance spectra (13C NMR) were recorded on a JEOL JNM-ECP 500 instrument (125 MHz). Chemical shifts are reported in ppm relative to tetramethylsilane (TMS) as the internal standard (0 ppm in 1H NMR) or the middle peak of chloroform-d (77.0 ppm in 13C NMR).
Starting Materials. 2-Methyl-3-phenyl-4H-thiochromen-4-one (3) was prepared using previously reported procedures.10 All other chemicals in this study were commercially available.
4-Oxo-3-phenyl-4H-thiochromene-2-carbaldehyde (5). A mixture of 2-methyl-3-phenyl-4H-thiochromen-4-one (3) (1.0 mmol, 252 mg) and SeO2 (2.4 mmol, 266 mg) was added to dichlorobenzene (10.0 mL). Then the mixture was heated at 150 °C. The progress of the reaction was monitored by 1H-NMR. After completion of the reaction, sat. NaHCO3 aq. was added, and the aqueous layer was extracted with CH2Cl2. The combined organic extract was washed with water and brine, and then dried over anhydrous MgSO4. The solvent was evaporated and the obtained residue was purified by flash chromatography on silica gel (Hexane−EtOAc, 10:1) to obtain pure product (163 mg, 61%). Yellow solid; mp 138.0-148.2 °C. IR (KBr): 1686, 1669 cm-1. 1H-NMR (500 MHz, CDCl3/TMS): δ = 9.73 (1H, s), 8.53 (1H, d, J = 7.9 Hz), 7.74 (2H, m), 7.60 (1H, dd, J = 6.7, 6.7 Hz), 7.51 (3H, m), 7.39 (2H, m). 13C-NMR (125 MHz, CDCl3/TMS): δ = 190.35, 180.93, 143.80, 143.74, 136.41, 132.51, 132.29, 131.54, 131.21, 129.57, 129.43, 128.38, 128.19, 127.54. HRMS (EI) Calcd for C16H10O2S: 266.0402; Found: 266.0401.
Indeno[2,1-b]thiochromene-6,11-dione (1). A mixture of 2-methyl-3-phenyl-4H-thiochromen-4-one (3) (1.0 mmol, 252 mg) and SeO2 (2.4 mmol, 266 mg) was added to dichlorobenzene (1.0 mL). Then the similar manner was followed as described for 5. After purified by flash chromatography to give 1 (254 mg, 96%). Red solid; mp 241.0-242.5 °C. UV-vis (in methanol): λmax = 313 (ε = 1.37 x 104 M-1 cm-1), 262 (ε = 1.90 x 104 M-1 cm-1) nm. IR (KBr): 1712, 1620 cm-1. 1H-NMR (500 MHz, CDCl3/TMS): δ = 8.65 (1H, d, J = 7.9 Hz), 8.27 (1H, d, J = 7.9 Hz), 7.77 (1H, d, J = 7.9 Hz), 7.69 (3H, m), 7.53 (1H, dd, J = 7.3, 7.3 Hz), 7.28 (1H, d, J = 7.9 Hz). 13C-NMR (125 MHz, CDCl3/TMS): δ = 192.21, 177.86, 144.31, 143.24, 137.80, 135.70, 135.59, 134.18, 132.03, 130.06, 129.08, 128.71, 128.52, 128.38, 124.68, 124.11. HRMS (EI) Calcd for C16H8O2S: 264.0245; Found: 264.0247.
ACKNOWLEDGEMENTS
This research was supported by grant-in-aid for scientific research from Nara Institute of Science and Technology (NAIST) and NAIST Advanced Research Partnership Project, which is gratefully appreciated. We also thank Ms. Huan Zhang, Mr. Yannick Escudie, and Dr. Hiroshi Nomura for their kind help with syntheses of 3, Ms. Yoshiko Nishikawa for HRMS measurement, and Mr. Shouhei Katao for X-ray Crystallographic analysis.
References
1. R. Bauer, G. Heisler, and C. Konigstein, Spectrochim. Acta, Part A, 1994, 50, 57. CrossRef
2. (a) M. T. Tierney and M. W. Grinstaff, J. Org. Chem., 2000, 65, 5355; CrossRef (b) M. L. Greenlee, J. B. Laub, G. P. Rouen, F. DiNinno, M. L. Hammond, J. L. Huber, J. G. Sundelof, and G. G. Hammond, Biorg. Med. Chem. Lett., 1999, 9, 3225; CrossRef (c) P. J. Perry, M. A. Read, R. T. Davies, S. M. Gowan, A. P. Reszka, A. A. Wood, L. R. Kelland, and S. Neidle, J. Med. Chem., 1999, 42, 2679. CrossRef
3. (a) H. Koyama and T. Kamikawa, Tetrahedron Lett., 1997, 38, 3973; CrossRef (b) S. J. Gould, C. R. Melville, M. C. Cone, J. Chen, and J. R. Carney, J. Org. Chem., 1997, 62, 320. CrossRef
4. (a) V. J. Mazzola, K. F. Bernady, and R. W. Franck, J. Org. Chem., 1967, 32, 486; CrossRef (b) A. S. Bailey, P. W. Scott, and M. H. Vandrevala, J. Chem. Soc., Perkin Trans. 1, 1980, 97; CrossRef (c) Z. Yu and D. Velasco, Tetrahedron Lett., 1999, 40, 3229. CrossRef
5. J. Barluenga, M. Trincado, E. Rubio, and J. M. Gonzalez, Angew. Chem. Int. Ed., 2006, 45, 3140. CrossRef
6. (a) X. Zhang and R. C. Larock, Org. Lett., 2005, 7, 3973; CrossRef (b) M. A. Campo and R. C. Larock, J. Org. Chem., 2002, 67, 5616. CrossRef
7. (a) D. Tilly, S. S. Samanta, A. De, A. S. Castanet, and J. Mortier, Org. Lett., 2005, 7, 827; CrossRef (b) A. D. Josey and E. L. Jenner, J. Org. Chem., 1962, 27, 2466. CrossRef
8. R. L. Danheiser, A. E. Gould, R. F. Pradilla, and A. L. Helgason, J. Org. Chem., 1994, 59, 5514. CrossRef
9. M. Nikalje and A. Sudalai, Tetrahedron, 1999, 55, 5903. CrossRef
10. S. Kitani, K. Sugawara, K. Tsutsumi, T. Morimoto, and K. Kakiuchi, Chem. Commun., 2008, 2103. CrossRef
11. Crystallographic data of 1 has been deposited with Cambridge Crystallographic Data Centre as supplementary publication No. CCDC-829244. Copies of the data can be obtained free of charge via http://www.ccdc.cam.ac.uk/data_request/cif.
12. H. L. Riley, J. F. Morley, and N. A. C. Friend, J. Chem. Soc., 1932, 1875. CrossRef
13. (a) C. P. Shah, C. Dwivedi, K. K. Singh, M. Kumar, and P. N. Bajaj, Materials Research Bulletin, 2010, 45, 1213; CrossRef (b) G. R. Waitkins and C. W. Clark, Chem. Rev., 1945, 36, 235. CrossRef