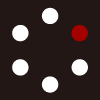
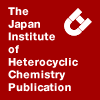
HETEROCYCLES
An International Journal for Reviews and Communications in Heterocyclic ChemistryWeb Edition ISSN: 1881-0942
Published online by The Japan Institute of Heterocyclic Chemistry
e-Journal
Full Text HTML
Received, 22nd July, 2011, Accepted, 29th September, 2011, Published online, 6th October, 2011.
DOI: 10.3987/COM-11-12313
■ Voltammetric Behavior of Mediator-Modified Electrode by Electrochemical Copolymerization of Nitroxyl Radical Precursor Containing Pyrrole Side Chain and Thiophenes
Yoshitomo Kashiwagi,* Makiko Tsunoda, and Tetsuya Ono
School of Pharmaceutical Sciences, Ohu University, 31-1 Misumido, Tomita-machi, Koriyama, Fukushima 963-8611, Japan
Abstract
This paper describes the voltammetric behavior of the TEMPO (2,2,6,6-tetramethylpiperidine-1-oxyl)-modified electrodes prepared by the electrochemical copolymerization of TEMPO precursor containing pyrrole side chain and thiophenes. The prepared TEMPO-modified electrode with 2,2’-bithiophene exhibits a symmetrical reversible redox wave at +0.7 V versus Ag/AgCl, which originates from the electron transfer between the pyrrole and 2,2’-bithiophene-copolymerized layer and the electrode. The electrochemical copolymerization using 2,2’-bithiophene showed a nonlinear behavior between the anodic peak current in the cyclic voltammogram of the copolymers-modified electrode and the fraction of TEMPO precursor containing pyrrole side chain in solution. The copolymers-modified electrode exhibits high electrocatalytic activity for the oxidation of alcohol.INTRODUCTION
Conductive polypyrrole and polythiophene are normally obtained via chemical and electrochemical oxidative polymerization of pyrrole and thiophene, respectively.1-3 The electrochemical polymerization method provides conducting polymers in the form of films adhered to the electrode surfaces. There are several reports regarding the preparation of a nitroxyl-radical-modified electrode by electrochemical polymerization of nitroxyl radical precursor containing pyrrple side chain.4,5 Recently, we have demonstrated that the possibility of electrochemical generation of polymer film supports on the electrode surface with TEMPO (2,2,6,6-tetrametylpiperidine-1-oxyl) sites attached to the polymer chains.6 On the other hand, Siu et al. reported the generation of polymeric TEMPO catalysts on the electrode surface by the electrochemical copolymerization of 2,2’-bithiophene with TEMPO catalyst precursors containing pyrrole side chains.5 However, the voltammetric behavior of the prepared TEMPO-modified electrodes has not been investigated in detail. We report here the voltammetric behavior of TEMPO-modified electrode prepared by the electrochemical copolymerization of 4-(3-(pyrrole-1-yl)propoxycarbonyl)-TEMPO (1) and thiophenes such as thiophene (2), 2,2’-bithiophene (3), and 2,2’:5’,2’’-terthiophene (4). It may be possible to modulate the conducting copolymer by varying the monomer feed ratio7-9 and the applied polymerization potential.8-10
RESULTS AND DISCUSSION
Electrochemistry is at the interface of solution and solid-phase chemistry, as reactions occur in the diffusion layer formed at the electrode surface.11 Our studies commenced with the generation of suitable conducting polymer precursors for heterogeneous TEMPO oxidation catalyst on the electrode surface.6 Direct electrochemical polymerization of monomer 1 on a platinum electrode in MeCN produced a film. The polymer 1 on the electrode surface was considerably stable, and no deactivation was observed in the cyclic voltammograms(CVs) after repeated potential scanning. The peak current of the CVs increased with an increase in the number of the scans, and it reached its peak at the fourth scan. To increase the density of electroactive 1 at the surface of electrode, we turned to copolymerization with thiophenes such as thiophene, 2,2’-bithiophene, and 2,2’:5’,2’’-terthiophene. The 2,2’-bithiophene is known for its ability to initiate electrochemical polymerizations due to its low oxidation potential (+1.3 V vs SCE).12
1. Electrochemical Copolymerization of 4-(3-(Pyrrole-1-yl)propoxycarbonyl)-TEMPO (1) and Thiophenes
We have reported that the poly-1-modified electrode can be effectively provided by repetitive cyclic voltammetric scans in the solution of 0.01 M monomer 1.6 Based on these results, electrochemical copolymerization of monomer 1 and thiophenes was performed in MeCN solution with 0.01 M total monomer concentration and 0.1 M TBAP as the supporting electrolyte. Figure 1 shows the CVs of the 1:1 mixtures of monomer 1/thiophenes. The anodic and the cathodic peak currents of the CV were not increased in the mixture of monomers 1 and 2 (Figure 1 (a)). This means that monomers 1 and 2 could not be copolymerized electrochemically because the oxidation potential of monomer 2 at + 2.0 V vs. SCE can be observed. On the other hand, in the CV of the mixture of monomers 1 and 3 (Figure 1 (b)) the reversible oxidation of a nitroxyl radical/oxoammonium ion redox system at +0.7 V and irreversible oxidation of monomer 3 at + 1.3 V were observed. The oxidation potential of monomer 3 and the pyrrole moiety of monomer 1 are very near. The re-reduction peak current of oxoammonium ion is larger than the oxidation peak current of nitroxyl radical. These suggest that while monomers 1 and 3 could be copolymerized electrochemically. Therefore, we prepared copolymers-1-3-modified electrode through the direct electrochemical copolymerizaition of monomers 1 and 3 on the electrode surface by applying the potential above + 1.3 V. The CV of the mixture of monomers 1 and 4 (Figure 1 (C)) showed the results similar to the CV of the mixture of monomers 1 and 3. However, the oxidation potential of monomer 4 exhibits an irreversible wave at + 1.0 V.
In the case of electrochemical copolymerization of monomer 3 with monomer 1, we have observed an interesting nonlinear behavior between the anodic peak current in the CV of the copolymers-1-3-modified electrode and the fraction of monomer 1 in solution (Figure 2). This electrochemical coplymerization behavior suggests an inhibiting effect of monomer 1 and an initiating/promoting effect of monomer 3. At higher monomer 3/monomer 1 feed ratios, the initiating/promoting effect dominates and the anodic peak current in the CV of the copolymers-1-3-modified electrode correlates with the monomer 1/monomer 3 ratio.5 When more monomer 1 is present in solution, its inhibiting effect becomes predominant, and the anodic peak current decreases with increasing monomer 1/monomer 3 feed ratio. The 2:1 feed ratio of monomer 1/monomer 3 corresponds to the largest anodic peak current in the CV of the copolymers-1-3-modified electrode. Similar nonlinear behavior between the anodic peak current in the CV and the fraction of monomer 1 in solution was observed in the electrochemical copolymerization of monomer 4 with monomer 1 (Figure 2). However, the 5:1 feed ratio of monomer 1/monomer 4 corresponds to the largest anodic peak current in CV of the copolymers-1-4-modified electrode. Figure 3 shows the CV of the copolymers-1-3-modified electrode, in which a reversible redox couple can be observed. This redox couple corresponds to the one electron oxidation of nitroxyl radical to oxoammonium ion. The immobilized 1 on the electrode surface was quite stable and no deactivation was observed in the CV after repeated potential scanning. The peak split between the oxidation and reduction potentials became wide as the potential scan rate was sped up (Figure 3). A linear relationship between the anodic peak current and the potential scan rate was observed within the scan rate range from 10 to 100 mV s-1 (Figure 4).11 The amount of electroactive 1 on the electrode surface was determined to be ca. 2.2 × 10-8 mol cm-2 by integrating the nitroxyl-radical oxidation current of the CV and applying Faraday’s law. Therefore, the copolymers-1-3-modified electrode can be effectively provided by repetitive cyclic voltammetric scans between +0.4 and +1.4 V with the scan rate of 50 mV s-1 in the solution of monomer 1/monomer 3 with the feed ratio of 2:1.
2. Electrocatalytic Activity for the Oxidation of Geraniol
Cyclic voltammetry was used for checking whether the electron transfer from the immobilized oxoammonium ion of the nitroxyl radical on the electrode surface to dissolved geraniol occurred. The CVs of the copolymers-1-3-modified electrode in the presence of geraniol and 2,6-lutidine are shown in Figure 5. The anodic peak current for geraniol was significantly enhanced in comparison with the blank voltammogram (the copolymers-1-3-modified electrode itself). The oxidation peak current was proportional to the concentration of geraniol (Figure 6). The ratio of the new oxidation peak height to the reversible nitroxide oxidation peak height decreased with an increase in the scan rate. This shows that the immobilized nitroxyl radical on the copolymers-1-3-modified electrode is electrocatalytically active for the oxidation of geraniol because geraniol is not electroactive below +1.0 V versus Ag/AgCl. All these results are characteristic of the electrochemical catalysis of the oxidation of geraniol to geranial via active oxoammonium ion oxidant.13,14 A linear relationship between the anodic peak current and the concentration of geraniol from 1 to 100 mM was observed (Figure 7). This suggests that the present system may also be applicable to the quantitative analysis of geraniol. The copolymers-1-3-modified electrode as a functions of the concentration of geraniol gave larger anodic peak current in the CVs than the polymer-1-modified electrode (Figure 7). This means that the copolymers-1-3-modified electrode has higher geraniol sensitivity than the polymer-1-modified electrode.
In summary, the nitroxyl-radical-modified electrode prepared by the electrochemical copolymerization of nitroxyl radical precursor containing pyrrole side chain with 2,2’-bithiophene or 2,2’:5’,2’’-terthiophehe exhibited a symmetrical reversible redox wave in the CVs. The properties of the immobilized copolymers on the electrode surface exhibited a nonlinear behavior between the anodic peak current in CV of the copolymer-modified electrode and the fraction of TEMPO precursor containing pyrrole side chain in solution. Furthermore, this copolymers-modified electrode exhibited high electrocatalytic activity for the oxidation of geraniol. We are now studying the electrocatalytic oxidation of alcohols on this copolymers-modified electrode.
EXPERIMENTAL
1. General Information
4-(3-(Pyrrole-1-yl)propoxycarbonyl)-TEMPO (1) was prepared by the procedure of a previous report.6,15 Thiophene, 2,2’-bithophene, and 2,2’:5’,2’’-terthiophene were purchased from Tokyo Chemical Industry Co., Ltd. Tetra-n-butylammonium perchlorate (TBAP) was purchased from Nacalai Tesque, Inc. Acetonitrile (MeCN) was purified by using the method described in the literature.16 All the other reagents used in this study were of the commercially available reagent grade. The electrochemical copolymerization and the cyclic voltammetry characterization were conducted on a BAS ALS/CHi420A.
2. General Procedure for Electrochemical Copolymerization
Electrochemical polymerization and characterizations were performed in a one-compartment cell with a platinum disk (0.07 cm2) as working electrode and a platinum wire as counter electrode. A Ag/AgCl (0.1 M (C4H9)4NClO4 (TBAP) in MeCN) electrode was used as reference electrode. All electrochemical copolymerization experiments were performed in MeCN solution with 0.01 M total monomer concentration and 0.1 M TBAP as the supporting electrolyte. Repetitive cyclic voltammetric scans between +0.4 and +1.4V with the scan rate of 50 mV s-1 led to the formation of a black electroactive film at the working electrode. Characterizations (by cyclic voltammetry) of the copolymer film were conducted in MeCN solutions with 0.1 M TBAP.
3. Electrocatalytic Activity for Oxidation of Geraniol
The electrocatalytic activity of the copolymers-1-bithiophene-modified electrode for the oxidation of geraniol was evaluated in a one-compartment cell with a platinum wire and a Ag/AgCl electrode. The electrolyte was 10 mL of MeCN solution containing geraniol as the substrate, 2,6-lutidine as the base, and 0.1 M TBAP.
ACKNOWLEDGEMENTS
This work was supported in part by a Grant-in-Aid for Young Scientists B (No. 21790040) from the Ministry of Education, Culture, Sports, Science and Technology of Japan and a Grant-in-Aid for Scientific Research C (No. 23590195) from the Japan Society for Promotion of Science. The Foundation for Japanese Chemical Research and Ohu University Joint Research Fund are also acknowledged for financial support.
References
1. L. F. Warren and D. P. Anderson, J. Electrochem. Soc., 1987, 134, 101. CrossRef
2. S. P. Armes, Synth. Met., 1987, 20, 365. CrossRef
3. J. Roncali, J. Mater. Chem., 1999, 9, 1875. CrossRef
4. A. Derozier, D. Limosin, and J.-C. Moutet, Electrochim. Acta, 1987, 32, 1643. CrossRef
5. T. Siu, S. Yekta, and A. K. Yudin, J. Am. Chem. Soc., 2000, 122, 11787. CrossRef
6. T. Ono, M. Tsunoda, S. Takahashi, T. Obata, T. Sone, and Y. Kashiwagi, Heterocycles, 2010, 81, 2771. CrossRef
7. A. Bagheri, M. R. Nateghi, and A. Massoumi, Synth. Met., 1998, 97, 85. CrossRef
8. H.-P. Welzel, G. Kossmehl, G. Engelmann, W.-D. Hunnius, and W. Plieth, Electrochim. Acta, 1999, 44, 1827. CrossRef
9. X. Wan, W. Zhang, S. Jin, G. Xue, Q.-D. You, and B. Che, J. Electroanal. Chem., 1999, 470, 23. CrossRef
10. R.-M. Latonen, C. Kvarnstrom, and A. Ivaska, Electrochim. Acta, 1999, 44, 1933. CrossRef
11. A. J. Bard and L. R. Faulkner, Electrochemical Methods: Fundamentals and Applications, John Wiley & Sons, Inc, New York, 1980.
12. E. M. Peters and J. D. van Dyke, J. Polym. Sci. A: Polym. Chem., 1991, 29, 1379. CrossRef
13. R. W. Murray, in “Electroanalytical Chemistry”, ed. by A. J. Bard, vol. 13, Marcel Dekker, New York, 1984, p. 191.
14. R. W. Murray, in “Molecular Design of Electrode Surface”, ed. by R. W. Murray, in “Techniques of Chemistry”, Series 22, Wiley-Interscience, New York, 1992.
15. R. C. Blume and H. G. Lindwall, J. Org. Chem., 1945, 10, 255. CrossRef
16. J. A. Riddick and W. B. Bunger, “Techniques of Chemistry Vol. II, Organic Solvent”, John Wiley & Sons, Inc., New York, 1970.