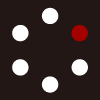
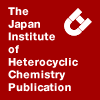
HETEROCYCLES
An International Journal for Reviews and Communications in Heterocyclic ChemistryWeb Edition ISSN: 1881-0942
Published online by The Japan Institute of Heterocyclic Chemistry
e-Journal
Full Text HTML
Received, 21st June, 2011, Accepted, 20th July, 2011, Published online, 28th July, 2011.
DOI: 10.3987/COM-11-12288
■ Utilization of 2-Halo-1,3,4-thiadiazoles in the Synthesis of 2-Functionalized 1,3,4-Thiadiazole Derivatives
Xi-Cun Wang,* Jin-Ge Luo, Xiao-Mei Ding, and Zheng-Jun Quan
Gansu Key Laboratory of Polymer Materials, College of Chemistry and Chemical Engineering, Northwest Normal University, Lanzhou, Gansu 730070, China
Abstract
Novel 2-bromo/iodo-5-aryloxymethyl-1,3,4-thiadiazoles were prepared by the diazotization of 2-amino-1,3,4-thiadiazoles using p-TsOH as acid under the copper-free conditions. 2-Chloro-5-aryloxymethyl-1,3,4-thiadiazoles, which were prepared by traditional diazotization, were treated with various nucleophiles to give a series of 2-substituted-1,3,4-thiadiazole derivatives including 2-methylamino/ethylamino/hydroxylethylamino/hydrazinyl-5-aryloxymethyl-1,3,4-thiadizoles and 6-aryloxymethyl[1,2,4]triazolo[3,4-b][1,3,4]thiadiazoles.INTRODUCTION
1,3,4-Thiadiazoles are five-membered aromatic heterocycles with great utility in synthetic, medicinal, agricultural, and materials chemistry.1-4 The widespread use of 1,3,4-thiadiazoles as a scaffold in medicinal chemistry establishes this moiety as an important bio-active class of heterocycles. It has also been reported in literature that 2-methyl/ethylamino-1,3,4-thiadiazole and [1,2,4]triazolo- [3,4-b][1,3,4]thiadiazoles exhibit diverse biological properties, including antibacterial,5 antimicrobial,6 analgesic,7 anticancer,8,9 anti-inflammatory,10 antidepressant11 and anti-HIV12 activities. On the other hand, halides of 1,3,4-thiadiazoles are important building blocks in modern organic synthesis, which could produce many derivatives from SN displacement by nucleophiles, such as methylamine, ethylamine, hydroxyethylamine, hydrazine hydrate. Generally, chlorides of 1,3,4-thiadiazoles are obtained by the diazotization of 2-amino-1,3,4-thiadiazoles with sodium nitrite, Cu powder in conc. hydrochloric acid.13 Recently, progressive one-pot methods for the introduction of iodine into an aromatic substrate have been suggested. These methods use a sequence involving diazotization-iodination of the corresponding amines with HI/KNO2 in DMSO14 or KI/NaNO2/p-TsOH in CH3CN.15 Victor et al.16 have reported the synthesis of stable arenediazonium tosylates by using a polymer-supported diazotization reagent (“Resin–NO2-”), then, these salts effectively reacted at room temperature with KI, KBr to give the corresponding halides. Although many important processes in organic chemistry and biochemistry involve C-X (Cl, Br, I) formation by diazotization, the synthesis of 2-bromo/iodo-5-aryloxymethyl-1,3,4-thiadiazoles has not been reported.
A triazolothiadiazole system can be viewed as a cyclic analogue of two very important components-thiosemicarbazide17,18 and biguanide,19 which often display diverse biological activities. [1,2,4]triazolo[3,4-b][1,3,4]thiadiazoles were prepared by treatment of 4-amino- 5-substituted-3-mercapto-1,2,4-triazoles with (un)substituted aromatic acids in the presence of phosphorous oxychloride, usually under harsh conditions.20 Moreover, condensation of 1,2,4-triazoles with the acids either in PPA or with para-toluenesulfonyl chloride in toluene or under microwave irradiation in DMF.21,22 Recently, Belen Batanero et al. have reported the synthesis of the final products by anodic oxidation in acetonitrile of 2-arylidene-1-(5-aryl-1,3,4-thiadiazol-2-yl)hydrazine.23
In the previous reports, we demonstrated the use of 2-amino-5-aryl/aryloxymethyl 1,3,4-thiadiazoles as versatile building blocks for the synthesis of functionalized heterocycles, such as 1,3,4-thiadiazole functionalized N-phenylacetamide,24 5H,6H-[1,3,4]thiadiazolo[3,2-a]pyrimidine-7-one,25 2-(5-substituted-1,3,4-thiadiazol-2-ylimino)-4-thiazolidinones,26 2-(N-formyl)-1,3,4-thiadiazoles.27 We now report the investigations on the use of 2-amino-5-aryloxymethyl-1,3,4-thiadiazoles 1 for the synthesis of 2-halo-5-aryloxymethyl-1,3,4-thiadiazoles 2-3 and their derivatives, such as, 2-methylamino/ethylamino/hydroxylethylamino-5-aryloxymethyl-1,3,4-thiadizoles 4a-i, 2-hydrazinyl- 5-aryloxymethyl-1,3,4-thiadizoles 5a-d, and 6-aryloxymethyl[1,2,4]triazolo[3,4-b][1,3,4]-thiadiazoles 6a-d (Scheme 1).
RESULTS AND DISCUSSIONS
We have recently reported the synthesis of 2-chloro-1,3,4-thiadiazoles by diazotization of the 2-amino-1,3,4-thiadiazoles with sodium nitrite, Cu powder in conc. hydrochloric at low temperature.24 In order to obtain these compounds under a mild conditions in the absence of copper or HCl, experiments were carried out for the halogenation reaction of 2-amino-1,3,4-thiadiazoles using the reaction for 2a as a typical reaction (Table 1). Our initial attempts was conducted using p-TSA, PEG-O-SO3H,28 and SiO2-O-SO3H29 as catalyst and KCl as source of chloride. However, no product 3a was observed (entries 1-3). Therefore, we screened different salts LiCl, NaCl, KI, and KBr in subsequent experiments (entries 4-11). No chloro-1,3,4-thiadiazole was detected. Interestingly, we found that 2-iodo- and bromo-1,3,4-thiadiazoles can be obtained in good yields. The best yield of 2a (87%) was obtained by carrying out the reaction at room temperature for 2 h using p-toluenesulfonyl acid as catalyst (entry 8). The acid catalyst has a significant effect on the yield of the reaction and no desired 2-iodo-1,3,4-thiasiazole was obtained in the presence of PEG-O-SO3H, SiO2-O-SO3H (entries 9-10).
These optimal reaction conditions were applied to investigate the scope of the reaction. A wide array of 2-amino-1,3,4-thiadiazoles were subjected to the reaction, and corresponding 2-bromo- and iodo-1,3,4-thidadizaoles (2a-h) were isolated in good yields (Table 2).
Then we investigated nucleophilic substitution of 2-halo-1,3,4-thiadiazoles with several fatty amines (methylamine, ethylamine, hydroxyethylamine) (Table 3). Initially, the reaction mixture of 2-chloro- thiadiazole 3a, methylamine and K2CO3 was reacted for 8 h in ethanol at room temperature and the corresponding compound 4a was obtained with low yield (42%). The employment of 2-bromo- and 2-iodo-thiadiazole (2a and 2e) in the same reaction conditions also gave the product 4a, however, with more lower yield (36% and 10%) (entries 1-3). When 3a reacted with methylamine in the presence of NaOH, the yield of 4a was 35%. Consequently, other temperature was tested and the best yield of 4a (86%) was obtained by carrying out the reaction in the absence of a base at 80 °C for 3 h (entries 5-7). Subsequently, 2-bromo- and 2-iodo-thiadiazole (2a and 2e) were examined in the same reaction condition and the yields of 4a were 67% and 49% (entries 8 and 9). Therefore, 2-chloro-1,3,4-thiadiazole 3a as the starting material was treated with ethylamine and hydroxyethylamine to allow the reactions to proceed smoothly and afforded the desired products 4b, c in high yields. In comparison with the yields of 4a-c, we found out that the nucleophilic activity of fatty amines probably was CH3NH2 > CH3CH2NH2 > HOCH2CH2NH2. Then, other 2-chloro-1,3,4-thiadiazoles with electron-withdrawing groups as well as electron-donating groups, were transformed into corresponding compounds 4d-i in good yields (Table 4).
The reaction for 2-alkylamino-1,3,4-thiadiazoles led us to investigate the synthesis of 2-hydrazino-1,3,4-thiadiazole and its cyclization reaction. When 2-chloro-1,3,4-thiadiazoles 3 and hydrazine hydrate were stirred in ethanol under reflux, the corresponding 5a-d were obtained in good yields after 3 h. Subsequently, compound 5 was treated with formic acid leading to the 6-aryloxymethyl[1,2,4]triazolo[3,4-b][1,3,4]thiadiazoles 6a-d (Table 5).
In conclusion, 2-bromo/iodo-5-aryloxymethyl-1,3,4-thiadiazoles, 2-methylamino/ethylamino/ hydroxylmethyl/hydrazinyl-5-aryloxymethyl-1,3,4-thiadiazole and 6-aryloxymethyl[1,2,4]triazolo- [3,4-b][1,3,4]thiadiazoles using readily available 2-amino-5-aryloxymethyl-1,3,4-thiadiazoles as starting material were prepared. To the best of our knowledge, these compounds have not been reported previously. Furthermore, synthesis and screening of desired compounds based on 1,3,4-thiadiazole scaffolds may lead to the discovery of interesting biological activities.
EXPERIMENTAL
All reagents were obtained commercially and used without further purification. Melting points were determined on an XT-4 electrothermal micromelting point apparatus and uncorrected. IR spectra were recorded using KBr pellets on Nicolet AVATAR 36 FT-IR spectrophotometer. NMR spectra were recorded at 400 (1H) and 100 (13C) MHz, respectively, on a Varian Mercury plus-400 instrument using CDCl3 or DMSO-d6 as solvent and TMS as internal standard. Elemental analyses were performed on a Carlo-Erba 1106 Elemental Analysis instrument. 2-Amino-5-aryloxymethyl-1,3,4-thiadiazoles30 and 2-chloro-5-aryloxymethyl-1,3,4-thiadiazoles24 were prepared as described in the literature procedures.
General procedure for compounds 2a-h. To a solution of p-TsOH (30 mmol) in MeCN (25 mL) was added the 2-amino-1,3,4-thiadiazoles (10 mmol) at rt. The resulting suspension of amine salt was added gradually a solution of NaNO2 (20 mmol) and KI/KBr (25 mmol) in H2O (6 mL). This process was completed for 30 min. Then the reaction mixture was stirred at rt for 2 h. To the reaction mixture was then added H2O (100 mL), 1 M NaHCO3 aqueous (until pH = 9-10) and Na2S2O3 (2 M, 30 mL). The precipitated aromatic iodide was filtered or extracted with EtOAc and purified by flash chromatography (petroleum-EtOAc, 1: 6).
2a: Yield 87%; mp 99-100 °C. 1H NMR (400 MHz, DMSO-d6): δ = 7.36-7.00 (m, 5H, HAr), 5.64 (s, 2H, OCH2). 13C NMR (100 MHz, DMSO-d6): δ = 172.2, 157.2, 129.8 (2C), 122.0, 115.4 (2C), 112.2, 63.6. IR (KBr) ν: 3443, 1597, 1496, 1245 cm-1. MS: m/z = 318 (M+). Anal. Calcd for C9H7IN2OS (317.93): C 33.98, H 2.22, N 8.81. Found: C 34.12, H 2.28, N 8.73.
2b: Yield 76%; mp 133-135 °C. 1H NMR (400 MHz, DMSO-d6): δ = 8.02-6.93(m, 4H, HAr), 5.52 (s, 2H, OCH2). 13C NMR (100 MHz, DMSO-d6): δ = 178.5, 158.2, 155.4, 129.6, 127.8, 122.9,122.3,116.6, 65.0. IR (KBr) ν: 3324, 3179, 1577, 1228 cm-1. MS: m/z = 352 (M+). Anal. Calcd for C9H6IClN2OS (351.89): C 30.66, H 1.72, N, 7.95. Found: C 30.52, H 1.79, N 7.87.
2c: Yield 91%; mp 159-161 °C. 1H NMR (400 MHz, DMSO-d6): δ = 7.26-6.83 (m, 4H, HAr), 5.58 (s, 2H, OCH2), 2.37 (s, 3H, CH3). 13C NMR (100 MHz, DMSO-d6): δ = 171.5, 157.0, 158.4, 127.6 (2C), 124.5, 120.8, 114.2, 65.0, 15.8. IR (KBr) ν: 3327, 3169, 1591, 1221 cm-1. MS: m/z = 332 (M+). Anal. Calcd for C10H9IN2OS (331.95): C 36.16, H 2.73, N 8.43. Found: C 36.31, H 2.62, N 8.58.
2d: Yield 79%; mp 184-186 °C. 1H NMR (400 MHz, DMSO-d6): δ = 8.02-7.11 (m, 4H, HAr), 5.54 (s, 2H, OCH2). 13C NMR (100 MHz, DMSO-d6): δ = 175.5, 156.2, 131.6, 129.8 (2C), 121.9, 117.6 (2C), 65.0. IR (KBr) ν: 3319, 3174, 1587, 1231 cm-1. MS: m/z = 352 (M+), 354 (M+2). Anal. Calcd for C9H6IClN2OS (351.89): C 30.66, H 1.72, N 7.95. Found: C 30.54, H 1.67, N 8.01.
2e: Yield 84%; mp 48-50 °C. 1H NMR (400 MHz, CDCl3): δ = 7.34-6.96 (m, 5H, HAr), 5.46 (s, 2H, OCH2). 13C NMR (100 MHz, CDCl3): δ = 171.4, 157.1, 140.1, 129.8 (2C), 122.3, 114.7 (2C), 64.7. IR (KBr) ν: 3441, 3062, 1595, 1492, 1244 cm-1. MS: m/z = 270 (M+), 272 (M+2). Anal. Calcd for C9H7BrN2OS (269.95): C, 39.87; H, 2.60; N, 10.33. Found: C 39.98, H 2.54, N 10.42.
2f: Yield 73%; mp 109-111 °C. 1H NMR (400 MHz, CDCl3): δ = 7.43-6.99 (m, 4H, HAr), 5.52 (s, 2H, OCH2). 13C NMR (100 MHz, CDCl3): δ = 170.8, 152.8, 140.5, 130.7, 128.0, 123.5, 123.3, 114.3, 66.0. IR (KBr) ν: 3456, 2924, 1585, 1478, 1290 cm-1. MS: m/z = 304 (M+) 306 (M+2). Anal. Calcd for C9H6BrClN2OS (303.91): C 35.37, H 1.98, N 9.17. Found: C 35.45, H 1.91, N 9.26.
2g: Yield 89%; mp 99-101 °C. 1H NMR (400 MHz, CDCl3): δ = 7.26-6.86 (m, 4H, HAr), 5.43 (s, 2H, OCH2). 2.30 (s, 3H, CH3). 13C NMR (100 MHz, CDCl3): δ = 171.7, 155.0, 140.1, 131.8 (2C), 130.2, 114.6 (2C), 65.0, 20.5. IR (KBr) ν: 3447, 2918, 1609, 1508, 1242 cm-1. MS: m/z = 284 (M+) 286 (M+2). Anal. Calcd for C10H9BrN2OS (283.96): C 42.12, H 3.18, N 9.82. Found: C 42.23, H 3.14, N 9.95.
2h: Yield 75%; mp 85-87 °C. 1H NMR (400 MHz, CDCl3): δ = 7.56-6.83 (m, 4H, HAr), 5.36 (s, 2H, OCH2). 13C NMR (100 MHz, CDCl3): δ = 170.6, 151.3, 139.7, 128.3 (2C), 121.8, 112.6 (2C), 64.8. IR (KBr) ν: 3448; 2917; 1576; 1407; 1273 cm-1. MS: m/z = 304 (M+) 306 (M+2). Anal. Calcd for C9H6BrClN2OS (303.91): C 35.37, H 1.98, N 9.17. Found: C 35.12, H 1.92, N 9.28.
General procedure for the synthesis of compounds 4a-i. Fatty amine (3 mmol) was added to a solution of compound 3 (1 mmol) in EtOH (10 mL). Then the reaction mixture was refluxed for 3 h and quenched to ice water. Then the precipitate was filtered off and crystallized from acetone with petroleum ether to afford compounds 4.
4a: Yield 86%; mp 88-90 °C. 1H NMR (400 MHz, CDCl3): δ = 7.32-6.98 (m, 5H, HAr), 6.34 (s, 1H, NH), 5.28 (s, 2H, OCH2), 3.04 (s, 3H, CH3). 13C NMR (100 MHz, CDCl3): δ = 172.8, 157.6, 155.4, 129.6 (2C), 121.8, 114.8 (2C), 64.9, 33.3. IR (KBr) ν: 3222, 2942, 1586 cm-1. MS: m/z = 221 (M+). Anal. Calcd for C10H11N3OS (221.06): C 54.28, H 5.01, N 18.99. Found: C 54.06, H 5.04, N 18.92.
4b: Yield 82%; mp 98-100 °C. 1H NMR (400 MHz, CDCl3): δ = 7.32-6.97 (m, 5H, HAr), 5.84 (s, 1H, NH), 5.28 (s, 2H, OCH2), 3.34 (q, J = 8.0 Hz, 2H, CH2CH3), 1.31 (t, J = 8.0 Hz, 3H, CH2CH3). 13C NMR (100 MHz, CDCl3): δ = 172.3, 157.5, 155.1, 129.6 (2C), 121.6, 114.9 (2C), 64.8, 42.0, 14.6. IR (KBr) ν: 3187, 2924, 1581 cm-1. MS: m/z = 235 (M+). Anal. Calcd for C11H13N3OS (235.08): C 56.15, H 5.57, N 17.86. Found: C 56.39, H 5.54, N 17.80.
4c: Yield 80%; mp 62-64 °C. 1H NMR (400 MHz, CDCl3): δ = 7.31-6.97 (m, 5H, HAr), 6.87 (s, 1H, NH), 5.29 (s, 2H, OCH2), 3.91 (t, J = 4.0 Hz, 2H, CH2OH), 3.53 (t, J = 4.0 Hz, 2H, CH2CH2), 2.79 (s, 1H, OH). 13C NMR (100 MHz, CDCl3): δ = 173.3, 157.5, 155.7, 129.5 (2C), 121.7, 114.8 (2C), 64.8, 61.2, 49.2. IR (KBr) ν: 3270, 2918, 1511 cm-1. MS: m/z = 251 (M+). Anal. Calcd for C11H13N3O2S (251.07): C 52.57 H 5.21, N 16.72. Found: C 52.34, H 5.24, N 16.78.
4d: Yield 84%; mp 113-115 °C. 1H NMR (400 MHz, CDCl3): δ = 7.09 (d, J = 8.0 Hz, 2H, HAr), 6.89 (d, J = 8.0 Hz, 2H, HAr), 6.34 (s, 1H, NH), 5.27 (s, 2H, OCH2), 3.03 (s, 2H, CH3), 2.23 (s, 3H, CH3). 13C NMR (100 MHz, CDCl3): δ = 172.6, 155.5, 155.3, 130.4 (2C), 130.1, 115.0 (2C), 64.5, 33.3, 20.3. IR (KBr) ν: 3206, 2917, 1585 cm-1. MS: m/z = 235 (M+). Anal. Calcd for C11H13N3OS (235.08): C 56.15, H 5.57, N 17.86. Found: C 55.84, H 5.61, N 17.75.
4e: Yield 75%; mp 128-130 °C. 1H NMR (400 MHz, CDCl3): δ = 7.08 (d, J = 8.0 Hz, 2H, HAr), 6.89 (d, J = 8.0 Hz, 2H, HAr), 5.85 (s, 1H, NH), 5.27 (s, 2H, OCH2), 3.35 (q, J = 8.0 Hz, 2H, CH2CH3), 2.23 (s, 3H, CH3), 1.32 (t, J = 8.0 Hz, 3H, CH2CH3). 13C NMR (100 MHz, CDCl3): δ = 172.2, 155.70, 154.9, 130.3 (2C), 130.2, 115.1 (2C), 64.3, 42.1, 20.5, 14.6. IR (KBr) ν: 3168, 2924, 1581 cm-1. MS: m/z = 249 (M+). Anal. Calcd for C12H15N3OS (249.09): C 57.81, H 6.06, N 16.85. Found: C 58.01, H 6.03, N 16.94.
4f: Yield 69%; mp 74-76 °C. 1H NMR (400 MHz, CDCl3): δ = 7.09 (d, J = 8.0 Hz, 2H, HAr), 6.90 (d, J = 8.0 Hz, 2H, HAr), 6.88 (s, 1H, NH), 5.26 (s, 2H, OCH2), 3.90 (t, J = 4.0 Hz, 2H, CH2OH), 3.51 (t, J = 4.0 Hz, 2H, CH2CH2), 2.78 (s, 1H, OH), 2.24 (s, 3H, CH3). 13C NMR (100 MHz, CDCl3): δ = 173.2, 155.8, 155.3, 130.2 (2C), 130.1, 115.1 (2C), 64.9, 42.1, 20.5, 14.6. IR (KBr) ν: 3277, 2918, 1511 cm-1. MS: m/z = 265 (M+). Anal. Calcd for C12H15N3O2S (265.09): C 54.32, H 5.70, N 15.84. Found: C 54.55, H 5.66, N 15.93.
4g: Yield 87%; mp 118-120 °C. 1H NMR (400 MHz, CDCl3): δ = 7.40-6.97 (m, 4H, HAr), 6.35 (s, 1H, NH), 5.34 (s, 2H, OCH2), 3.03 (s, 3H, CH3). 13C NMR (100 MHz, CDCl3): δ = 172.7, 155.4, 153.0, 130.5, 128.0, 123.2, 122.6, 114.5, 65.9, 33.3. IR (KBr) ν: 3130, 2949, 1549 cm-1. MS: m/z = 255 (M+). Anal. Calcd for C10H10ClN3OS (255.02): C 46.97, H 3.94, N 16.43. Found: C 47.22, H 3.96, N 16.35.
4h: Yield 83%; mp 103-105 °C. 1H NMR (400 MHz, CDCl3): δ = 7.40-6.95 (m, 4H, HAr), 5.85 (s, 1H, NH), 5.35 (s, 2H, OCH2), 3.35 (q, J = 8.0 Hz, 2H, CH2CH3), 1.32 (t, J = 8.0 Hz, 3H, CH2CH3). 13C NMR (100 MHz, CDCl3): δ = 172.3, 155.1, 153.0, 130.6, 128.0, 123.4, 122.7, 114.5, 66.0, 42.1, 14.7. IR (KBr) ν: 3193, 2919, 1581 cm-1. MS: m/z = 269 (M+) 271 (M+2). Anal. Calcd for C11H12ClN3OS (269.04): C 48.98, H 4.48, N 15.58. Found: C 49.23, H 4.46, N 15.51.
4i: Yield 76%; mp 138-139 °C. 1H NMR (400 MHz, CDCl3): δ = 7.39-6.94 (m, 4H, HAr), 6.87 (s, 1H, NH), 5.34 (s, 2H, OCH2), 3.90 (t, J = 4.0 Hz, 2H, CH2OH), 3.52 (t, J = 4.0 Hz, 2H, CH2CH2), 2.78 (s, 1H, OH). 13C NMR (100 MHz, CDCl3): δ = 173.2, 155.7, 153.1, 130.5, 127.8, 123.5, 122.8, 114.3, 65.8, 61.1. IR (KBr) ν: 3187, 2904, 1572 cm-1. MS: m/z = 285 (M+) 287 (M+2). Anal.calcd for C11H12ClN3O2S (285.03): C 46.24, H 4.23, N 14.71. Found: C 46.02, H, 4.25, N 14.65.
General procedure for the preparation of compounds 5a-d. To the solution of compound 3 (2 mmol) in EtOH (20 mL) was added hydrazine hydrate solution (85%) in water (3 mmol) and refluxed for 3 h. After completion of the reaction, the reaction mixture was concentrated and cooled to rt to give a solid. Then the precipitation was filtered and recrystallized from EtOH and DMF to give the product 5.
5a: Yield 79%; mp 154-156 °C. 1H NMR (400 MHz, DMSO-d6): δ = 8.80 (s, 1H, NH), 7.30-6.95 (m, 5H, HAr), 5.28 (s, 2H, OCH2), 5.11 (s, 2H, NH2). 13C NMR (100 MHz, DMSO-d6): δ = 178.5, 157.5, 155.4, 129.6 (2C), 121.8, 114.9 (2C), 65.0. IR (KBr) ν: 3324, 3179, 2929, 1594 cm-1. MS: m/z = 222 (M+). Anal. Calcd for C9H10N4OS (222.06): C 48.63, H 4.53, N 25.21. Found: C 48.88, H 4.51, N 25.36.
5b: Yield 89%; mp 187-189 °C. 1H NMR (400 MHz, DMSO-d6): δ = 8.82 (s, 1H, NH), 7.10 (d, J = 8.0 Hz, 2H, HAr), 6.92 (d, J = 8.0 Hz, 2H, HAr), 5.24 (s, 2H, OCH2), 5.10 (s, 2H, NH2), 2.24 (s, 3H, CH3). 13C NMR (100 MHz, DMSO-d6): δ = 178.4, 155.4, 154.9, 130.3 (2C), 130.0, 115.0 (2C), 64.5, 20.2. IR (KBr) ν: 3323, 3192, 2927, 1563 cm-1. MS: m/z = 236 (M+). Anal. Calcd for C10H12N4OS (236.07): C 50.83, H 5.12, N 23.71. Found: C 51.11, H 5.09, N 23.85.
5c: Yield 73%; mp 176-178 °C. 1H NMR (400 MHz, DMSO-d6): δ = 8.82 (s, 1H, NH), 7.39-6.95 (m, 4H, HAr), 5.35 (s, 2H, OCH2), 5.10 (s, 2H, NH2).13C NMR (100 MHz, DMSO-d6): δ = 178.3, 154.8, 153.1, 130.5, 127.9, 123.3, 122.7, 114.4, 65.9. IR (KBr) ν: 3314, 3161, 2924, 1587 cm-1. MS: m/z = 256 (M+) 258 (M+2). Anal. Calcd for C9H9ClN4OS (256.02): C 42.11, H 3.53, N 21.82. Found: C 41.90, H 3.52, N 21.90.
5d: Yield 83%; mp 147-149 °C. 1H NMR (400 MHz, DMSO-d6): δ = 8.82 (s, 1H, NH), 7.07-6.68 (m, 4H, HAr), 5.37 (s, 2H, OCH2), 5.31 (s, 2H, NH2), 3.78 (s, 3H, OCH3).13C NMR (100 MHz, DMSO-d6): δ = 179.1, 159.5, 150.9 (2C), 126.3 (2C), 116.7 (2C), 66.8, 57.6. IR (KBr) ν: 3345, 3172, 2926, 1557 cm-1. MS: m/z = 252 (M+). Anal. Calcd for C10H12N4O2S (252.07): C 47.61, H 4.79, N 22.21. Found: C 47.76, H 4.72, N 22.19.
General procedure for compounds 6a–d. The solution of compound 5 (1 mmol) in formic acid (5 mL) was refluxed for 2 h. After completion of the reaction, the excess formic acid was evaperated under reduce pressure. Then the residue was quenched to ice water and neutralized with saturated NaHCO3 aqueous. The precipitation was filtered and the crude product was recrystallized from EtOH to give pure compounds 6a-d.
6a: Yield 80%; mp 136-138 °C. 1H NMR (400 MHz, DMSO-d6): δ = 9.60 (s, 1H, CH), 7.39-7.35 (m, 2H, HAr), 7.13-7.03 (m, 3H, HAr), 5.70 (s, 2H, OCH2). 13C NMR (100 MHz, DMSO-d6): δ = 167.8, 157.1, 136.4, 129.9, 122.2, 115.1, 64.7. IR (KBr) ν: 3341, 3111, 2923, 1593 cm-1. MS: m/z = 232 (M+). Anal. Calcd for C10H8N4OS (232.04): C 51.71, H 3.47, N 24.12. Found: C 51.60, H 3.56, N 24.01.
6b: Yield 84%; mp 134-136 °C. 1H NMR (400 MHz, DMSO-d6): δ = 9.49 (s, 1H, CH), 7.15 (d, J = 8.0 Hz, 2H, HAr), 6.92 (d, J = 8.0 Hz, 2H, HAr), 5.52 (s, 2H, OCH2), 2.42 (s, 3H, CH3). 13C NMR (100 MHz, DMSO-d6): δ = 167.5, 157.1, 152. 6, 138.2, 126.0, 122.2, 115.3, 65.7, 19.6. IR (KBr) ν: 3329, 3106, 2920, 1585cm-1. MS: m/z = 246 (M+). Anal. Calcd for C11H10N4OS (246.06): C53.64, H 4.09, N 22.75. Found: C 53.55, H 4.17, N 22.86.
6c: Yield 78%; mp 130-132 °C. 1H NMR (400 MHz, DMSO-d6): δ = 9.55 (s, 1H, CH), 7.19-6.88 (m, 4H, HAr), 5.41 (s, 2H, OCH2), 3.77 (s, 3H, OCH3). 13C NMR (100 MHz, DMSO-d6): δ = 167.2, 156.1, 152.4, 141.8, 136.0, 122.2, 115.1, 64.7. 55.6. IR (KBr) ν: 3339, 3109, 2920, 1590 cm-1. MS: m/z = 266 (M+) 268 (M+2). Anal. Calcd for C10H7ClN4OS (266.00): C 45.03, H 2.65, N 21.01. Found: C 45.11, H 2.68, N 21.13.
6d: Yield 82%; mp 135-137 °C. 1H NMR (400 MHz, DMSO-d6): δ = 9.51 (s, 1H, CH), 7.01-6.83 (m, 4H, HAr), 5.43 (s, 2H, OCH2), 3.78 (s, 3H, OCH3). 13C NMR (100 MHz, DMSO-d6): δ = 167.2 161.1, 152.4, 151.4, 139.8, 136.4, 122.7, 115.1, 64.5, 55.9. IR (KBr) ν: 3340, 3110, 2922, 1591cm-1. MS: m/z = 262 (M+). Anal. Calcd for C11H10N4O2S (262.05): C 50.37, H 3.84, N 21.36. Found: C 50.15, H 3.80, N 21.47.
ACKNOWLEDGMENTS
We are thankful for the financial support from the National Nature Science Foundation of China (Nos. 20902073 and 21062017), and Scientific and Technological Innovation Engineering program of Northwest Normal University (nwnu-kjcxgc-03-64 and nwnu-lkqn-10-15).
References
1. R. Jack, N. D. Reid, and J. Heindel, J. Heterocycl. Chem., 1976, 13, 925. CrossRef
2. R. S. Sharma and S. C. Bahel, J. Indian Chem. Soc., 1982, 59, 877.
3. J. Matysiak and A. Opolski, Bioorg. Med. Chem., 2006, 14, 4483. CrossRef
4. F. Bentiss, M. Traisnel, H. Vezin, H. F. Hildebrand, and M. Lagrenee, Corrosion Science, 2004, 46, 2781. CrossRef
5. N. Anbalagan, V. Gunasekaran, and J. T. Leonard, Chem. Pharm. Bull., 2003, 51, 162. CrossRef
6. N. Demirbas, S. A. Karaoglu, A. Demirbas, and K. Sancak, Eur. J. Med. Chem., 2004, 39, 793. CrossRef
7. M. Amir, H. Kumar, and A. S. Javed, Bioorg. Med. Chem. Lett., 2007, 17, 4504. CrossRef
8. T. Wang, Y. H. Zhang, S. Yu, H. Ji, Y. S. Lai, and S. X. Peng, Chin. Chem. Lett., 2008, 19, 928. CrossRef
9. M. X. Wei, L. Feng, X. Q. Li, X. Z. Zhou, and Z. H. Shao, Eur. J. Med. Chem., 2009, 44, 3340. CrossRef
10. U. Salgin-Goksen, N. Gokhan-Kelekci, O. Goktas, Y. Koysal, E. Kilic, S. Isik, G. Aktay, and M. Ozalp, Bioorg. Med. Chem., 2007, 15, 5738. CrossRef
11. F. Clerici and D. Pocar, J. Med. Chem., 2001, 44, 931. CrossRef
12. T. Akhtar, S. Hameed, N. A. Al-Masoudi, and K. M. Khan, Heteroatom Chem., 2007, 18, 316. CrossRef
13. A. Foroumadi, Z. Kiani, and F. Soltani, Il Farmaco, 2003, 58, 1073. CrossRef
14. W. Baik, W. Luan, H. J. Lee, C. H. Yoon, S. Koo, and B. H. Kim, Can. J. Chem., 2005, 83, 213. CrossRef
15. E. A. Krasnokutskaya, N. I. Semenischeva, V. D. Filimonov, and P. Knochel, Synthesis, 2007, 1, 81. CrossRef
16. V. D. Filimonov, M. Trusova, P. Postnikov, E. A. Krasnokutskaya, Y. M. Lee, H. Y. Hwang, H. Kim, and K. W. Chi, Org. Lett., 2008, 10, 3961. CrossRef
17. K. C. Joshi and S. Giri, J. Indian Chem. Soc., 1963, 40, 42.
18. M. C. Goldsworthy, Phytopathology, 1942, 32, 498.
19. J. Haglind, Chem. Abstr., 1966, 64, 16509. Intern. Congr. Chemotherapy, Proc., 3rd, Stuttgart, 1963.
20. T. Karabasanagouda, A. V. Adhikari, and N. S. Shetty, Eur. J. Med. Chem., 2007, 42, 521. CrossRef
21. D. A. Ibrahim, Eur. J. Med. Chem., 2009, 44, 2776. CrossRef
22. S. N. Swamy, Basappa, B. S. Priya, B. Prabhuswamy, B. H. Doreswamy, J. S. Prasad, and K. S. Rangappa, Eur. J. Med. Chem., 2006, 41, 531. CrossRef
23. B. Batanero, R. Saez, and F. Barba, Tetrahedron, 2011, 67, 3076. CrossRef
24. X. C. Wang, S. Q. Wang, X. M. Ding, and X. F. Chen, Phosphorus Sulfur Silicon Relat. Elem., 2010, 8, 1788. CrossRef
25. X. C. Wang, Z. J. Quan, and Z. Zhang, Tetrahedron, 2007, 63, 8227. CrossRef
26. X. C. Wang, G. L. Huang, Z. J. Quan, C. W. Lv, and W. L. Yang, Synth. Commun., 2008, 38, 973. CrossRef
27. X. C. Wang, X. M. Ding, S. Q. Wang, X. F. Chen, and Z. J. Quan, Chin. Chem. Lett., 2010, 21, 301. CrossRef
28. X. C. Wang, Z. J. Quan, F. Wang, M. G. Wang, Z. Zhang, and Z. Li, Synth. Commun., 2006, 36, 451. CrossRef
29. T. S. Jin, H. X. Wang, K. F. Wang, and T. S. Li, Synth. Commun., 2004, 34, 2993. CrossRef
30. A. A. F. Wasfy, S. A. Nassar, and A. M. F. Eissa, Indian J Chem. Sect. B., 1996, 35, 1218.