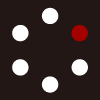
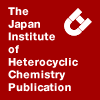
HETEROCYCLES
An International Journal for Reviews and Communications in Heterocyclic ChemistryWeb Edition ISSN: 1881-0942
Published online by The Japan Institute of Heterocyclic Chemistry
e-Journal
Full Text HTML
Received, 3rd August, 2011, Accepted, 5th September, 2011, Published online, 14th September, 2011.
DOI: 10.3987/COM-11-12326
■ Microwave-Assisted Synthesis and Bioactivity of Novel 2,2,4,5-Tetrasubstituted 3-Dichloroacetyl-1,3-oxazolidines
Ying Fu, Lei Yang, Fei Ye,* and Shuang Gao
Department of Applied Chemistry, College of Sciences, Northwest Agricultural University, Harbin 150030, Heilongjiang, China
Abstract
An efficient one-pot synthesis of 2,2,4,5-tetrasubstituted-3-dichloroacetyl-1,3-oxazolidines under microwave irradiation was developed. The intermediate oxazolidines were attained by the reaction of amino alcohol with aldehyde or ketone in refluxing benzene with microwave-assisted cycloaddition, and then the acylation followed with dichloroacetyl chloride and NaOH acting as the attaching acid agent. All the compounds were characterized by IR, 1H-NMR, 13C-NMR and elemental analysis. The preliminary biological test shows that these compounds could protect maize against injury caused by acetochlor to a certain extent.In recent years, oxazolidine derivatives are important target compounds because they are found to possess useful biological activities and pharmacological activities.1 They are also utilized as chiral synthons in asymmetric syntheses of biological active compounds or their synthetic intermediates.2 In particular, 3-dichloroacetyl oxazolidine derivatives display significant herbicide safener properties.3
Recently, some papers were published on the synthesis of oxazolidines. A general synthetic method for the synthesis of 1,3-oxazolidines was the condensation of β-amino alcohols with carbonyl compounds in the presence of an acid catalyst.4 Several other catalytic methods using transition metals as catalysts have been developed. For example, Yamamoto and Shim showed the Pd-catalyzed [3+2] cycloaddition of vinylic oxiranes with imines,5 and the reaction of β-amino alcohols with nitrile derivatives catalyzed by Pd/C or Rh/C under hydrogen was reported.6 In addition, 1,3-oxazolidines were synthesized from imines and epoxides catalyzed by samarium compounds.7 All these methods suffered the disadvantages of low efficiency, expensive catalysts, and unsatisfactory yields. Microwave technology played an important role in synthesis with it’s rapidly form of heating. It was one of the most convenient and efficient paths to promote organic reaction. There were many synthetic chemists who utilized microwave to achieve fast, clean and high-yielding transformations,8 which prompted us to investigate the synthesis of 3-dichloroacetyl oxazolidines by microwave irradiation. With the survey of references, there were few reports on the microwave-assisted synthesis of 2,2,4,5-tetrasubstituted 3-dichloroacetyl oxazolidines. Here we extended our earlier work9 and developed a microwave-irradiated method to synthesize novel 2,2,4,5-tetrasubstituted 3-dichloroacetyl-1,3-oxazolidines starting from the amino alcohol, aldehyde or ketone and dichloroacetyl chloride (Scheme 1).
A mixture of amino alcohol 1 and aldehyde or ketone 2 in benzene was stirred for 15 min at 33 °C, then refluxed to remove water irritated by MW (800 W) for 15 min, the corresponding oxazolidine derivatives 3 were obtained. For the condensation was a reversible process, numerous methods for binding of the liberated water or its removal from the reaction mixture were proposed in order to increase the yields of oxazolidines. Usually, azeotropic distillation was used10 or drying agents were added.11 The possible process was depicted in Scheme 2, and there was equilibrium between open-chain and cyclic tautomers.12 Even very small amount of water in the system strongly affected the state of reversible equilibrium. With the rapid heating mode of microwave irradiation, water was produced and removed from the reaction system right away. Microwave-assisted heating shortened the reflux time.
The ratio of the open-chain and cyclic tautomers considerably changed, depending on the solvent polarity: the fraction of the open-chain tautomer increased in more polar solvents. Stabilization of the open-chain form by polar solvents was explained by formation of a strong hydrogen bond involving the hydroxy group. For the polarity of oxazolidine was lower than imine, the nonpolar solvent was benefit to the formation of oxazolidine. Thus benzene was employed as solvent.
The steric hindrance effect on positions 2 and 4 hindered the acylation of dichloroacetyl chloride with 3, which made the yield of 4h lower than 4a and 4e. With the alkaline conditions, oxazolidine easily transfered to the imine especially for the substitute was H at position 2.13 If there were some substitutes on the oxazolidine ring, the ratio of cyclic tautomers was increased.14 Thus, the yields of 4c and 4f were lower than other compounds.
The structures of all the novel compounds 4a-h were elucidated by elemental analysis and spectroscopic technique data. In their IR spectra a characteristic carbonyl band at around 1670 cm-1 proved the presence of p-π conjunction between N atom and O=C. 1H-NMR spectra of compounds 4a−h gave a characteristic of Cl2CH- with single signal in the δ 6.05 ppm range. For the asymmetry of the oxazolidine ring, the one hydrogen of -N-CH-C splited in multiplet at δ 3.9−4.0 ppm. δ 3.6−3.7 ppm range accounted for the one hydrogen of -C-CH-O-. In the 13C-NMR spectra of the synthesized compounds, the signals observed in the region δ160 ppm accounting for the carbon of Cl2C-, δ 95-100 ppm, δ 80−85 ppm, and δ 60−65 ppm accounting for the signals of the three carbons of oxazolidine ring, which also confirm the formation of oxazolidines.
The bioactivity determination of the novel 3-dichloroacetyl oxazolidine derivatives was carried out on corn from the injury of acetochlor.15 The results were listed in Table 1. The recovery rates of the growth index could be attained over 50% with 25 mg/kg of the 4a−h when the concentration of acetochlor in the soil was 20 mg/kg. Among the compounds tested, 4f showed the best activity against the injury of acetochlor.
In conclusion, we have developed an efficient, fast and convenient method for the microwave-assisted preparation of 2,2,4,5-tetrasubstituted 3-dichloroacetyl-1,3-oxazolidines. This method offers short reaction time and good yields. The preliminary bioactivity results showed that compound 4f attained the best herbicide safener activity of these novel compounds.
EXPERIMENTAL
The Infrared (IR) spectra were taken on a KJ-IN-27G infrared spectrophotometer (KBr). The 1H-NMR spectra and 13C-NMR spectra were recorded on a Bruker Avance 300MHz nuclear magnetic resonance spectrometer with CDCl3 as the solvent and TMS as the internal standard. The elemental analysis was performed on FLASH EA1112 elemental analyzer. The automatic microwave synthesizer was XH-100B of Beijing Xianghu Company. The melting points were determined on Beijng Taike melting point apparatus (X-4) and uncorrected. All the reagents were of analytical reagents grade.
Preparation of 2,2,4,5-tetrasubstituted 3-dichloroacetyl-1,3-oxazolidines.
General Procedure: amino alcohol (1, 0.026 mol), aldehyde or ketone (2, 0.026 mol) and benzene (25 mL) were stirred for 15 min under room temperature. Then, the mixture was heated to reflux under microwave irradiation (800 W) for 15 min and water was stripped off, followed by cooling to 0−4 °C and addition of 3 mL of 33% sodium hydroxide solution. Afterwards 3 mL (0.031 mol) of dichloroacetyl chloride was added dropwise with stirring and cooling in an ice bath. Stirring was continued for 1 h. The organic phase was washed until pH = 7. The organic layer was dried over magnesium sulfate anhydrous and vacuum distillation solvent. Compound 4b−d and 4f were separated on silica gel by column chromatography [V (EtOAc): V (light petroleum) = 1:12]. The other crude products were recrystallized with EtOAc and light petroleum until the white crystals were obtained. The physical and spectra data of the compounds 4a−h are as follows:
3-Dichloroacetyl-4,5-dimethyl-2,2-diethyl-1,3-oxazolidine (4a). Yield 80%. White solid, mp 63-64 °C. IR (KBr, cm-1) ν: 3033-2883 (CH), 1674 (C=O), 1415 (Cl2HC-CO-), 1099 (N-C-O). 1H-NMR (CDCl3) δ: 6.17 (s, 1H, Cl2CH-), 4.32-4.38 (m, 1H, N-CH-C), 3.90-3.99 (m, 1H, C-CH-O), 2.16-2.33 (m, 2H, C-CH2-C), 1.88-1.93 (m, 2H, C-CH2-C), 1.23-1.43 (m, 6H, 2×CH3-C), 0.82-0.94 (m, 6H, 2×CH3-C); 13C-NMR (CDCl3) δ: 160.24, 100.39, 72.54, 65.42, 55.55, 27.55, 27.34, 16.42, 14.35, 8.32, 8.13. Anal. Calcd for C11H19Cl2NO2: C 49.42, H 7.17, N 5.24. Found: C 49.45, H 7.14, N 5.29.
3-Dichloroacetyl-2,2,4-trimethyl-5-ethyl-1,3-oxazolidine (4b). Yield 86%. White solid, mp 64-65 °C. IR (KBr, cm-1) ν: 3028-2870 (C-H), 1666 (C=O), 1409 (Cl2HC-CO-), 1134 (N-C-O); 1H-NMR (CDCl3) δ: 6.13 (s, 1H, Cl2CH-), 3.79-4.05 (m, 2H, N-CH-C and C-CH-O), 1.59-1.68 (m, 8H, 2×CH3-C and C-CH2-C), 1.22-1.44 (m, 3H, CH3-C-N), 0.98-1.04 (m, 3H, CH3-C-C); 13C-NMR (CDCl3) δ: 160.57, 95.37, 84.29, 65.47, 55.55, 27.34, 26.67, 21.95, 16.10, 9.99. Anal. Calcd for C10H17Cl2NO2: C 47.42, H 6.77, N 5.53. Found: C 47.36, H 6.74, N 5.60.
3-Dichloroacetyl-4-methyl-5-ethyl-2-n-propyl-1,3-oxazolidine (4c). Yield 61%. Yellow oil. IR (KBr, cm-1) ν: 2964-2870 (C-H), 1666 (C=O), 1417 (Cl2HC-CO-), 1188 (N-C-O); 1H-NMR (CDCl3) δ: 6.07 (s, 1H, Cl2CH-), 5.13-5.34 (m, 1H, N-CH-O), 3.77-4.14 (m, 2H, N-CH-C and C-CH-O), 1.74-2.33 (m, 2H, C-CH2-C), 1.40-1.53 (m, 4H, 2×C-CH2-C), 1.21-1.24 (m, 3H, CH3-C-N), 0.92-1.04 (m, 6H, 2×CH3-C-C); 13C-NMR (CDCl3) δ: 161.15, 90.39, 81.58, 55.37, 54.77, 35.96, 22.01, 17.76, 15.45, 13.93, 10.35. Anal. Calcd for C11H19Cl2NO2: C 49.42, H 7.17, N 5.24. Found: C 49.46, H 7.20, N 5.18.
3-Dichloroacetyl-2,4-dimethyl-5-ethyl-2-n-propyl-1,3-oxazolidine (4d). Yield 71%. White solid, mp 68-69 °C. IR (KBr, cm-1) ν: 3030-2870 (C-H), 1670 (C=O), 1411 (Cl2HC-CO-), 1135 (N-C-O); 1H-NMR (CDCl3) δ: 6.11 (s, 1H, Cl2CH-), 3.75-4.02 (m, 2H, C-CH-N and C-CH-O), 1.60-1.82 (m, 5H, CH3-C-CH2), 1.38-1.52 (m, 5H, CH3-C-N and CH2-C-O), 1.18-1.20 (m, 2H, C-CH2-C), 0.86-1.01 (m, 6H, 2×CH3-C-C); 13C-NMR (CDCl3) δ: 160.64, 99.22, 84.36, 65.54, 57.40, 40.81, 27.54, 24.65, 22.16, 17.54, 14.25, 10.38. Anal. Calcd for C12H21Cl2NO2: C 51.23, H 7.53, N 4.98. Found: C 51.26, H 7.58, N 4.94.
3-Dichloroacetyl-4-methyl-2,2,5-triethyl-1,3-oxazolidine (4e). Yield 76%. White solid, mp 48-49 °C. IR (KBr, cm-1) ν: 3033-2880 (C-H), 1662 (C=O), 1415 (Cl2HC-CO-), 1130 (N-C-O); 1H-NMR (CDCl3) δ: 6.16 (s, 1H, Cl2CH-), 3.96-4.07 (m, 1H, C-CH-N), 3.60-3.75 (m, 1H, C-CH-O), 1.89-2.35 (m, 4H, 2×C-CH2), 1.65-1.70 (m, 2H, C-CH2-C), 1.23-1.44 (m, 3H, CH3-C-N), 0.78-1.06 (m, 9H, 2×C-CH3 and CH3-C-C-O); 13C-NMR (CDCl3) δ: 160.19, 100.23, 83.62, 65.30, 54.97, 27.50, 27.20, 22.11, 16.28, 10.53, 8.39, 8.20. Anal. Calcd for C12H21Cl2NO2: C 51.23, H 7.53, N 4.98. Found: C 51.18, H 7.56, N 4.92.
3-Dichloroacetyl-4-methyl-5-ethyl-2-benzyl-1,3-oxazolidine (4f). Yield 66%. Yellow oil. IR (KBr, cm-1) ν: 2966-2875 (C-H), 1677 (C=O), 1413 (Cl2HC-CO-), 1166 (N-C-O); 1H-NMR (CDCl3) δ: 7.32-7.49 (m, 5H, C6H5-), 6.10 (s, 1H, Cl2CH-), 5.46 (s, 1H, CH-Ar), 4.25-4.50 (m, 1H, C-CH-N), 3.95-4.02 (m, 1H, C-CH-O), 1.59-1.73 (m, 2H, C-CH2-C), 1.35-1.44 (m, 3H, CH3-C-N), 0.97-1.06 (m, 3H, CH3-C-C-O); 13C-NMR (CDCl3) δ: 160.97, 136.36, 130.75, 129.87, 129.87, 127.43, 127.43, 90.17, 82.15, 64.50, 56.16, 21.79, 13.68, 10.43. Anal. Calcd for C14H17Cl2NO2: C 55.80, H 5.69, N 4.65. Found: C 55.72, H 5.75, N 4.60.
3-Dichloroacetyl-2,2-dimethyl-4,5-diethyl-1,3-oxazolidine (4g). Yield 75%. White solid, mp 62-63 °C. IR (KBr, cm-1) ν: 3050-2880 (C-H), 1670 (C=O), 1409 (Cl2HC-CO-), 1142 (N-C-O); 1H-NMR (CDCl3) δ: 6.13 (s, 1H, Cl2CH-), 3.98-4.12 (m, 1H, C-CH-N), 3.70-3.82 (m, 1H, C-CH-O), 1.51-1.83 (m, 10H, 2×C-CH2-C and 2×CH3-C), 0.96-1.06 (m, 6H, 2×CH3-C-C); 13C-NMR (CDCl3) δ: 160.44, 95.20, 79.15, 65.87, 60.97, 27.24, 23.67, 22.47, 21.83, 11.12, 10.98. Anal. Calcd for C11H19Cl2NO2: C 49.42, H 7.17, N 5.24. Found: C 49.36, H 7.21, N 5.22.
3-Dichloroacetyl-2,2,4,5-tetraethyl-1,3-oxazolidine (4h). Yield 70%. White solid, mp 53-54 °C. IR (KBr, cm-1) ν: 2980-2870 (C-H), 1662 (C=O), 1411 (Cl2HC-CO-), 1130 (N-C-O); 1H-NMR (CDCl3) δ: 6.08 (s, 1H, Cl2CH-), 3.96-4.02 (m, 1H, C-CH-N), 3.65-3.72 (m, 1H, C-CH-O), 1.59-1.88 (m, 8H, 4×C-CH2-C), 0.96-1.06 (m, 6H, CH3-C-C-N and CH3-C-C-O), 0.79-0.90 (m, 6H, 2×CH3-C); 13C-NMR (CDCl3) δ: 160.53, 99.93, 79.02, 65.93, 60.96, 27.86, 27.16, 24.78, 21.94, 11.84, 11.03, 8.38, 8.24. Anal. Calcd for C13H23Cl2NO2: C 52.86, H 7.85, N 4.75. Found: C 52.85, H 7.81, N 4.72.
ACKNOWLEDGEMENTS
This work was supported by the National Nature Science Foundation of China (No. 31101473) and Research Science Foundation in Technology Innovation of Harbin (No. 2010RFQYN108).
References
1. (a) W. Adam and S. B. Schambony, Org. Lett., 2001, 3, 79; CrossRef (b) C. Agami, S. Comesse, and C. Kadouri-Puchot, J. Org. Chem., 2002, 67, 1496; CrossRef (c) A. Iwata, H. Tang, A. Kunai, J. Ohshita, Y. Yamamoto, and C. Matui, J. Org. Chem., 2002, 67, 5170. CrossRef
2. (a) C. Agami and F. Couty, Eur. J. Org. Chem., 2004, 677; CrossRef (b) J. Blanchet, B. L. Micouin, and H.-P. Husson, J. Org. Chem., 2000, 65, 6423. CrossRef
3. (a) T. Matola and I. Jablonkai, Crop Protection, 2007, 26, 278; CrossRef (b) J. Davies and J. C. Caseley, Pestic. Sci., 1999, 55, 1043; CrossRef (c) A. W. Abu-Qare and H. J. Duncan, Chemosphere, 2002, 48, 965. CrossRef
4. (a) K. Schwekendiek and F. Glorius, Synthesis, 2006, 18, 2996; CrossRef (b) J. T. Yli-Kauhaluoma, C. W. Harwig, P. Wentworth, and K. D. Janda, Tetrahedron Lett., 1998, 39, 2269; CrossRef (c) F. Polyak, T. Dorofeeva, G. Zelchans, and G. Shustov, Tetrahedron Lett., 1996, 37, 8223. CrossRef
5. (a) J.-G. Shim and Y. Yamamoto, Tetrahedron Lett., 1999, 40, 1053; CrossRef (b) A. R. Katritzky, D. Feng, and M. Qi, Tetrahedron Lett., 1998, 39, 6835. CrossRef
6. (a) S. Létinois, J.-C. Dumur, F. Hénin, and J. Muzart, Tetrahedron Lett., 1998, 39, 2327; CrossRef (b) F. Henin, S. Letinois, and J. Muzart, Tetrahedron Lett., 1997, 38, 7187. CrossRef
7. T. Nishitani, H. Shiraishi, S. Sakaguchi, and Y. Ishii, Tetrahedron Lett., 2000, 41, 3389. CrossRef
8. S. Caddick and R. Fitzmaurice, Tetrahedron, 2009, 65, 3325. CrossRef
9. Y. Fu, H. G. Fu, F. Ye, J. D. Mao, and X. T. Wen, Synth. Commun., 2009, 39, 2454. CrossRef
10. F. Fulop, K. Pihlaja, K. Neuvonen, G. Bernath, G. Argay, and A. Kalman, J. Org. Chem., 1993, 58, 1967. CrossRef
11. (a) C. Agami, S. Comesse, C. Kadouri-Puchot, and M. Lusinchi, Synlett, 1999, 1094; CrossRef (b) E. I. Jwanowicz, P. Blomgren, P. T. W. Cheng, K. Smith, W. F. Lau, Y. Y. Pan, H. H. Gu, M. F. Malley, and J. Z. Gougoutas, Synlett, 1998, 664; CrossRef (c) P. Mangeney, A. Alexakis, and J. E. Normant, Tetrahedron, 1984, 40, 1803. CrossRef
12. Y. Fu and F. Ye, J. Chem. Eng. Chinese Univ., 2006, 20, 852.
13. L. Lázár and F. Fülöp, Eur. J. Org. Chem., 2003, 3025. CrossRef
14. N. A. Keiko, E. A. Funtikova, L. G. Stepanova, Y. A. Chuvashev, and L. I. Larina, Russ. J. Org. Chem., 2003, 39, 1477. CrossRef
15. J. W. Gronwald, E. P. Fuerst, C. V. Eberlein, and M. A. Egli, Pestic. Biochem. Phys., 1987, 29, 66. CrossRef