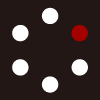
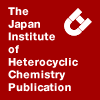
HETEROCYCLES
An International Journal for Reviews and Communications in Heterocyclic ChemistryWeb Edition ISSN: 1881-0942
Published online by The Japan Institute of Heterocyclic Chemistry
e-Journal
Full Text HTML
Received, 7th July, 2011, Accepted, 5th October, 2011, Published online, 7th October, 2011.
DOI: 10.3987/COM-11-S(P)78
■ Synthesis of 6-Hydroxyisochromenes and 6-Hydroxyisocoumarins from 3-Ethoxycyclohex-2-en-1-one
George Majetich* and Jeremy L. Grove
Department of Chemsitry, The University of Georgia, Athens, Georgia 30602, U.S.A.
Abstract
The synthesis of alkylated 6-hydroxyisochromenes and alkylated 6-hydroxyisocoumarins from 3-ethoxycyclohex-2-en-1-one was developed. The key reaction features a novel intramolecular hydroalkoxylation of a cyclohex-2-en-3-yn-1-one to assemble the dihydropyran moiety. Aromatization of the cyclohex-2-en-1-one ring used a selenylation/oxidative elimination sequence. The isochromenes were oxidized to their isocoumarin analogues using DDQ.Our synthesis of (–)-salviasperanol featured a novel intramolecular Friedel–Crafts reaction, also known as a cyclialkylation.1 In particular, enynone 1 was treated with excess Lewis acid and a catalytic amount of ethanethiol and formed 6,7,6-fused tricycle 2 in 94% yield (Scheme 1).2 In order to synthesize the icetexone diterpenes,3 enynone 3 with a benzyl ether at C-19 was prepared. Although the desired ring closure occurred in 85% yield (cf. 4), all conditions used to deprotect the benzyl ether also reduced the cyclohexene double bond. A p-methoxybenzyl ether (PMB), a more labile protecting group, was also
examined. Although PMB ethers can be deprotected by using Lewis acid catalysts and thiols,4 we hoped that the cyclization of 5 to tricycle 6 would occur without the loss of the PMB ether.5 In contrast to enynones 1 and 3, excess BF3·Et2O and a catalytic amount of EtSH failed to promote the cyclization of 5. Instead, treatment of 5 with two equivalents of BF3·Et2O and 1.2 equivalents of EtSH in dilute DCM at room temperature produced dihydropyran 7 in 85% yield (Scheme 2). This transformation begins with the Lewis acid activation of the oxygen of the C-19 ether to produce intermediate i. The ethanethiol present undergoes SN2 displacement at the benzylic position of the PMB ether to generate intermediates ii and iii; proton transfer produces thioether PMBSEt (iv) and alcohol v. Because an excess of ethanethiol was used, enynone v then undergoes 1,6-addition of EtSH to generate vinyl sulfide vi in situ. Dihydropyran formation results from an addition/elimination sequence as shown (i.e., vi→ vii → viii → 7). The rapid nature of this transformation precluded the isolation of alcohol v or vinyl sulfide vi.
It occurred to us that, by analogy with the formation of v, enynone 11 would undergo an intramolecular hydroalkoxylation to produce dihydropyran 10 having the salient features of the isocoumarins (Scheme 3). Enynone 11 was expected to be prepared from alcohol 12, the product of an aldol reaction of formaldehyde and the kinetic enolate of 3-ethoxycyclohex-2-en-1-one (13).6 Many reagents aromatize cyclohexenones, making the transformation of 10 → 9 attractive to us. The final synthetic step would be the benzylic oxidation of isochromene 9 to produce 6-hydroxyisocoumarin 8. The simplicity of this unknown route warranted its investigation.
A large number of naturally-occurring 6-hydroxyisocoumarins have been isolated from plants, molds, lichens, bacteria, and insect sources.7 Many isocoumarins exhibit useful biological activity (Figure 1), i.e., galloflavin, which has a gallic acid subunit, is an HIV-inhibitor whereas brevifolin carboxylic acid, isolated from the Rose plant Geranium Bellum, inhibited triosephosphate isomerase from Trypsanoma cruzi and showed cytotoxic activity in the low micromolar range.7b,c In addition, bergenin has been identified as the antiarrythmic constituent in Fluggea virosa.7d,e
Chart 1 presents four representative strategies to prepare isocoumarins from a plethora of routes which have been extensively reviewed.8 The most versatile method for the construction of isocoumarins is the transition-metal mediated cycloisomerization of alkenyl- or alkynylbenzoic acid derivatives. For example, Hegedus and co-workers treated the sodium salt of o-bromobenzoates (ix) with π-allylnickel bromide to afford allyl benzoates x (eq. 1).9 These carboxylic acids were then treated with PdCl2 in the presence of Na2CO3 to yield 3-substituted isocoumarins (cf. xi). The directed o-metallation of aromatic amides, carbamates, or other directing groups allowed for electrophile trapping, to form isocoumarins with a high degree of structural diversity (xii → xiii → xiv, eq. 2).10 In 1984, Larock reported that o-thallated benzoic acid derivative xv was produced by treating benzoic acid with Tl(O2CCF3)3 (eq. 3). Intermediate xv underwent vinylation and subsequent Pd(II)-catalyzed cyclization to produce 3-substituted isocoumarin xvi.11 Yamamoto and co-workers have developed many methods to prepare isocoumarins. For example, they have reported that Cu(I) catalysis in the presence of an alcohol formed only acetal xvii, while Cu(II) catalysis produced a mixture of acetals xvii and xviii in 89% yield in a 1:1 ratio (eq. 4).12 While these methods represent efficient routes to prepare the isocoumarin framework, they are all limited to the availability of an appropriately substituted aromatic precursor. Herein, we report a new approach to prepare alkylated 6-hydroxyisochromenes and alkylated 6-hydroxyisocoumarins whereby the dihydropyran ring is formed before the aromatic ring is formed.
RESULTS AND DISCUSSION
We believed that bubbling gaseous formaldehyde into a solution containing the kinetic enolate derived from 13 would readily produce aldol product 12 (Scheme 4).13 Instead, bis-hydroxymethylated diol 14 was the major product (36% yield). The formation of 14 was overcome for the most part by adding vacuum-dried solid paraformaldehyde to a solution of the preformed kinetic enolate stirred at 0 °C. Although this reaction never went to completion, workup and isolation afforded 12 (60%), recovered 13 (32%), and only a trace of 14. The next step was to introduce the enynone moiety. Although the addition of lithium acetylide to enone 12 would give 16 directly, lithium (trimethylsilyl)acetylide was more convenient to work with. Enone 12 in THF was added dropwise to a solution of lithium (trimethylsilyl)acetylide stirred at 0 °C until TLC analysis indicated that the 1,2-addition was complete. Note that this reaction did not suffer retro-aldol fragmentation (i.e., 12 → 13). The trimethylsilyl group was removed from alkyne 15 with TBAF in 82% yield. Treatment of enynone 16 with 1.5 equivalent of BF3·Et2O in the presence of 1.2 equivalents of EtSH rapidly formed vinyl sulfide 17 by the 1,6-addition of the ethanethiol; 17 could be isolated or transformed in 82% yield to dihydropyran 10 upon continued exposure to Lewis acid. The mechanism for the formation of 10 parallels the mechanism that was presented in Scheme 2.
The aromatization of dihydropyran 10 would give 6-hydroxyisochromene 9. We first tried to achieve this transformation via a one-pot bromination/elimination sequence (Scheme 5). However, the treatment of enone 10 with NBS, Br2, or pyridinium hydrobromide perbromide under a variety of different conditions gave a mixture of bromides 18 and 19 (unoptimized). Bromination occurred first at C-5 because even conjugated enolates such as zwitterion xix react with electrophiles at the α-position.14 The in situ generation of HBr would promote enolization of the carbonyl group, resulting in further bromination of 18 at the C-7 position. We are confident that bromides 18 and 19 will serve as precursors for the synthesis of halogenated isocoumarins such as aviennin A (20).7k
The next aromatization procedure we investigated featured the conversion of a cyclohexenone to a cyclohexadienone using a selenoxide elimination procedure so that tautomerization of the nascent dienone (cf. xx) would produce the isochromene arene ring (Scheme 6).15 Treatment of 10 with LDA at -78 °C, followed by the addition of a solution of PhSeCl, gave inconsistent results. However, simply adding 1.5 equivalents of solid PhSeCl to a solution of 10 in THF at room temperature produced phenylselenide 21 within a few hours, whereas using EtOAc as the solvent resulted in decomposition. Phenylselenide 21 was then treated with a threefold excess of 30% aqueous H2O2 at room temperature. Although THF is generally used as the solvent when aqueous H2O2 is the oxidant, the reaction time was shortened to < 30 minutes instead of overnight if EtOAc was used. Standard ethereal workup was modified to include two basic washes (saturated aqueous NaHCO3) to ensure complete removal of excess H2O2 and the elimination byproduct PhSeOH; otherwise significant decomposition was observed.
Since many oxidants are known to convert isochroman 22 to isochroman-1-one 23,16 the oxidation of isochromene 9 to isocoumarin 8 was expected to be trivial. Unfortunately, 6-hydroxyisocoumarin 8 was produced from 9 in only 37% yield using 3 equivalents of CrO3 in wet acetic acid at low temperatures (Scheme 7). Other oxidants such as selenium dioxide, chromium trioxide, potassium permanganate, PCC, or sodium hypochlorite, failed to react.
DDQ can oxidize benzylic, allylic, and heteroatom-activated methylenes and methines.17 Xu18 and others19 have used DDQ in the presence of alcohols to oxidize isochromans to the corresponding C-1 acetals. In our hands, the treatment of 6-hydroxyisochromene 9 with 2.0 equivalents of DDQ in 1,4-dioxane in an open flask gave a 91% yield of isocoumarin 8 (Scheme 8). We believe that the oxidation of 9 occurs because of capricious water in the p-dioxane, and not oxygen from the air. The addition of trace water to isochromenylium intermediate xxi would generate hemiacetal xxii that is further oxidized by the excess DDQ present to intermediate xxiii to form isocoumarin 8 on deprotonation. Isocoumarin 8 was also produced when the DDQ oxidation of 9 was carried out in degassed or wet p-dioxane thus confirming that water is the oxygen source.
Since enone 13 is amenable to several synthetic manipulations, we wanted to prepare dialkylated isochromene derivatives by systematically modifying the general strategy (Scheme 9). The first modification studied was to carry out the aldol reaction of 13 with an aliphatic aldehyde culminating in the formation of isochromene A with an alkyl substituent at C-1. We were also curious to see whether substitution at the terminal position of the alkyne moiety would produce a C-3 substituted isochromene (cf. B).
Our first functionalization of the 6-hydroxyisochromene skeleton was to introduce a substituent at the C-1 position (Scheme 10). This was accomplished by adding an aldehyde, such as acetaldehyde, propionaldehyde, isobutyraldehyde, pentanal, or benzaldehyde, to the kinetic enolate of 13, giving the desired aldol adducts in excellent yields and with the diastereomeric ratios shown. While all sets of diastereomers (24A-D) could be separated by silica gel chromatography, they were carried forward as diastereomeric mixtures. It should be noted that when benzaldehyde was used in the aldol reaction with 13, some retro–aldol reaction was observed even at -78 °C. Thus, this reaction occurred in low yield and further attempts to introduce an aromatic substituent at the C-1 position of the 6-hydroxyisochromene skeleton were abandoned. Conversion of hydroxyketones 24A-D to enynones 25A-D was accomplished as described earlier (cf. Scheme 3). A retro-aldol process was observed when 2.5 equivalents of lithium (trimethylsilyl)acetylide were added to the carbonyl component at 0 °C. Fortunately, carrying out the 1,2-addition of the acetylide anion at -78 °C precluded these side reactions and cleanly gave TMS-enynones 25A-D. The trimethylsilyl group was removed using TBAF to furnish enynones 26A-D in high yields.
Subjecting enynones 26A-D to 1.5 equivalents BF3·Et2O and 1.2 equivalents of EtSH in DCM at room temperature for 4-6 hours gave substituted dihydropyrans 28A-D in very good yields (Scheme 11). We were concerned that the secondary alcohol in 26A-D could dehydrate under the cyclization conditions used to give 27A-D, but this elimination did not occur. Aromatization of the cyclohexenone ring was achieved using the selenylation/oxidation strategy to generate C-1 substituted isochromenes 29A-D in very high yield.20
The introduction of a substituent at the C-3 position of the 6-hydroxyisochromene and isocoumarin skeletons was accomplished by adding a substituted acetylide anion to enone 12 (Scheme 12). Treatment of 12 with an excess of the lithium anion of 1-hexyne at 0 °C, followed by acid hydrolysis gave 30A in excellent yield. Phenylacetylene, which introduced an aryl group at the C-3 position, added similarly (cf. 30B). Dihydropyran formation, followed by the aromatization of 30A-B to isochromenes 31A-B occurred in very good to high yields. Oxidation to the C-3 substituted isocoumarins 32A-B was accomplished with DDQ in 1,4-dioxane at room temperature. The overall yield of this sequence from 13 is comparable to that of the cycloisomerization of substituted alkenylbenzoic acids.
In conclusion, we have developed a practical route to prepare 6-hydroxyisochromenes and 6-hydroxyisocoumarins that feature a novel intramolecular hydroalkoxylation reaction, followed by the aromatization of the cyclohexenone ring. This method provided access to C-1 and C-3 substituted 6-hydroxyisochromenes via slight modifications to the synthetic route. In the final step the 6-hydroxyisochromenes were efficiently oxidized to 6-hydroxycoumarins using DDQ. Further work is ongoing to fully test the scope and limitations of this new method for heterocycle formation.
EXPERIMENTAL SECTION
General Procedures: All reactions were run under a nitrogen atmosphere and monitored by TLC analysis. Unless otherwise indicated, all extractive workups consisted of the following procedure: The reaction was slowly quenched by the addition of saturated aqueous NaHCO3. The aqueous layer was extracted with two portions of Et2O. The combined ether extracts were washed with water, brine, and dried over anhydrous sodium sulfate. Filtration, followed by concentration at reduced pressure on a rotary evaporator and at 100 torr to a constant weight, afforded a crude residue which was purified using silica gel 60 (230-400 mesh ASTM) and reagent grade petroleum ether (pet ether), Et2O, and EtOAc. 1H and 13C NMR spectra were recorded on Bruker AVB-400 and DRX-500 MHz spectrometers with 13C operating frequencies of 100 MHz and 125 MHz, respectively. 1H NMR spectra were obtained in CDCl3 and were calibrated using trace CHCl3 present (δ 7.27) as an internal reference. 13C NMR spectra were obtained in CDCl3 and were calibrated using trace CHCl3 present (δ 77.23) as an internal reference.
Preparation of 3-ethoxy-6-(hydroxymethyl)cyclohex-2-en-1-one (12): To a solution of diisopropylamine (33 mL, 0.23 mol) dissolved in 250 mL THF at -78 °C was added n-butyllithium (93.6 mL, 0.23 mol) over a 5-min period. The resulting mixture was allowed to rise to 0 °C over 30 min. A solution of 13 (30.00 g, 0.21 mol) dissolved in 50 mL of THF was added using a cannula to the reaction mixture over a 5-min period and the resulting mixture was stirred at 0 °C for an additional 30 min. Solid paraformaldehyde (9.50 g, 0.32 mol) was added to the reaction mixture in one portion and the reaction was kept at 0 °C for 15 min, at which time the reaction was quenched with ammonium chloride (50 mL). Standard extractive workup, followed by silica gel chromatography (elution with pet ether/EtOAc = 1:1) gave 16.3 g (89% brsm, 60% conversion) of 12 as a dark red oil which was homogeneous by TLC analysis [Rf (12) = 0.31, 1:1, pet ether/EtOAc]: 1H NMR (400 MHz, CHCl3) δ 5.24 (s, 1H), 3.91 (septet, J = 7.1 Hz, 2H), 3.76-3-82 (m, 1H), 3.70 (bs, 1H), 3.52 (bs, 1H), 2.49-2.56 (m, 1H), 2.37-2.44 (m, 2H), 1.90-1.98 (m, 1H), 1.75 (dq, J = 12.7, 5.0 Hz, 1H), 1.36 (t, J = 7.0 Hz, 3H); 13C NMR (100 MHz, CHCl3) δ 202.5, 178.5, 102.6, 64.7, 63.7, 46.9, 28.8, 24.1, 14.2.
3-Ethoxy-6,6-bis(hydroxymethyl)cyclohex-2-en-1-one (14) was generated when the above aldol reaction (preparation of 12) was carried out under extended reaction time. Silica gel chromatography (elution with 100% EtOAc) gave variable yields of 14 as a yellow oil (36% yield) which was homogeneous by TLC analysis [Rf (14) = 0.62, 100% acetone]: 1H NMR (400 MHz, CHCl3) δ 5.17 (s, 1H), 4.12 bs, 2H), 3.79 (q, J = 6.9 Hz, 2H), 3.63 (d, J = 11.2 Hz, 2H), 3.51 (d, J = 11.2 Hz, 2H), 2.38 (t, J = 6.3 Hz, 2H), 1.76 (t, J = 6.3 Hz, 2H), 1.24 (t, J = 7.0 Hz, 3H); 13C NMR (100 MHz, CHCl3) δ 204.2, 178.6, 102.2, 64.8, 62.9, 49.9, 25.8, 24.6, 14.2.
General Procedure A (1,2-Addition of Acetylide Anion to a Cyclohex-2-en-1-one): To a solution of TMS-acetylene (2.5 equiv., ~50 mmol) in 75 mL of THF at -78 °C was added n-butyllithium (2.5M, 2.2 equiv., ~44 mmol) over a 2-min period. The resulting mixture was stirred at -78 °C for 30 min, and then warmed to 0 °C over a 30-min period. The resulting solution was cooled to -78 °C and a solution of enone-alcohol 12 (1.0 equiv., ~20 mmol) dissolved in 20 mL of THF was then added via cannulation over a 5-min period. The resulting reaction mixture was stirred at -78 °C for 1 h. The reaction mixture was quenched by the addition of water (20 mL), followed by the portion-wise addition of aqueous 6 M HCl (50 mL). After warming the resulting solution to rt, and stirring for 30 min, the resulting solution was subjected to standard extractive workup to yield the crude TMS-enynone, which was used in the next step without further purification or characterization. To a solution of crude TMS-enynone dissolved in 150 mL of THF at rt was added TBAF-trihydrate (2.5 equiv., ~50 mmol) in a single portion. The resulting solution was stirred for 5 min. Standard extractive workup yielded the crude enynone, which was purified by silica gel chromatography.
Preparation of 3-ethynyl-4-(hydroxymethyl)cyclohex-2-en-1-one (16): Enone 12 (3.50 g, 21 mmol) was reacted according to general procedure A, but was warmed to 0 °C after the addition of enone 12 to the solution of lithium (trimethylsilyl)acetylide. The crude silyated enynone 15 was deprotected as described in general procedure A to give crude enynone 16. Silica gel chromatography (elution with pet ether/EtOAc = 1:1) gave 2.54 g (82%) of 16 as a yellow oil which was homogeneous by TLC analysis [Rf (16) = 0.26, 1:1, pet ether/EtOAc]: 1H NMR (400 MHz, CHCl3) δ 6.31 (s, 1H), 3.91 (s, 2H), 3.62 (s, 1H), 2.32-2.66 (m, 5H) 2.10-2.19, (m, 2H); 13C NMR (100 MHz, CHCl3) δ 198.9, 143.0, 135.9, 88.9, 81.5, 63.8, 41.6, 35.7, 25.1.
General Procedure B (Dihydropyran Formation): To a solution of enynone (~15 mmol) and ethanethiol (1.2, equiv., ~18 mmol) in 100 mL of DCM at 0 °C was added BF3·Et2O (1.5 equiv., ~33 mmol) over a 2-min period. The resulting solution was stirred at rt for 6 h. The reaction mixture was diluted with Et2O (100 mL) and 50 mL H2O (100 mL) and stirred vigorously at rt for 5-min. Standard extractive workup gave the crude pyran.
Preparation of 8,8a-dihydro-1H-isochromen-6(7H)-one (10): Enynone 16 (2.20 g, 15 mmol) was reacted according to general procedure B. Purification using silica gel chromatography (elution with pet ether/EtOAc = 4:1) gave 1.8 g (82%) of 10 as a red oil which was homogeneous by TLC analysis [Rf (10) = 0.26, 1:1, pet ether/EtOAc]: 1H NMR (400 MHz, CHCl3) δ 6.81 (d, J = 5.7 Hz, 1H), 5.72 (s, 1H), 5.56 (d, J = 5.7 Hz, 1H), 4.34 (dd, J = 5.4, 10.8 Hz, 1H), 3.65 (dd, J = 13.3, 11.4 Hz, 1H), 2.80-2.89 (m, 1H), 2.54-2.61 (m, 1H), 2.44-2.50 (m, 1H), 1.99-2.03 (m, 1H), 1.55-1.63 (m, 1H); 13C NMR (100 MHz, CHCl3) δ 198.7, 153.0, 152.8, 119.2, 104.7, 70.4, 37.3, 34.1, 25.4.
General Procedure C (Aromatization of the Cyclohex-2-en-1-one):
To a solution of cyclohexenone (~3 mmol) in THF (20 mL) was added PhSeCl (4.5 mmol) in one portion. The reaction mixture was stirred until TLC analysis indicated the consumption of the starting pyran. Standard ethereal workup gave a crude α-phenylselenide, which was used directly in the next step without purification or characterization.
To a solution of phenylselenide in EtOAc (20 mL) at rt was added a solution of hydrogen peroxide (30% in water, 9 mmol). The resulting solution was stirred at rt for 30 min, at which time Et2O (20 mL) was added to the reaction mixture. The organic layer was washed with water (5 mL), saturated aqueous NaHCO3 (5 mL), water (4x5 mL), and brine (5 mL). The crude isochromene was obtained by drying over anhydrous sodium sulfate, filtered, and then concentrated under reduced pressure.
Preparation of 1H-isochromen-6-ol (9): Dienone 10 (650 mg, 4.3 mmol) was reacted according to general procedure C. Purification using silica gel chromatography (elution with pet ether/EtOAc = 4:1) gave 505 mg (78%) of 9 as a clear colorless oil which was homogeneous by TLC analysis [Rf (9) = 0.26, 2:1, pet ether/EtOAc]: 1H NMR (400 MHz, CHCl3) δ 6.87 (d, J = 8.0 Hz, 1H), 6.60-6.66 (m, 2H), 6.47 (s, 1H), 5.74 (d, J = 5.6 Hz, 1H), 5.00 (s, 2H); 13C NMR (100 MHz, CHCl3) δ 155.9, 146.9, 132.1, 125.4, 120.6, 113.2, 110.2, 105.5, 68.0.
General Procedure D (Isochromene to Isocoumarin Oxidation using DDQ): To a solution of isochromene (~ 0.5-2 mmol) in 1,4-dioxane (~6-15 mL) at rt was added DDQ (2.0 equiv.) in one portion. The resulting suspension was allowed to stir at rt until TLC analysis indicated consumption of the starting isochromene. To the reaction mixture was added Et2O (20 mL). The reaction mixture was washed with H2O (4x4 mL) and brine (5 mL). The crude isocoumarin was obtained by drying over anhydrous sodium sulfate and concentration under reduced pressure.
Preparation of 6-hydroxy-1H-isochromen-1-one (8): Isochromene 9 (79 mg, 0.5 mmol) was reacted according to general procedure D. Purification using silica gel chromatography (elution with pet ether/EtOAc = 4:1) gave 79 mg (91%) of 8 as a clear colorless oil which was homogeneous by TLC analysis [Rf (8) = 0.23, 2:1, pet ether/EtOAc]: 1H NMR (400 MHz, CHCl3) δ 9.72 (s, 1H), 8.06 (d, J = 8.8 Hz, 1H), 7.37 (d, J = 5.6 Hz, 1H), 7.06 (dd, J = 2.4, 8.4 Hz, 1H), 6.94 (d, J = 2.6 Hz, 1H), 6.57 (d, J = 5.6 Hz, 1H); 13C NMR (100 MHz, CHCl3) δ 163.4, 161.4, 149.9, 139.4, 132.0, 117.6, 114.2, 110.4, 106.8.
General Procedure E (Stork–Danheiser Aldol Reaction6): To a solution of 13 (29 mmol) in THF (50 mL) at -78 °C was added dropwise a solution of LDA (1.8M, 35 mmol). The resulting solution was stirred at -78 °C for 30 min, at which time the corresponding aldehyde (41 mmol) in THF (10 mL) was added dropwise and the reaction mixture was stirred at -78 °C for 1 h. The reaction was quenched by the addition of ammonium chloride (10 mL). Standard extractive workup, followed by silica gel chromatography gave both diastereomers of the aldol adducts. NP indicates the more nonpolar diastereomer and P indicates the more polar diastereomer by silica gel chromatography.
Preparation of 3-ethoxy-6-(1-hydroxyethyl)cyclohex-2-en-1-one (24A): Enone 13 (4.00 g, 29 mmol) was reacted with acetaldehyde according to general procedure E. Purification using silica gel chromatography (elution with pet ether/EtOAc = 1:1) gave 4.7 g (89% combined yield) of 24A as two components as yellow oils which by TLC analysis [Rf (24A-NP) = 0.31, 1:1, pet ether/EtOAc; Rf (24A-P) = 0.22, 1:1, pet ether/EtOAc]: 24A-NP: 1H NMR (400 MHz, CHCl3) δ 5.34 (s, 1H), 5.07 (s, 1H), 3.90-3.95 (m, 2H), 2.28-2.60 (m, 3H) 2.00-2.09 (m, 2H), 1.57-1.61 (m, 1H), 1.33-1.38 (m, 3H), 1.20 (s, 3H); 13C NMR (100 MHz, CHCl3) δ 203.0, 178.4, 102.6, 68.2, 64.5, 51.2, 28.8, 23.5, 20.1, 14.1. 24A-P: 1H NMR (400 MHz, CHCl3) δ 5.36 (s, 1H), 4.18-4.25 (m, 1H), 3.85-3.94 (m, 2H), 3.26 (d, J = 10.1 Hz. 1H), 2.34-2.58 (m, 3H), 1.81-2.02 (m, 2H), 1.38 (t, J = 7.0 Hz, 3H), 1.22 (d, J = 6.6 Hz); 13C NMR (100 MHz, CHCl3) δ 201.8, 178.3, 103.3, 67.1, 64.7, 50.9, 29.1, 22.2, 19.3, 14.3.
Preparation of 3-ethoxy-6-(1-hydroxypropyl)cyclohex-2-en-1-one (24B): Enone 13 (4.00 g, 29 mmol) was reacted with propionaldehyde according to general procedure E. Purification of the crude reaction residue using silica gel chromatography (elution with pet ether/EtOAc = 1:1) gave 5.5 g (97% combined yield) of 24B as two components as yellow oils which by TLC analysis [Rf (24B-NP) = 0.47, 1:1, pet ether/EtOAc; Rf (24B-P) = 0.36, 1:1, pet ether/EtOAc]: 24B-NP: 1H NMR (400 MHz, CHCl3) δ 5.35 (s, 1H), 4.87 (s, 1H), 3.93 (m, 2H), 3.79 (m, 1H), 2.50 (m, 1H), 2.42 (m, 1H), 2.21 (m, 1H), 2.03 (m, 1H), 1.68 (m, 2H), 1.45 (m, 1H), 1.38 (t, J = 7.0 Hz, 3H), 1.00 (t, J = 7.4 Hz, 3H); 13C NMR (100 MHz, CHCl3) δ 203.3, 178.3, 102.5, 72.8, 64.5, 49.1, 28.8, 26.2, 23.4, 14.1, 9.1. 24B-P: 1H NMR (400 MHz, CHCl3) δ 5.35 (d, J = 7.4 Hz, 1H), 4.04-4.14 (m, 1H), 3.85-4.00 (m, 2H), 2.32-2.56 (m, 6H), 1.90-2.04 (m, 2H), 1.37 (t, J = 8.2 Hz, 3H), 1.00 (t, J = 8.0 Hz, 3H); 13C NMR (100 MHz, CHCl3) δ 201.6, 178.1, 103.2, 71.6, 64.5, 50.0, 28.1, 26.2, 21.4, 14.4, 10.9.
Preparation of 3-ethoxy-6-(1-hydroxy-2-methylpropyl)cyclohex-2-en-1-one (24C): Enone 13 (4.00 g, 29 mmol) was reacted with isobutyraldehyde according to general procedure E. Purification using silica gel chromatography (elution with pet ether/EtOAc = 1:1) gave 5.63 g (95% combined yield) of 24C as two components as yellow oils which by TLC analysis [Rf (24C-NP) = 0.61, 1:1, pet ether/EtOAc; Rf (24C-P) = 0.42, 1:1, pet ether/EtOAc]: 24C-NP: 1H NMR (400 MHz, CHCl3) δ 5.33 (s, 1H), 4.73 (s, 1H), 3.85-3.95 (m, 2H), 3.55-3.67 (m, 2H), 2.43-2.41 (m, 1H), 2.31-2.39 (m, 1H), 2.20-2.28 (m, 1H), 1.93-2.00 (m, 1H), 1.73-1.81 (m, 1H), 1.60-1.69 (m, 1H), 1.35 (t, J = 6.2 Hz, 3H), 1.03 (d, J = 6.0, 3H), 1.03 (d, J = 6.0, 3H), 0.89 (d, J = 6.0, 3H); 13C NMR (100 MHz, CHCl3) δ 203.6, 178.0, 102.4, 75.7, 64.4, 47.7, 29.3, 28.6, 23.5, 20.0, 14.3, 14.0. 24C-P: 1H NMR (400 MHz, CHCl3) δ 5.39 (s, 1H), 3.81-3.99 (m, 4H), 2.41-2.53 (m, 1H), 2.37-2.45 (m, 1H), 2.21-2.32 (m, 1H), 1.99-2.09 (m, 2H), 1.67-1.76 (m, 1H), 1.37 (t, J = 6.4 Hz, 3H), 1.06 (d, J = 7.0 Hz, 3H), 0.87 (d, J = 6.7 Hz, 3H); 13C NMR (100 MHz, CHCl3) δ 201.6, 177.9, 103.2, 74.6, 64.4, 48.2, 30.1, 28.8, 20.1, 19.7, 19.1, 14.2.
Preparation of 3-ethoxy-6-(1-hydroxypentyl)cyclohex-2-en-1-one (24D): Enone 13 (4.00 g, 29 mmol) was reacted with pentanal according to general procedure E and silica gel chromatography (elution with pet ether/EtOAc = 1:1) gave 6.1 g (95% combined yield) of 24D as two components as yellow oils which by TLC analysis [Rf (24D-NP) = 0.56, 1:1, pet ether/EtOAc; Rf (24D-P) = 0.47, 1:1, pet ether/EtOAc]: 24D-NP: 1H NMR (400 MHz, CHCl3) δ 5.24 (s, 1H), 4.84 (s, 1H), 3.90-3.96 (m, 2H), 3.83 (bt, 1H), 2.37-2.57 (m, 2H), 2.16-2.22 (m, 1H), 2.00-2.07 (m, 1H), 1.29-1.75 (m, 9H), 0.92 (t, J = 7.1 Hz, 3H); 13C NMR (100 MHz, CHCl3) δ 203.2, 178.2, 102.5, 71.7, 64.5, 49.9, 33.5, 28.8, 27.1, 23.6, 22.8, 14.0, 14.0. 24D-P: 1H NMR (400 MHz, CHCl3) δ 5.37 (s, 2H), 4.16 (bs, 1H), 3.82-3.90 (m, 2H), 2.72 (d, J = 5.9 Hz, 1H, 2.32-2.58 (m, 3H), 1.90-2.03 (m, 2H), 1.48-1.57 (m, 2H), 1.27-1.45 (m, 8H), 0.91 (t, J = 7.1 Hz, 3H); 13C NMR (100 MHz, CHCl3) δ 201.6, 178.2, 103.2, 70.2, 64.5, 50.3, 33.0, 29.0, 28.6, 22.8, 21.3, 14.2, 14.2.
Preparation of 3-ethynyl-4-(1-hydroxyethyl)cyclohex-2-en-1-one (26A): Enone 24A (4.10 g, 22 mmol) was reacted according to general procedure A. Purification using silica gel chromatography (elution with pet ether/EtOAc = 1:1) gave 3.0 g (82%) of 26A as a yellow oil which was homogeneous by TLC analysis [Rf (26A) = 0.32, 1:1, pet ether/EtOAc]: 1H NMR (400 MHz, CHCl3) δ 6.34 (s, 1H), 4.43 (pentet, J = 5.8 Hz, 1H), 3.64 (s, 1H), 2.56-2.71 (m, 2H), 2.37 (dq, J = 17.2, 5.1, 1H), 2.17-2.23 (m, 1H), 1.89-1.96 (m, 2H), 1.25 (d, J = 6.4 Hz, 3H); 13C NMR (100 MHz, CHCl3) δ 198.9, 143.1, 136.0, 89.2, 82.0, 69.3, 45.3, 36.2, 23.0, 19.7.
Preparation of 3-ethynyl-4-(1-hydroxypropyl)cyclohex-2-en-1-one (26B): Enone 24B (3.90 g, 20 mmol) was reacted according to general procedure A. Purification using silica gel chromatography (elution with pet ether/EtOAc = 1:1) gave 3.13 g (89%) of 26B as a yellow oil which was homogeneous by TLC analysis [Rf (26B) = 0.36, 1:1, pet ether/EtOAc]: 1H NMR (400 MHz, CHCl3) δ 6.35 (s, 1H), 4.01-4.12 (m, 1H), 3.62 (s, 1H), 2.59-2.73 (m, 2H), 2.36 (dq, J = 17.2, 5.1, 1H), 2.17-2.27 (m, 1H), 1.87-1.95 (m, 2H), 1.62 (s, 1H), 1.47-1.54 (m, 1H), 1.03 (t, J = 7.3, 3H); 13C NMR (100 MHz, CHCl3) δ 198.9, 143.9, 136.1, 89.0, 82.1, 75.3, 44.7, 36.2, 26.9, 24.0, 11.0.
Preparation of 3-ethynyl-4-(1-hydroxy-2-methylpropyl)cyclohex-2-en-1-one (26C): Enone 24C (4.40 g, 21 mmol) was reacted according to general procedure A. Purification using silica gel chromatography (elution with pet ether/EtOAc = 1:1) gave 3.63 g (91%) of 26C as a yellow oil which was homogeneous by TLC analysis [Rf (26C) = 0.44, 1:1, pet ether/EtOAc]: 1H NMR (400 MHz, CHCl3) δ 6.33 (s, 1H), 3.60-3.69 (m, 2H), 2.57-2.73 (m, 2H), 2.34 (dt, J = 17.6, 6.1 Hz, 1H), 2.11-2.20 (m, 1H), 1.90-1.99 (m, 1H), 0.98 (dd, J = 15.6, 6.7 Hz, 6H); 13C NMR (100 MHz, CHCl3) δ 199.1, 144.1, 135.8, 89.2, 83.3, 79.6, 42.5, 35.2, 31.8, 26.5, 20.1, 17.6.
Preparation of 3-ethynyl-4-(1-hydroxypentyl)cyclohex-2-en-1-one (26D): Enone 24D (4.50 g, 20 mmol) was reacted according to general procedure A. Purification using silica gel chromatography (elution with pet ether/EtOAc = 1:1) gave 3.75 g (92%) of 26D as a yellow oil which was homogeneous by TLC analysis [Rf (26D) = 0.46, 1:1, pet ether/EtOAc]: 1H NMR (400 MHz, CHCl3) δ 6.35 (s, 1H), 4.11-4.22 (m, 1H), 3.62 (s, 1H), 2.57-2.73 (m, 2H), 2.35 (dq, J = 17.1, 5.1 Hz, 1H), 2.20-2.27 (m, 1H), 1.63-1.99 (m, 2H), 1.47-1.55 (m, 2H), 1.31-1.39 (m, 2H), 0.92 (t, J = 6.7 Hz, 3H); 13C NMR (100 MHz, CHCl3) δ 199.2, 143.3, 136.0, 89.2, 82.0, 73.4, 44.9, 36.3, 33.3, 28.8, 23.6, 22.7, 14.2.
Preparation of 1-methyl-8,8a-dihydro-1H-isochromen-6(7H)-one (28A): Enynone 26A (1.80 g, 11 mmol) was reacted according to general procedure B. Purification using silica gel chromatography (elution with pet ether/EtOAc = 4:1) gave 1.4 g (77%) of 28A as a red oil which was homogeneous by TLC analysis [Rf (28A) = 0.33, 2:1, pet ether/EtOAc]: 1H NMR (400 MHz, CHCl3) δ 6.84 (d, J = 5.4 Hz, 1H), 5.71 (s, 1H), 5.53 (d, J = 5.4 Hz, 1H), 3.60-3.67 (m, 1H), 2.51-2.60 (m, 2H), 2.41 (dt, J = 14.7, 4.9 Hz, 1H), 2.05-2.13 (m, 1H), 1.86-1.95 (m, 1H), 1.50-1.73 (m, 2H), 1.05 (t, J =7.3 Hz, 3H); 13C NMR (100 MHz, CHCl3) δ 198.6, 153.8, 153.6, 119.3, 104.8, 81.6, 37.8, 37.4, 25.7, 24.8.
Preparation of 1-ethyl-8,8a-dihydro-1H-isochromen-6(7H)-one (28B): Enynone 26B (2.40 g, 13 mmol) was reacted according to general procedure B. Purification using silica gel chromatography (elution with pet ether/EtOAc = 4:1) gave 2.15 g (89%) of 28B as a red oil which was homogeneous by TLC analysis [Rf (28B) = 0.41, 2:1, pet ether/EtOAc]: 1H NMR (400 MHz, CHCl3) δ 6.81 (d, J = 5.6 Hz, 1H), 5.71 (s, 1H), 5.54 (d, J = 5.6 Hz, 1H), 3.80 (sextet, J = 5.9 Hz, 1H), 2.35-2.60 (m, 2H), 2.10-2.18- (m, 1H), 1.53-1.59 (m, 1H), 1.44 (d, J = 6.2 Hz, 3H); 13C NMR (100 MHz, CHCl3) δ 198.7, 153.4, 153.4, 119.2, 105.0, 77.4, 40.3, 37.4, 25.8, 18.7.
Preparation of 1-isopropyl-8,8a-dihydro-1H-isochromen-6(7H)-one (28C): Enynone 26C (1.70 g, 9 mmol) was reacted according to general procedure B. Purification using silica gel chromatography (elution with pet ether/EtOAc = 4:1) gave 1.17 g (69%) of 28C as a red oil which was homogeneous by TLC analysis [Rf (28C) = 0.49, 2:1, pet ether/EtOAc]: 1H NMR (400 MHz, CHCl3) δ 6.80 (d, J = 5.5 Hz, 1H), 5.71 (s, 1H), 5.50 (d, J = 5.6 Hz, 1H), 3.58 (d, J = 12.2 Hz, 1H), 2.59-2.71 (m, 1H), 2.50-2.59 (m, 1H), 2.41 (dt, J = 14.4, 5.0 Hz, 1H), 2.01-2.10 (m, 2H), 1.56 (dq, J = 12.8, 4.5 Hz, 1H), 1.10 (d, J = 7.0 Hz, 1H), 0.97 (d, J = 7.0 Hz, 1H); 13C NMR (100 MHz, CHCl3) δ 198.6, 154.4, 153.8, 199.2, 104.6, 84.5, 37.4, 36.2, 27.9, 25.3, 20.0, 14.2.
Preparation of 1-butyl-8,8a-dihydro-1H-isochromen-6(7H)-one (28D): Enynone 26D (2.0 g, 10 mmol) was reacted according to general procedure B. Purification using silica gel chromatography (elution with pet ether/EtOAc = 4:1) gave 1.83 g (91%) of 28D as a red oil which was homogeneous by TLC analysis [Rf (28D) = 0.54, 2:1, pet ether/EtOAc]: 1H NMR (400 MHz, CHCl3) δ 6.82 (d, J = 5.6 Hz, 1H), 5.69 (s, 1H), 5.2 (d, J = 5.6 Hz, 1H), 3.62-3.69 (m, 1H), 2.49-2.56 (m, 2H), 2.40 (dt, J = 14.4, 5.0 Hz, 1H), 2.09-2.18 (m, 1H), 1.75-1.83 (m, 1H), 1.52-1.61 (m, 2H), 1.31-1.39 (m, 1H), 0.93 (t, 7.1 Hz, 3H); 13C NMR (100 MHz, CHCl3) δ 198.6, 153.8, 153.5, 119.2, 104.9, 80.7, 38.3, 37.4, 31.6, 26.6, 25.8, 22.8, 14.2.
Preparation of 1-methyl-1H-isochromen-6-ol (29A): Dienone 28A (710 mg, 4.3 mmol) was reacted according to general procedure C. Purification using silica gel chromatography (elution with pet ether/EtOAc = 6:1) gave 631 mg (90%) of 29A as a red oil which was homogeneous by TLC analysis [Rf (29A) = 0.35, 4:1, pet ether/EtOAc]: 1H NMR (400 MHz, CHCl3) δ 6.86 (d, J = 8.1 Hz, 1H), 6.64 (d, J = 8.0 Hz, 1H), 6.54 (d, J = 5.6 Hz, 1H), 6.46 (s, 1H), 5.88 (bs, 1H), 5.69 (d, J = 5.6 Hz, 1H), 5.18 (q, J = 6.4 Hz, 1H), 1.58 (d, J = 6.4 Hz, 3H); 13C NMR (100 MHz, CHCl3) δ 155.6, 145.6, 131.6, 125.2, 124.9, 113.4, 110.3, 105.0, 73.7, 20.0.
Preparation of 1-ethyl-1H-isochromen-6-ol (29B): Dienone 28B (562 mg, 3.2 mmol) was reacted according to general procedure C. Purification using silica gel chromatography (elution with pet ether/EtOAc = 6:1) gave 512 mg (92%) of 29B as a red oil which was homogeneous by TLC analysis [Rf (29B) = 0.38, 4:1, pet ether/EtOAc]: 1H NMR (400 MHz, CHCl3) δ 6.83 (d, J = 8.1 Hz, 1H), 6.62 (dd, J = 2.1, 8.0 Hz, 1H), 6.52 (d, J = 5.7 Hz, 1H), 6.46 (d, J = 2.0 Hz, 1H), 5.65 (d, J = 5.7 Hz, 1H), 5.45 (bs, 1H), 4.97 (dd, J = 5.2, 7.7 Hz, 1H), 2.00 (m, 2H), 1.71-1.80 (m, 2H), 1,01 (t, J = 7.4 Hz, 3H); 13C NMR (100 MHz, CHCl3) δ 155.5, 145.1, 131.5, 125.6, 124.1, 113.1, 110.3, 104.4, 78.8, 27.4, 10.1.
Preparation of 1-isopropyl-1H-isochromen-6-ol (29C): Dienone 28C (450 mg, 2.3 mmol) was reacted according to general procedure C. Purification using silica gel chromatography (elution with pet ether/EtOAc = 6:1) gave 303 mg (69%) of 29C as a red oil which was homogeneous by TLC analysis [Rf (29C) = 0.41, 4:1, pet ether/EtOAc]: 1H NMR (400 MHz, CHCl3) δ 6.80 (d, J = 8.1 Hz, 1H), 6.61 (dd, J = 2.1, 8.0 Hz, 1H), 6.53 (d, J = 5.7 Hz, 1H), 6.44 (d, J = 2.0 Hz, 1H), 5.61 (d, J = 5.7 Hz, 1H), 5.53 (bs, 1H), 4.74 (d, J = 6.8 Hz, 1H), 2.21 (sextet, J = 6.7 Hz, 1H), 1.03 (d, J = 6.6 Hz, 3H), 0.89 (d, J = 6.6 Hz, 3H); 13C NMR (100 MHz, CHCl3) δ 155.4, 145.3, 131.6, 126.9, 122.8, 112.9, 110.2, 104.3, 82.9, 31.9, 19.2, 18.3.
Preparation of 1-butyl-1H-isochromen-6-ol (29D): Dienone 28D (733 mg, 3.6 mmol) was reacted according to general procedure C. Purification using silica gel chromatography (elution with pet ether/EtOAc = 6:1) gave 662 mg (91%) of 29D as a red oil which was homogeneous by TLC analysis [Rf (29D) = 0.41, 4:1, pet ether/EtOAc]: 1H NMR (400 MHz, CHCl3) δ 6.84 (d, J = 8.0 Hz, 1H), 6.62 (dd, J = 2.0, 8.1 Hz, 1H), 6.51 (d, J = 5.6 Hz, 1H), 6.46 (d, J = 2.1 Hz, 1H), 5.67 (d, J = 5.7 Hz, 1H), 5.44 (bs, 1H), 5.05 (dd, J = 4.9, 8.2 Hz, 1H), 4.16 (q, J = 7.1 Hz, 1H), 1.92-2.01 (m, 1H), 1.66-1.73 (m, 1H), 1.45-1.50 (m, 1H), 1.33-1.41 (m, 2H), 1.26-1.32 (m, 1H), 0.93 (t, J = 7.0 Hz, 3H); 13C NMR (100 MHz, CHCl3) δ 155.4, 145.1, 131.4, 125.5, 124.4, 113.2, 110.3, 104.5, 76.9, 34.1, 27.8, 22.8, 14.3.
Preparation of 3-(hex-1-yn-1-yl)-4-(hydroxymethyl)cyclohex-2-en-1-one (30A): To a solution of 1-hexyne (5.6 mL, 48 mmol) in 75 mL of THF at -78 °C was added n-butyllithium (17.0 mL, 2.5 M, ~43 mmol) over a 2-min period. The resulting mixture was stirred at -78 °C for 30 min, and then warmed to 0 °C over a 30 min period. To the resulting solution was added a solution of enone-alcohol 12 (3.30 g, 19 mmol) dissolved in 20 mL of THF via cannulation over a 5-min period. The resulting reaction mixture was stirred at 0 °C for 1 h. The reaction mixture was quenched by the addition of water (20 mL), followed by the portion-wise addition of aqueous 6M HCl (50 mL). After warming the resulting solution to rt, and stirring for 30 min, the resulting solution was subjected to standard extractive workup. Silica gel chromatography (elution with pet ether/EtOAc = 1:1) gave 3.2 g (80%) of 30A as a yellow oil which was homogeneous by TLC analysis [Rf (30A) = 0.30, 1:1, pet ether/EtOAc]: 1H NMR (400 MHz, CHCl3) δ 6.19 (s, 1H), 3.82-3.91 (m, 3H), 2.50-2.59 (m, 2H), 2.33-2.41 (m, 3H), 1.95-2.19 (m, 2H), 1.50-1.59 (m, 2H), 1.33-1.41 (m, 2H), 0.91 (t, J = 7.0 Hz, 3H); 13C NMR (100 MHz, CHCl3) δ 199.5, 145.5, 133.4, 104.2, 79.4, 63.7, 42.1, 35.4, 30.5, 24.9, 22.1, 19.7, 13.7.
Preparation of 4-(hydroxymethyl)-3-(phenylethynyl)cyclohex-2-en-1-one (30B): To a solution of phenylacetylene (5.2 mL, 47 mmol) in 75 mL of THF at -78 °C was added n-butyllithium (16.5 mL, 2.5M, ~42 mmol) over a 2-min period. The resulting mixture was stirred at -78 °C for 30 min, and then warmed to 0 °C over a 30-min period. To the resulting solution was added a solution of enone 12 (3.20 g, 19 mmol) dissolved in 20 mL of THF via cannulation over a 5-min period. The resulting reaction mixture was stirred at 0 °C for 1 h. The reaction mixture was quenched by the addition of water (20 mL), followed by the portion-wise addition of aqueous 6M HCl (50 mL). After warming the resulting solution to rt, and stirring for 30-min, the resulting solution was subjected to standard extractive workup. Silica gel chromatography (elution with pet ether/EtOAc = 1:1) gave 3.0 g (72%) of 30B as a yellow oil which was homogeneous by TLC analysis [Rf (30B) = 0.42, 1:1, pet ether/EtOAc]: 1H NMR (400 MHz, CHCl3) δ 7.43-7.49 (m, 2H), 7.30-7.39 (m, 3H), 6.33 (s, 1H), 3.89-4.04 (m, 2H), 2.53-2.85 (m, 3H), 2.35-2.43 (m, 1H), 2.09-2.16 (m, 2H); 13C NMR (100 MHz, CHCl3) δ 199.1, 144.2, 134.1, 132.2, 129.9, 128.8, 121.9, 101.5, 87.5, 64.0, 41.9, 35.7, 25.2.
Preparation of Dihydropyran 31A. Enynone 30A (752 mg, 3.7 mmol) was reacted according to general procedure B. Purification using silica gel chromatography (elution with pet ether/EtOAc = 4:1) gave 660 mg (88%) of 31A as a red oil which was homogeneous by TLC analysis [Rf (31A) = 0.44, 2:1, pet ether/EtOAc]: 1H NMR (400 MHz, CHCl3) δ 5.63 (s, 1H), 5.41 (s, 1H), 4.32 (dd, J = 10.7, 5.4 Hz, 1H), 3.61 (dd, J = 13.3, 11.0 Hz, 1H), 2.75 (m, 1H), 2.48-2.55 (m, 1H), 2.37-2.45 (m, 1H), 2.19 (t, J = 7.1 Hz, 2H), 2.05-2.13 (m, 1H), 1.47-1.55 (m, 3H), 1.33 (sextet, J = 7.5 Hz, 2H), 0.90 (t, J = 7.3 Hz, 3H); 13C NMR (100 MHz, CHCl3) δ 198.7, 167.0, 154.9, 117.3, 101.0, 70.7, 37.2, 34.4, 33.7, 29.0, 25.2, 22.4, 14.0.
Preparation of Dihydropyran 31B. Enynone 30B (890 mg, 3.9 mmol) was reacted according to general procedure B. Purification using silica gel chromatography (elution with pet ether/EtOAc = 4:1) gave 540 mg (61%) of 31B as a red oil which was homogeneous by TLC analysis [Rf (31B) = 0.42, 2:1, pet ether/EtOAc]: 1H NMR (400 MHz, CHCl3) δ 7.63-7.76 (m, 2H), 7.38-7.46 (m, 3H), 6.18 (s, 1H), 5.84 (s, 1H), 4.55 (dd, J = 10.7, 5.3 Hz, 1H), 3.61 (dd, J = 13.0, 11.8 Hz, 1H), 2.84-2.91 (m, 1H), 2.55-2.64 (m, 1H), 2.48 (dt, J = 14.3, 4.9 Hz, 1H), 2.01-2.12 (m, 1H), 1.64 (dt, J = 13.4, 4.0 Hz, 1H); 13C NMR (100 MHz, CHCl3) δ 198.7, 160.4, 154.5, 133.7, 130.6, 128.8, 126.0, 119.3, 100.7, 71.0, 37.3, 34.1, 25.3.
Preparation of 3-butyl-1H-isochromen-6-ol (32A): Dienone 31A (415 mg, 2.0 mmol) was reacted according to general procedure C. Purification using silica gel chromatography (elution with pet ether/EtOAc = 6:1) gave 342 mg (82%) of 32A as a clear colorless oil which was homogeneous by TLC analysis [Rf (32A) = 0.45, 4:1, pet ether/EtOAc]: 1H NMR (400 MHz, CHCl3) δ 6.87 (d, J = 6.9 Hz, 1H), 6.56 (d, J = 6.9 Hz, 1H), 6.42 (s, 1H), 5.58 (s, 1H), 4.98 (s, 2H), 4.81 (bs, 1H), 2.19 (t, J = 7.6 Hz, 2H), 1.50-1.58 (m, 2H), 1.31-1.40 (m, 2H), 0.93 (t, J = 7.2 Hz, 3H); 13C NMR (100 MHz, CHCl3) δ 159.8, 155.7, 133.9, 125.0, 120.0, 112.2, 109.5, 100.9, 68.7, 33.6, 29.4, 22.5, 14.1.
Preparation of 3-phenyl-1H-isochromen-6-ol (32B): Dienone 31B (620 mg, 2.7 mmol) was reacted according to general procedure C. Purification using silica gel chromatography (elution with pet ether/EtOAc = 6:1) gave 569 mg (93%) of 32B as a clear colorless oil which was homogeneous by TLC analysis [Rf (32B) = 0.39, 4:1, pet ether/EtOAc]: 1H NMR (400 MHz, CHCl3) δ 7.73 (d, J = 7.1 Hz, 2H), 7.31-7.40 (m, 3H), 6.95 (d, J = 7.8 Hz, 1H), 6.64 (d, J = 7.8 Hz, 1H), 6.60 (s, 1H), 6.39 (s, 1H), 5.17 (s, 2H), 4.81 (bs, 1H); 13C NMR (100 MHz, CHCl3) δ 155.9, 154.7, 134.4, 133.7, 130.4, 129.2, 128.6, 125.4, 125.1, 120.7, 113.2, 110.6, 101.2, 69.0.
Preparation of 3-butyl-6-hydroxy-1H-isochromen-1-one (33A): Isochromene 32A (109 mg, 0.5 mmol) was reacted according to general procedure D. Purification using silica gel chromatography (elution with pet ether/EtOAc = 4:1) gave 83 mg (71%) of isocoumarin 33A as a clear colorless oil which was homogeneous by TLC analysis [Rf (33A) = 0.50, 4:1, pet ether/EtOAc]: 1H NMR (400 MHz, CHCl3) δ 8.13 (d, J = 8.7 Hz, 1H), 8.00 (bs, 1H), 7.02 (dd, J = 1.7, 8.7 Hz, 1H), 6.82 (d, J = 1.9 Hz, 1H), 6.20 (s, 1H), 2.50 (t, J = 7.6 Hz, 2H), 1.65 (pentet, J = 7.6 Hz, 2H), 1.37 (pentet, J = 7.5 Hz, 2H), 0.92 (t, J = 7.3 Hz, 3H); 13C NMR (100 MHz, CHCl3) δ 164.3, 162.8, 158.9, 140.6, 132.3, 117.2, 112.7, 110.0, 103.5, 33.3, 29.1, 22.3, 14.0.
Preparation of 3-phenyl-6-hydroxy-1H-isochromen-1-one (33B): Isochromene 32B (160 mg, 0.7 mmol) was reacted according to general procedure D. Purification using silica gel chromatography (elution with pet ether/EtOAc = 4:1) gave 134 mg (79%) of isocoumarin 33B as a clear colorless oil which was homogeneous by TLC analysis [Rf (33B) = 0.44, 4:1, pet ether/EtOAc]: 1H NMR (400 MHz, CH3OH) δ 8.06 (d, J = 8.7 Hz, 1H), 7.86 (d, J = 7.5 Hz, 2H), 7.40-7.50 (m, 3H), 7.10 (s, 1H), 6.96 (d, J = 8.8 Hz, 1H), 6.91 (s, 1H); 13C NMR (100 MHz, CH3OH) δ 164.2, 163.1, 153.7, 140.5, 132.2, 131.6, 129.8, 128.8, 125.0, 117.4, 112.0, 110.5, 102.1.
ACKNOWLEDGEMENTS
We thank the National Science Foundation (CHE-0506486) for support of this research.
References
1. H. A. Bruson and J. W. Kroeger, J. Am. Chem. Soc., 1940, 62, 36. CrossRef
2. G. Majetich, G. Zou, and J. L. Grove, Org. Lett., 2008, 10, 81. CrossRef
3. G. Majetich and J. L. Grove, Org. Lett., 2009, 11, 2904. CrossRef
4. (a) S. M. Daly and R. W. Armstrong, Tetrahedron Lett., 1989, 30, 5713; CrossRef (b) M. Ishizaki, O. Hoshino, and Y. Iitaka, Tetrahedron Lett., 1991, 32, 7079. CrossRef
5. For other applications of this A + C →ABC cyclialkylation strategy, see: (a) G. Majetich, Y. Zhang, T. L. Feltman, and S. G. Duncan, Jr., Tetrahedron Lett., 1993, 34, 445; CrossRef (b) G. Majetich and G. Zou, Org. Lett., 2008, 10, 85; CrossRef (c) G. Majetich, Y. Li, and G. Zou, Heterocycles, 2007, 73, 217; CrossRef (d) G. Majetich, J. Yu, and Y. Li, Heterocycles, 2007, 73, 227. CrossRef
6. G. Stork and R. L. Danheiser, J. Org. Chem., 1973, 38, 1775. CrossRef
7. (a) H. Hong, N. Neamati, H. E. Winslow, J. L. Christensen, A. Orr, Y. Pommier, and G. W. A. Milne, Antiviral Chemistry & Chemotherapy, 1998, 9, 461; (b) J. Gayosso-De-Lucio, M. Torres-Valencia, A. Rojo-Domínguez, H. Nájera-Peña, B. Aguirre-López, J. Salas-Pacheco, C. Avitia-Domínguez, and A. Téllez-Valencia, Bioorg. Med. Chem. Lett., 2009, 19, 5936; CrossRef (c) I. Lee and M. Yang, Arch. Pharmacal. Res., 1994, 17, 476; CrossRef (d) H. Lim, H. Kim, H. Choi, S. Oh, and J. Choi, J. Ethnopharmacol., 2000, 72, 469; CrossRef (e) H. Pu, X. Huang, J. Zhao, and A. Hong, Planta Med., 2002, 68, 372; CrossRef (f) S. Huneck, K. Schreiber, G. Snatzke, and H. W. Fehlhaber, Zeit. Fuer. Naturforschung, Teil B., 1970, 25, 49; (g) T. Furuta, Y. Fukuyama, and Y. Asakawa, Phytochem., 1986, 25, 517; CrossRef (h) A. Badar-ud-Din, R. Hafiz, H. Nasim, M. A. Malana, and G. Qadeer, Ind. J. Chem., B., 2006, 45B, 820; (i) J. Grimshaw and R. D. Haworth, J. Chem. Soc., 1956, 418; CrossRef (j) J. Herzig and R. Wachsler, Monatsh. Chem., 1914, 35, 77; CrossRef (k) A. J. Birch, J. H. Birkinshaw, P. Chaplen, L. Mo, A. H. Manchanda, A. Pelter, and M. Riano-Martin, Aust. J. Chem., 1969, 22, 1933. CrossRef
8. For reviews, see: (a) R. Barry, Chem. Rev., 1964, 64, 229; CrossRef (b) N. Narasimhan and R. Mali, Synthesis, 1983, 957; CrossRef (c) R. Hill, Prog. Chem. Org. Nat. Prod., 1986, 49, 1; (d) E. Napolitano, Org. Prep. Proc. Int., 1997, 29, 631. CrossRef
9. D. Korte, L. Hegedus, and R. Wirth, J. Org. Chem., 1977, 42, 1329. CrossRef
10. For a comprehensive review, see: V. Snieckus, Chem. Rev., 1990, 90, 879. CrossRef
11. R. Larock, S. Varaprath, H. Lau, and C. Fellows, J. Am. Chem. Soc., 1984, 106, 5274. CrossRef
12. N. Patil and Y. Yamamoto, J. Org. Chem., 2004, 69, 5139. CrossRef
13. P. A. Grieco and K. Hiroi, J. Chem. Soc., Chem. Commun., 1972, 1317. CrossRef
14. (a) Y. Nakadaira, J. Hayashi, H. Sato, and K. Nakanishi, J. Chem. Soc., Chem. Commun., 1972, 5, 282; CrossRef (b) T. A. Bryson and R. B. Gammill, Tetrahedron Lett., 1974, 45, 3963. CrossRef
15. H. Reich, J. Renga, and I. Reich, J. Am. Chem. Soc., 1975, 97, 5434. CrossRef
16. For the oxidation of isochromans to isochroman-1-ones, see: (a) A. Shaabani, P. Mirzaei, S. Naderi, and D. Lee, Tetrahedron, 2004, 60, 11415; CrossRef (b) N. Noureldin, D. Zhao, and D. Lee, J. Org. Chem., 1997, 62, 8767; CrossRef (c) M. Reetz and K. Töllner, Tetrahedron Lett., 1995, 36, 9461; CrossRef (d) Y. Bonvin, E. Callens, I. Larrosa, D. Henderson, J. Oldham, A. Burton, and A. Barrett, Org. Lett., 2005, 7, 4549; CrossRef (e) S. Silvestre and J. Salvador, Tetrahedron, 2007, 63, 2439; CrossRef (f) S. Yamazaki, Org. Lett., 1999, 1, 2129; CrossRef (g) F. Bonadies, R. Di Fabio, and C. Bonini, J. Org. Chem., 1984, 49, 1647. CrossRef
17. (a) L. Liu and P. Floreancig, Org. Lett., 2009, 11, 3152; CrossRef (b) W. Tu, L. Liu, and P. E. Floreancig, Angew. Chem., Int. Ed., 2008, 47, 4184; CrossRef (c) W. Tu and P. E. Floreancig, Angew. Chem., Int. Ed., 2009, 48, 4567. CrossRef
18. (a) Y.-C. Xu, E. Lebeau, J. W. Gillard, and G. Attardo, Tetrahedron Lett., 1993, 34, 3841; CrossRef (b) Y.-C. Xu, C. Roy, and E. Lebeau, Tetrahedron Lett., 1993, 34, 8189; CrossRef (c) Y.-C. Xu, E. Lebeau, P. L. Myers, and J. W. Gillard, J. Org. Chem., 1994, 59, 4868; CrossRef (d) W. Wang, T. Li, and G. Attardo, J. Org. Chem., 1997, 62, 6598. CrossRef
19. (a) D. Walker and J. D. Hiebert, Chem. Rev., 1963, 67, 153; CrossRef (b) J. N. Marx and Y.-S. P. Hahn, J. Org. Chem., 1988, 53, 2866; CrossRef (c) A. Begouin, S. Hesse, and G. Kirsch, ARKIVOC, 2008, 7, 84; (d) M. S. Islam, K. Ishigami, and H. Watanabe, Tetrahedron, 2007, 63, 1074; CrossRef (e) E.-C. Wang, Y.-S. Wein, and Y.-H. Kuo, Tetrahedron Lett., 2006, 47, 9195; CrossRef (f) V. K. Ahluwaliar, S. Jolly, and A. K. Tehim, Tetrahedron, 1982, 38, 3673; CrossRef (g) L. Y. Chen, S. R. Li, P. Y. Chen, H. C. Chang, T. P. Wang, I. L. Tsai, and E. C. Wanga, ARKIVOC, 2010, 11, 64.
20. G. Majetich and S. Allen, ARKIVOC, 2010, 11, 104.