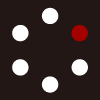
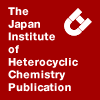
HETEROCYCLES
An International Journal for Reviews and Communications in Heterocyclic ChemistryWeb Edition ISSN: 1881-0942
Published online by The Japan Institute of Heterocyclic Chemistry
e-Journal
Full Text HTML
Received, 18th August, 2011, Accepted, 29th September, 2011, Published online, 6th October, 2011.
DOI: 10.3987/COM-11-12340
■ A New Synthetic Approach to Pentacyclic Ring, 6,7,14,15-Tetrahydro[1,5]diazocino[1,2-a:6,5-a’]-8-dihydrodiindole
Kittisak Sripha,* Darius Paul Zlotos, UIrike Holzgrabe, and Somsak Ruchirawat
Department of Pharmaceutical Chemistry, Faculty of Pharmacy, Mahidol University, 447, Sri-Ayudhya Rd. Rachatevee, Bangkok 10400, Thailand
Abstract
The construction of a new pentacyclic ring system, 6,7,14,15-tetrahydro[1,5]diazocino[1,2-a:6,5-a′]-8-dihydrodiindole (7) from simple precursors through our new synthetic route was presented to be a facile and concise method for an intramolecular N-alkylation of tosylate 12 which was obtained from N-acylation of indoline 4 with indole acid 9, followed by reduction and tosylation. This new synthetic approach can overcome the disadvantage of uncontrollable intermolecular double N-alkylation of the precursor 3 to produce the unexpected ring system 2.The synthesis of the derivatives of 6,7,14,15-tetrahydro[1,5]diazocino[1,2-a:6,5-a′]diindole was reported.1 Because of the potent allosteric modulators on muscarinic M2 receptors of bisquaternary analogs of Strychnos alkaloid caracurine V,2,3 the idea to generate an uncomplicated ring skeleton derived from caracurine V was explored. This ring system was designed by simplify the complex analog of Caracurine V to the essential pharmacophore of two positively charged nitrogens at a distance of about 10 Å surrounded by two aromatic ring systems.4 The accomplish construction of the ring system involved the self-condensation of N,N-disubstituted-ethanamine-2-(2-bromoethyl)-1H-indole. In order to decorate the varieties of the length of side-chains, the synthesis only pentacyclic ring skeleton without the side changes was investigated by the dimerization of the 2-(2-tosylethyl)-1H-indole 3. Interestingly, under strong base condition, the unexpected isomeric hexacylcic ring system, 7,7-a,13,14-tetrahydro-6H-cyclobuta[b]pyrimido[1,2-a:3,4-a′]diindole 2 was found instead of the expected desired ring system 15 (Figure 1).
Due to the highly reactive of indole ring at C-3 position toward both intra- and inter-molecular alkylations, the dimerization of 3 to form 1 could not be controlled. The C-3 indole acted as nucleophile, so, the intramolecular cyclization was constructed the four-membered ring up, followed by intermolecular cyclization from another indolyl anion. In fact, the ring with the most strain would be formed difficultly. Nevertheless, the intramolecular cyclization to form the strained 4-membered ring was favorable because of the less entropy. Furthermore, the proximity effect should be stated in this case owning to the close position between C-3 indole which acted as nucleophile and electrophilic carbon of a flexible ethylene side chain. The formation of 6-membered ring by intermolecular cyclization was favorable more than 8-membered ring because of the less ring strain and less entropy. The mechanism for the formation of 2 was proposed by Attia.5 To overcome this uncontrollable dimerization of 3, an idea of new synthesis pathway involved a connection side by side between indole and indoline derivatives was generated as shown in Scheme 1.
The two precursors of this route, tosylate 3 and indoline ethyl ester 4, could be prepared from known ethyl-indol-2-ylacetate.6 The tosylate 3 was synthesized in two step from ethyl-indol-2-ylacetate using the reaction sequences describe previously by Attia.5 The reduction of ethyl-indol-2-ylacetate to the corresponding 4 was carried out by using borane-trimethylamine complex in trifluoroacetic acid.7 N-substitution of 4 by the tosylate 3 was attempted by using triethylamine in dry tetrahydrofuran (THF) or potassium carbonate in dry acetonitrile (Scheme 2). Unfortunately, no desired product was obtained under the condition using trimethylamine as reagent, and surprisingly, a complex decacyclic heterocycles 8 (13%) was obtained instead of the expected product 5 when the reaction mixture was refluxed in dry acetonitrile in the presence of potassium carbonate. Using the data establishment from the experimental techniques of H,H-COSY, and HMQC with the assistance of NMR analysis data of 2, the structural feature of 8 comprising of tetrahydro-cyclobutyl indole connected with two indoles was illustrated. So, under the desired conditions in Scheme 1, the further steps to reach compound 6 and 7 were not carried out.
The initial formation of the amide 10 was another synthetic strategy to connect the side chain of the indole acid 9 to the N-atom of indoline 4. The acid 9 was coupled with indoline 4 in the presence of N-(3-dimethylaminopropyl)-N′-ethylcarbodiimide hydrochloride (EDCI·HCl) to obtain amide 10 in 65% yield. Simultaneous amide and ester reduction using lithium aluminium hydride (LiAlH4) yielded the unstable alcohol 11, which was used for the tosylation step without purification to give the tosylate 12 in 72%. The final formation of the targeted pentacyclic ring system 7 comprising one indole and one indoline moiety was achieved by means of intramolecular cyclization using potassium hydroxide (KOH) in dry dimethylformamide in 65% yield (Scheme 3).
From this synthetic pathway, the consecutive connection using ethylene side chain of indole and indoline derivatives could overcome the drawback involved the entropy effect. The attachment of N-indoline 4 with the side chain of indole acid 9 was carried out by means of N-acylation. After the reduction and tosylation, in the presence of strong base, the proximity of N-indole and ethylene side chain of indoline comforted the intramolecular N-alkylation to gain the expected pentacyclic ring system. The complete NMR assignment of 7 were carried out using H,H-COSY, HMQC, and HMBC. The connecting pathway in the COSY correlation starting from germinal coupling of H-8b at δ = 2.50 ppm and H-8a at δ = 3.32 ppm to H-7a, and further to H-7b, H-7a and H-6b, H-6a revealed the alicyclic spin system of indoline and one side of ethylene groups within the central diazocine ring. The remaining alicyclic signals correspond to the other side of ethylene protons at C-14 and C-15 revealed as multiplet at δ = 3.14 and 3.87 ppm, respectively. The HMQC data was established a high rigidity of this ring system because of the separately wide germinal hydrogens of diazocine ring. All new synthesized compounds were also characterized by IR, 1H NMR, 13C NMR, and MS.
In summary, a new synthetic route towards the pentacyclic ring system, 6,7,14,15-tetrahydro[1,5]diazocino[1,2-a:6,5-a′]-8-dihydrodiindole was established. Starting from N-acylation of indoline 4 with indole acid 9, the targeted pentacyclic ring could be achievable in four steps in an overall yield of 31%. This synthetic route can suppress the production of unexpected ring system from the uncontrollable intermolecular N-alkylation of its precursor, 2-(2-tosylethyl)-1H-indole 3, due to the consecutive connection of ethylene side chains of indole and indoline derivatives, The concise synthesis of this new pentacyclic ring skeleton will be useful for the synthetic methodology in the medicinal chemistry research of muscarinic receptors. The various side chains of this ring skeleton can be provided as new investigated analogs of allosteric ligands at the muscarinic receptors.
EXPERIMENTAL
Starting compounds and reagents were purchased from commercial suppliers. All melting points were determined using a capillary melting point apparatus (Gallenkamp, Sanyo) and are uncorrected. Column chromatography was carried out on silica gel 60 (0.063-0.200 nm) obtained from Merck. A Bruker AV-400 spectrophotometer was used to obtain 1H NMR (400 MHz) and 13C NMR (100 MHz) spectra, respectively. The chemical shifts (δ) were reported in ppm relative to CDCl3 (7.24 for 1H, 77.0 for 13C). IR spectra were recorded as ATR technique on Bio Rad, PharmalyzIR instrument. Mass spectra using EI technique were determined on a Finnigan MAT 8200 spectrophotometer. High resolution mass spectra were obtained using Bruker Micro time-of-flight mass spectrometry.
Starting Materials. 2-(2-tosylethyl)-1H-indole (3),5,7 ethyl-2-(indolin-2-yl)acetate (4),8 2-(1H-indol-2yl)acetic acid (9)9,10 were prepared followed the report procedures. All other chemicals used in this study were commercially available.
Ethyl 2-(1-(2-(1H-indol-2-yl)acetyl)indolin-2-yl)acetate (10). To the mixture of 1H-indole-2-acetic acid (0.8 g, 4.57 mmol) and ethyl-2,3-dihydro-1H-indole-2-acetate (1.03 g, 5.02 mmol) in dry CH2Cl2 (80 mL), under argon, EDCI·HCl (1.31 g, 6.85 mmol) was added. The reaction mixture was allowed to stir at room temperature for 2 h. The solvent was removed in vacuum to yield an brown oil which was subjected to chromatograph using EtOAc:Hex/1:2 as an eluent to obtain 1.24 g (74%) of 10 as a brown solid: mp 108.7-109.6 oC; TLC Rf = 0.4 (SiO2, EtOAc:Hex/1:2); FT-IR (ATR) ν (cm-1) 3350, 2928, 1713, 1630, 1595, 1402, 1192, 1152, 746, 687, 666; 1HNMR (400 MHz, CDCl3) δ 9.13 (brs, 1H, NH), 8.15 (d, 1H, J = 6.4 Hz, H-4), 7.55 (d, 1H, J = 7.7 Hz, H-4′), 7.24 (m, 2H, H-5 and H-6′), 7.15 (td, 1H, J = 7.6, 1.1 Hz, H-6), 7.10-7.07 (m, 2H, H-5′ and H-7′), 6.41 (s, 1H, H-3), 5.21-4.99 (m, 1H, H-2′), 4.20-4.06 (m, 4H, CH2-CO-N and CH3-CH2-O), 3.42 (dd, 1H, J = 8.7, 8.6 Hz H-3′a), 2.84 (d, 1H, J = 16.1 Hz, H-3′’b), 2.71-2.58 (m, 2H, CH2-CO-O), 1.27 (t, 3H, J = 7.1 Hz, O-CH2-CH3); 13C NMR (100 MHz, CDCl3) δ 170.3 (CO-O), 167.7 (CO-N), 136.4 (C-7′a), 131.1 (C-3′a), 130.0 (C-7a), 128.1 (C-3a), 127.6 (C-5), 125.1 (C-5′), 124.7 (C-6′), 121.6 (C-6), 120 (C-4′), 119.7 (C-7′), 118.1 (C-4), 110.9 (C-7), 101.4 (C-3), 60.4 (CH3-CH2-O), 57.2 (C-2′), 40.2 (CH2-CO-O), 34.8 (CH2-CO-N), 14.1 (O-CH2-CH3); MS (EI, 70 eV) m/z (rel. int) 362 [M+] (36), 205 (58), 190 (37), 130 (100), 118 (39). HRMS calcd. for C22H22N2O3, m/z: 362.1630 (M+); Found, m/z: 362.1631.
1-(2-(2-Hydroxyethyl)indolin-1-yl)-2-(1H-indol-2-yl)ethanone (11). To a suspension of LiAlH4 (0.2 g, 5.0 mmol) in dry THF (20 mL) under argon at 0 oC, the solution of 10 (0.53 g, 1.46 mmol) was added dropwise. The reaction mixture was stirred at room temperature for 3 h and then terminated by adding carefully water (0.4 mL) after cooling down at 0 oC. The suspension was stirred vigorously at room temperature for 1 h and precipitate was filtered out. The filtrate was dried over anhydrous MgSO4 and evaporated in vacuo. The crude product 11 was obtained as a neat pale yellowish liquid (0.34 g, 76%). It was subsequently used for the further synthesis without purification; TLC Rf = 0.52 (Silica gel, CHCl3:MeOH/10:0.5); 1HNMR (400 MHz, CDCl3) δ 8.30 (s, 1H, NH), 7.55 (d, 1H, J = 7.59 Hz, H-4), 7.28 (d, 1H, J = 8.0 Hz, H-4′), 7.15-7.06 (m, 4H, H-5, H-5′, H-6′, H-7), 6.74 (t, 1H, J = 7.31, H-6), 3.86-3.68 (m, 3H, H-2′, H-9′), 3.51 (td, 2H, J = 7.36, 3.37 Hz, H-9), 3.15 (dd, 1H, J = 15.60, 8.77 Hz, H-3′a), 3.06-2.98 (m, 2H, H-8), 2.79 (dd, 1H, J = 15.57, 9.55 Hz, H3′b), 2.03-1.79 (m, 2H, H-8′); 13C NMR (100 MHz, CDCl3) δ 137.3 (C-7′a), 136.0 (C-7a), 128.6 (C-3a), 127.6 (C-3′a), 124.5 (C-6′), 121.2 (C-4′), 119.8 (C-6), 119.7 (C-5′), 118.7 (C-4), 110.5 (C-5 and C-7), 107.9 (C-7′), 62.7 (C-9′), 59.7 (C-2′), 35.5 (C-8′), 34.6 (C-3′), 25.9 (C-8).
2-(1-(2-(1H-Indol-2-yl)ethyl)indolin-2-yl)ethyl 4-methylbenzenesulfonate (12). The solution of p-toluenesulfonyl chloride (0.7 g, 3.5 mmol) in dry CH2Cl2 (30 mL) was added dropwise into a solution of crude product 11 and triethylamine (1.0 mL) in dry CH2Cl2 (30 mL) under argon at 0 oC. The reaction mixture was stirred at room temperature overnight. After removing the organic solvent, the residue was subjected to chromatograph by using CHCl3:Hex/3:1 as eluting solvent. The pure compound of 12 was obtained as brown solid (0.54 g, 80%); mp 110-113 oC; TLC Rf = 0.51 (SiO2, CHCl3); FT-IR (ATR) ν (cm-1) 3393, 3250, 3049, 2956, 2924, 1604, 1484, 1457, 1353, 1173, 1120, 1009, 746, 681, 664; 1HNMR (400 MHz, CDCl3) δ 8.37 (brs, 1H, NH), 7.75 (d, 1H, J = 8.3 Hz, H-4), 7.69 (d, 1H, J = 8.3 Hz, H-4′), 7.54 (d, 1H, J = 7.47 Hz, H-7), 7.31-7.04 (m, 8H, H-5, H-5′, H-6, H-6′, H-a, H-b), 6.28 (d, J = 1.0 Hz, H-3), 4.18-4.06 (m, 2H, H-9′), 3.72-3.64 (m, 2H, H-2′ and H-7′), 3.48 (m, 1H, H-9), 3.07-2.94 (m, 2H, H-8 and H-3′a), 2.61 (dd, 1H, J = 15.6, 9.2 Hz, H3′b), 2.41 (s, 1H, -CH3), 2.03-1.79 (m, 2H, H-8′); 13C NMR (100 MHz, CDCl3) δ 136.4 (C-7′a), 131.1 (C-3′a), 130.0 (C-7a), 128.1 (C-3a), 127.6 (C-5), 125.1 (C-5′), 124.7 (C-6′), 121.6 (C-6), 120 (C-4), 119.7 (C-7′), 118.1 (C-4′), 110.9 (C-7), 101.4 (C-3), 67.6 (C-9′), 61.5 (C-2′), 48.1 (C-9), 34.9 (C-3′), 33.2 (C-8′), 30.1 (C-2), 25.9 (C-8), 21.6 (-CH3); MS (EI, 70 eV) m/z (rel. int) 289 (100), 145 (58), 131 (35). HRMS calcd. for C20H20N2, m/z: 460.1821 (M+); Found, m/z: 460.1815.
6,7,14,15-Tetrahydro[1,5]diazocino[1,2-a:6,5-a′]-8-dihydrodiindole (7). At 0 oC, a powder of potassium hydroxide (0.07 g, 1.3 mmol) was added to a solution of 12 (0.14 g, 0.3 mmol) in dry DMF (6 mL). The reaction mixture was stirred overnight at room temperature. After the reaction was complete, water (30 ml) was added and the dark suspension was extracted with Et2O (4 x 20 ml). The combined organic phases were washed with water (3 x 20 mL) and brine (20 ml) and dried over anhydrous MgSO4. The solvent was removed in vacuo to obtain an brown neat residue, which was chromatographed eluting with CHCl3:Hex/1:1 to give the 7 (0.06 g, 68%) as a white solid; mp 144 – 145.8 oC (crystallization from CHCl3 and Hex); TLC Rf = 0.42 (SiO2, CHCl3:Hex/1:1); FT-IR (ATR) ν (cm-1) 3019, 2951, 2927, 1603, 1542, 1482, 1455, 1415, 1354, 1213, 1132, 1018, 897, 784, 734, 724, 676; 1HNMR (400 MHz, CDCl3) δ 7.58 (d, 1H, J = 7.8 Hz, H-4), 7.33 (d, 1H, J = 8.1 Hz, H-1), 7.21-7.08 (m, 3H, H-2, H-3 and H-12), 7.04 (d, 1H, J = 7.1 Hz, H-9), 6.75 (m, 2H, H-10 and H-11), 6.31 (s, 1H, H-16), 4.62-4.55 (m, 1H, H-6a), 4.29 (dd, 1H, J = 14.7, 4.0 Hz H-6b), 3.88-3.85 (m, 1H, H-14a), 3.32 (dd, 1H, J = 16.2, 10.3 Hz, H-8a), 3.17-3.10 (m, 3H, H-14b, H-15a and H-15b), 2.85-2.79 (m, 1H, H-7a), 2.50 (d, J = 16.0 Hz, H-8b), 2.17-2.08 (m, 1H, H-7a), 1.84-1.79 (m, 1H, H-7b); 13C NMR (100 MHz, CDCl3) δ 153.3 (C-12a), 138.9 (C-15a), 136.1 (C-4a), 135.0 (C-16a), 129.5 (C-8a), 128.2 (C-3), 127.6 (C-9), 124.9 (C-2), 120.8 (C-10), 120.1 (C-12), 119.4 (C-4), 113.1 (C-1), 109.1 (C-1), 99.3 (C-16), 61.2 (C-7a), 58.1 (C-14), 39.1 (C-6), 37.7 (C-7), 36.3 (C-8), 29.7 (C-15); MS (EI, 70 eV) m/z (rel. int) 288 [M+] (100), 172 (45), 158 (16), 144 (83), 130 (68), 115 (13). HRMS calcd. for C20H20N2, m/z: 288.1626 (M+); Found, m/z: 288.1625.
ACKNOWLEDGEMENT
Financial support from the TRF-CHE Research Grant for New Scholar (MRG4880025) and the DAAD is gratefully acknowledged.
References
1. K. Sripha and D. P. Zlotos, Tetrahedron, 2003, 59, 391. CrossRef
2. U. Holzgrabe and K. Mohr, Drug Discov. Today, 1998, 3, 214. CrossRef
3. D. P. Zlotos, S. Buller, C. Traenkle, and K. Mohr, Bioorg. Med. Chem. Lett., 2000, 10, 2529. CrossRef
4. U. Holzgrabe and A. J. Hopfinger, J. Chem. Inf. Comput. Sci., 1996, 36, 1018. CrossRef
5. M. I. Attia, D. Gueclue, B. Hertlein, J. Julius, P. A. Witt-Enderby, and D. P. Zlotos, Org. Biomol. Chem., 2007, 5, 2129. CrossRef
6. C. J. Moody and K. F. Rahimtoola, J. Chem. Soc., Perkin Trans. 1, 1990, 673. CrossRef
7. J. Bergman and B. Pelcman, Tetrahedron, 1988, 44, 5215. CrossRef
8. S. Coulton, T. L. Gilchrist, and K. J. Graham, J. Chem. Soc., Perkin Trans. 1, 1998, 1993.
9. M. I. Attia, J. Julius, P. A. Witt-Enderby, and D. P. Zlotos, Tetrahedron, 2007, 63, 754. CrossRef
10. W. Schindler, Helv. Chim. Acta, 1958, 41, 1441. CrossRef