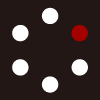
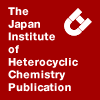
HETEROCYCLES
An International Journal for Reviews and Communications in Heterocyclic ChemistryWeb Edition ISSN: 1881-0942
Published online by The Japan Institute of Heterocyclic Chemistry
e-Journal
Full Text HTML
Received, 26th August, 2011, Accepted, 10th November, 2011, Published online, 8th December, 2011.
DOI: 10.3987/COM-11-12347
■ 1,3-Dipolar Cycloaddition Reaction in Porphyrin Systems with Functionalized Alkyl Nitrile Oxides — Synthesis of Isoxazoline-Fused Chlorins
Przemysław Wyrębek, Agnieszka Mikus, and Stanisław Ostrowski*
Institute of Chemistry, Uniwersytet Przyrodniczo-Humanistyczny w Siedlcach, ul. 3 Maja 54, 08-110 Siedlce, Poland
Abstract
meso-Tetraphenylporphyrin reacts at higher temperature with unstable alkyl nitrile oxides (R–C≡N→O) affording isoxazoline-fused chlorins according to dipolar [3+2]-cycloaddition pathway. The respective nitrile oxides were in situ generated from the corresponding functionalized nitroalkanes in the presence of base (NEt3, DABCO) and dehydrating agent (PhNCO, (Boc)2O). Substituent R bearing diverse of functionality allows synthesis of very attractive moieties which may be of potential use as sensitizers in photodynamic therapy. The products obtained are also suitable intermediates for further derivatization of porphyrins.In the past decade numerous investigations oriented towards the synthesis and utilization of chlorins and bacteriochlorins have been undertaken. These compounds may be considered as second generation photosensitizers in antitumor photodynamic therapy (PDT)1 due to their characteristic strong absorption bands shifted to the red region of visible spectrum (630-780 nm).
The attractive chlorin systems can be synthesized by various methods.2 One of the approaches involves 1,3-dipolar cycloaddition reaction of peripheral β,β-double bonds of porphyrin moiety with some 1,3- dipoles. Among others, nitrile oxides could be used for this purpose. In the recent past we reported our preliminary results concerning this type of cycloaddition with the use of alkyl nitrile oxides,3 generation of which is relatively difficult, and which are generally less stable4 as compared to the aryl ones. It was one of the first three published works in which synthesis of fused porphyrin-isoxazoline derivatives was described.3,5a,b
Herein we report our further investigations oriented towards the synthesis of diversely functionalized chlorins. Studies on the scope and limitations of this derivatization method and exploring other possibilities of generation this type of alkyl nitrile oxides (and their applications in the above cycloaddition) were attempted. The previous reactions of meso-tetraphenylporphyrin (1) with an excess of alkyl nitrocompounds 2a-c, carried out in the presence of NEt3 and PhNCO in refluxing benzene (Mukaiyama’s method4a), gave the expected isoxazoline-fused chlorins (3a-c)3 (Scheme 1).
The reactions were carried out in benzene due to a good solubility of the reagents in this solvent. The generated in situ nitrile oxides readily enter into the cycloaddition to β,β-double bond, which exhibits considerable olefinic character. Every 3 hours new portions of the substrates were supplied and the reaction mixtures were heated up to ca 50 hours. Due to the various difficulties, e.g. addition of new portions of R-NO2, NEt3, and PhNCO (to supplement the loss of the formed in situ nitrile oxide), long reaction time, and fast degradation of nitrile oxides, the yields of the chlorins were rather low or moderate (10-30%), and we recovered considerable amounts of the starting porphyrin. The prolonged reaction time did not give higher yields, because in these conditions the progressive degradation of the products was observed. Thus, some attempts concerning optimization of the reaction conditions were undertaken by changing the temperature, solvent, base, and dehydrating agent. These results are listed in Table 1.
It is known that most of the cycloaddition reactions in porphyrin systems usually takes place at higher temperature.2d,7 In our case, the raising of the temperature did not give better yields; however, it allows shortening the reaction time (from 50 h to 2 h, see entries 2 and 8 in Table 1). Probably the most important limitation herein is the moderate reactivity of the porphyrin as dipolarophile. Nevertheless, this synthesis is a challenging task because the chlorins prepared by the above method, bearing lipophilic alkyl chains, can be transformed into hydrophilic ketones, amines, alcohols, etc.,8 and therefore may lead to very attractive amphiphilic systems,9 which are sought in many fields of this chemistry.
In the next step, some attempts concerning generation of the nitrile N-oxides were undertaken. Historically, the first method involves elimination of hydrogen halides from the aliphatic aldoxime derivatives. The starting halogeno-aldoximes are rather unstable, thus they are usually used in the reactions as solutions, directly after their preparation. However, in our case, we could not improve the yields by using these precursors (Scheme 2).
Also the literature method10 involving chloramine-T (with the use of oxime 4) completely failed. The same result was observed for application of nitrolic acids11 (for example PhCH2C(NO2)=N-OH; toluene/reflux or DMSO/120 ºC; 10-20 h). None of the product was formed in these cases, even with activated porphyrins: meso-tetrakis(2,6-dichlorophenyl)porphyrin and meso-tetrakis(pentafluorophenyl)porphyrin.12
Finally, we applied the modification described by Basel and Hassner (DMAP, (Boc)2O).13 From the several solvents tested (e.g. toluene, cyclooctane, CHCl3) we choose cyclooctane, and the base DMAP was exchanged for DABCO. Under these conditions we carried out the remaining reactions with nitroalkanes bearing highly functionalized groups R (see Scheme 3 and Table 2).
The chlorins 3a-h were identified by 1H NMR, MS, and UV-Vis methods. The 1H NMR spectra reveal characteristic broad singlets at ca -1.75 ÷ -2.00 ppm originating from NH protons (deshielded as compared to porphyrins). The more diagnostic signals of Hβ-protons in reduced pyrrole ring (Hβ-chlorin) were always found as two doublets (J = 9.2 ÷ 10.0 Hz) in the region 6.50–7.80 ppm. The remaining signals of Hβ-protons usually appear as several doublets and one singlet (2H; opposite site to the ’chlorin junction’) in the region 7.92–8.68 ppm. The COSY spectrum measured for compound 3a also confirmed the proposed structure.
The yields of the products were moderate or low but the chlorins obtained are very attractive moieties. They are esters (3d), ethers (3e), acetals (3f-h), etc.; thus may be further transformed to novel diversely decorated chlorins of increased polarity. Additionally, the fused isoxazoline ring conceivably may be also easily cleaved8 to polar functionalities. Such chlorins are sought in antitumor PDT, because in their structures all the desired properties were deposited.
CONCLUSIONS
We presented herein an approach to fused isoxazoline-type chlorins by dipolar [3+2]-cycloaddition of unstable alkyl nitrile oxides to meso-tetraphenylporphyrin. This reaction may well receive future attention in the area of porphyrin skeleton modifications. The products obtained are potentially attractive and versatile intermediates for the further derivatization of meso-arylchlorins designed as second generation photosensitizers1a in PDT. They could be practically applied in this therapy because they absorb low-energy UV-Vis light, and subsequently may be easily converted to soluble in physiological milieu moieties.
EXPERIMENTAL
General. 1H NMR spectra were recorded with a Varian MR-400 spectrometer operating at 400 MHz. Coupling constants J are expressed in hertz [Hz]. Mass spectra were measured with a MARINER PerSeptive Biosystems (ESI-TOF) spectrometer (ESI method), 4000 Q-TRAP Applied Biosystems spectrometer (APPI method), and GCT Premier Waters (FD-TOF) spectrometer (FD method); m/z intensity values for peaks are given as % of relative intensity. UV-Vis spectra were measured with a Beckman DU-68 spectrometer. TLC analysis was performed on aluminium foil plates pre-coated with silica gel (60F 254, Merck); the products have lower Rf values as compared to the starting porphyrin 1 (TLC: CHCl3/n-hexane). Silica gel, 230-400 mesh (Merck AG), was used for column chromatography. Molecular formulas of the compounds synthesised were confirmed by HR-MS (ESI and FD) and by comparing the molecular isotope patterns (theoretical and experimental).
All the nitroalkanes used were commercial products.
Procedure A. To a stirred solution of meso-tetraphenylporphyin (1; 100 mg, 0.163 mmol) and nitrocompound (2a-c; 4.2 mmol) in anhydrous benzene (4 mL), triethylamine (320 μL, 2.30 mmol) was added, and the mixture was stirred for 15 min at room temperature. Then, the reaction mixture was heated to reflux, and a solution of phenyl isocyanate (395 mg, 360 μL, 3.31 mmol) in benzene (1 mL) was added dropwise via syringe (septum) over a period of ca 15 min. The new portions of nitroalkane, NEt3, and PhNCO were added every 3 h. The reaction was carried out under argon in a light-shielded flask equipped with a reflux condenser protected at the top with CaCl2 tube. After 50 h the reaction mixture was cooled to room temperature, the precipitate was filtered off, and the residue was chromatographed (eluent: CHCl3/n-hexane (2:1), then CHCl3) to give the desired product: 3a – 17.7 mg, 16% (66% for recovered 1); 3b – 11.8 mg, 10% (44%); 3c – 35.4 mg, 30% (55%).
Procedure B. To a boiling solution of meso-tetraphenylporphyrin (1; 390 mg, 0.634 mmol) and PhNCO (219 mg, 200 μL, 1.84 mmol) in anhydrous toluene (10 mL), a mixture of nitrohexane (84 mg, 0.64 mmol) and NEt3 (130 μL, 0.93 mmol) in toluene (0.5 mL) was added dropwise via syringe (septum) over a period of ca 15 min. The reaction was carried out under argon in a light-shielded flask equipped with a reflux condenser protected at the top with CaCl2 tube. The new portions of nitrohexane (84 mg, 0.64 mmol) and NEt3 (130 μL, 0.93 mmol) were added every 1 h, and PhNCO (219 mg, 200 μL, 1.84 mmol) – every 6 h. After 30 h the reaction mixture was cooled to room temperature and transferred quantitatively to separatory funnel filled with 100 mL of water. The product was extracted with chloroform (3×25 mL). The combined organic layers were washed with water (100 mL) and dried over anhydrous MgSO4. After evaporating the solvent, chlorin 3c was isolated by column chromatography (eluent: CHCl3/n-hexane, from 2:1 to 4:1). Yield: 37 mg (8%).
Procedure C. To a boiling solution of meso-tetraphenylporphyrin (1; 123 mg, 0.200 mmol), DABCO (90 mg, 0.80 mmol), and (Boc)2O (660 mg, 3.02 mmol) in anhydrous cyclooctane (8 mL), a solution of nitroalkane 2b,d-h (2.0 mmol) in cyclooctane (2 mL) was added dropwise via syringe (septum) over a period of ca 30 min. The reaction was carried out under argon in a light-shielded flask equipped with a reflux condenser protected at the top with CaCl2 tube. The new portions of nitroalkane (2.0 mmol), DABCO (90 mg, 0.80 mmol), and (Boc)2O (660 mg, 3.02 mmol) were added every 5 h. After 20 h the reaction mixture was cooled to room temperature, poured onto 100 mL of water, and extracted with chloroform (5×20 mL). The combined organic layers were dried over anhydrous MgSO4. After evaporating the solvent, the products were isolated by column chromatography (eluent: CHCl3/n-hexane, from 1:1 to 4:1). The desired chlorins (3b,d-h) were found in the second more polar fraction, eluted after the first fraction containing the recovered porphyrin 1. These chlorins were rechromatographed (eluent: CHCl3/n-hexane, 3:1) to give analytically pure products: 3b – 18.6 mg, 13% (35% for recovered 1); 3d – 13.2 mg, 9% (31%); 3e – 12.0 mg, 8% (24%); 3f – 15.6 mg, 10% (40%); 3g – 16.4 mg, 10% (63%); 3h – 0.6 mg, < 1%.
Chlorin 3a: mp > 300 °C. 1H NMR (CDCl3, 400 MHz), δ [ppm]: 8.65 (apparent d, J ∼ 4.9 Hz, 2H, 2×Hβ), 8.56 (d, J = 7.7 Hz, 1H, H-C6H5), 8.49 (s, 2H, 2×Hβ), 8.39-8.15 (m, 6H, 2×Hβ and 4H of H-C6H5), 8.09-7.97 (m, 2H, H-C6H5), 7.87-7.63 (m, 12H, 11H of H-C6H5 and CH [7.67 (d, J = 9.6 Hz)]), 7.62-7.55 (m, 2H, H-C6H5), 6.50 (d, J = 9.6 Hz, 1H, CH), 1.86-1.69 (m, 2H, CH2), 0.58 (t, J = 7.4 Hz, 3H, CH3), -1.75 (s, 1H, NH), -1.79 (s, 1H, NH). UV-Vis (CHCl3), λmax [nm] (lg ε): 645.5 (4.17), 594 (3.69), 548 (3.99), 519.5 (3.98), 414 (4.84, Soret). MS (ESI), m/z (% rel. int.): 688 (5), 687 (38), 686 (100) [isotope (M+H)+]. HR-MS (ESI) calcd for C47H36N5O [(M+H)+]: 686.2920, found: 686.2925.
Chlorin 3b: This compound has been already described in the earlier literature3; the respective 1H NMR spectrum is given here for more detailed and accurate characterization of the product. 1H NMR (CDCl3, 400 MHz), δ [ppm]: 8.63 (apparent d, J ∼ 4.8 Hz, 2H, 2×Hβ), 8.54 (d, J = 7.4 Hz, 1H, H-C6H5), 8.47 (s, 2H, 2×Hβ), 8.39-7.92 (m, 8H, 2×Hβ and 6H of H-C6H5), 7.89-7.12 (m, 14H, 13H of H-C6H5 and CH [7.65 (d, J = 10.0 Hz)]), 6.51 (d, J = 10.0 Hz, 1H, CH), 1.80-1.17 (m, 6H, 3×CH2), 0.64 (t, J = 7.0 Hz, 3H, CH3), -1.79 (broad s, 2H, 2×NH).
Chlorin 3c: mp > 300 °C. 1H NMR (CDCl3, 400 MHz), δ [ppm]: 8.64 (apparent d, J ∼ 4.8 Hz, 2H, 2×Hβ), 8.55 (d, J = 7.6 Hz, 1H, H-C6H5), 8.48 (s, 2H, 2×Hβ), 8.38-7.95 (m, 8H, 2×Hβ and 6H of H-C6H5), 7.85-7.53 (m, 14H, 13H of H-C6H5 and CH [7.65 (d, J = 9.6 Hz)]), 6.50 (d, J = 9.6 Hz, 1H, CH), 1.82-1.63 (m, 2H, CH2), 1.10-0.78 (m, 6H, 3×CH2), 0.70 (t, J = 7.0 Hz, 3H, CH3), -1.75 (s, 1H, NH), -1.79 (s, 1H, NH). UV-Vis (CHCl3), λmax [nm] (lg ε): 645 (3.68), 593 (3.22), 548 (3.52), 519 (3.51), 416 (4.59, Soret). MS (ESI), m/z (% rel. int.): 730 (9), 729 (36), 728 (100) [isotope (M+H)+]. HR-MS (ESI) calcd for C50H42N5O [(M+H)+]: 728.3389, found: 728.3397.
Chlorin 3d: mp > 300 °C. 1H NMR (CDCl3, 400 MHz), δ [ppm]: 8.68 (d, J = 4.8 Hz, 1H, Hβ), 8.63-8.52 (m, 2H, 1Hβ and 1H of H-C6H5), 8.50 and 8.49 (AB, J = 4.5 Hz, 2H, 2×Hβ), 8.40-8.31 (m, 2H, 1Hβ and 1H of H-C6H5), 8.23-7.94 (m, 6H, 1Hβ and 5H of H-C6H5), 7.84 (d, 1H, J = 7.3 Hz, H-C6H5), 7.79-7.60 (m, 10H, CH and 9H of H-C6H5), 7.56-7.47 (m, 3H, H-C6H5), 6.77 (d, J = 9.2 Hz, 1H, CH), 4.02 (q, J = 7.2 Hz, 2H, CH2), 1.16 (t, J = 7.2 Hz, 3H, CH3), -1.93 (s, 1H, NH), -1.99 (s, 1H, NH). UV-Vis (CHCl3), λmax [nm] (lg ε): 643 (4.09), 590.5 (3.57), 545.5 (3.84), 517 (3.87), 417 (4.99, Soret). MS (APPI), m/z (% rel. int.): 732 (19), 731 (61), 730 (100) [isotope (M+H)+]. HR-MS (ESI) calcd for C48H36N5O3 [(M+H)+]: 730.2818, found: 730.2787.
Chlorin 3e: mp > 300 °C. 1H NMR (CDCl3, 400 MHz), δ [ppm]: 8.63 (apparent d, J ∼ 4.8 Hz, 2H, 2×Hβ), 8.52 (d, J = 7.6 Hz, 1H, H-C6H5), 8.48 (s, 2H, 2×Hβ), 8.35-8.15 (m, 6H, 2×Hβ [8.34 (apparent d, J = 4.8 Hz)] and 4H of H-C6H5), 8.10-7.95 (m, 2H, H-C6H5), 7.83-7.62 (m, 12H, 11H of H-C6H5 and CH [7.65 (d, J = 9.7 Hz)]), 7.60-7.51 (m, 2H, H-C6H5), 6.57 (d, J = 9.7 Hz, 1H, CH), 3.64 and 3.48 (AB, J = 14.5 Hz, 2H, CH2), 0.83 (s, 9H, C(CH3)3), -1.81 (s, 1H, NH), -1.83 (s, 1H, NH). UV-Vis (CHCl3), λmax [nm] (lg ε): 647 (3.85), 594 (3.37), 548 (3.68), 519 (3.66), 418 (4.77, Soret). MS (ESI), m/z (% rel. int.): 746 (13), 745 (55), 744 (100) [isotope (M+H)+]. HR-MS (ESI) calcd for C50H42N5O2 [(M+H)+]: 744.3339, found: 744.3300.
Chlorin 3f: mp > 300 °C. 1H NMR (CDCl3, 400 MHz), δ [ppm]: 8.64-8.59 (m, 2H, 2×Hβ), 8.51 (d, J = 7.4 Hz, 1H, H-C6H5), 8.47 (s, 2H, 2×Hβ), 8.33 (d, J = 5.0 Hz, 1H, Hβ), 8.29 (d, J = 4.7 Hz, 1H, Hβ), 8.25-7.92 (m, 6H, H-C6H5), 7.84-7.63 (m, 12H, 11H of H-C6H5 and CH [7.68 (d, J = 9.5 Hz)]), 7.60-7.52 (m, 2H, H-C6H5), 6.65 (d, J = 9.5 Hz, 1H, CH), 4.45 (s, 1H, CH(OCH2-)2), 3.34 (dd, J = 11.3, 2.7 Hz, 1H, Hax of CH2), 3.22 (dd, J = 11.1, 2.7 Hz, 1H, Hax of CH2), 3.14 (d, J = 11.3 Hz, 1H, Heq of CH2), 3.00 (d, J = 11.1 Hz, 1H, Heq of CH2), 0.94 (s, 3H, CH3), 0.59 (s, 3H, CH3), -1.77 (s, 1H, NH), -1.80 (s, 1H, NH). UV-Vis (CHCl3), λmax [nm] (lg ε): 647 (3.91), 594.5 (3.41), 549 (3.75), 520 (3.71), 417 (4.85, Soret). MS (FD), m/z (% rel. int.): 774 (3), 773 (15), 772 (58), 771 (100) [isotope M+]. HR-MS (FD) calcd for C51H41N5O3 [M+]: 771.3209, found: 771.3203.
Chlorin 3g: mp > 300 °C. 1H NMR (CDCl3, 400 MHz), δ [ppm]: 8.63 (apparent d, J ∼ 4.8 Hz, 2H, 2×Hβ), 8.52 (d, J = 7.7 Hz, 1H, H-C6H5), 8.47 (s, 2H, 2×Hβ), 8.36-8.15 (m, 6H, 2×Hβ and 4H of H-C6H5), 8.08-7.93 (m, 2H, H-C6H5), 7.84-7.63 (m, 12H, 11H of H-C6H5 and CH [7.65 (d, J = 9.6 Hz)]), 7.62-7.55 (m, 2H, H-C6H5), 6.50 (apparent t, ’J’ ~ 10.3 Hz, 1H, CH), 4.18-4.14 (m, 1H, CH(OCH2-)2), 3.50-3.34 (m, 2H, OCH2), 3.15-3.00 (m, 2H, OCH2), 2.16-1.96 (m, 2H, CH2), 1.76-1.43 and 1.39-1.15 (2×m, 6H, 3×CH2), -1.78 (s, 1H, NH), -1.82 (s, 1H, NH). UV-Vis (CHCl3), λmax [nm] (lg ε): 647 (4.15), 594.5 (3.64), 548.5 (3.97), 519.5 (3.94), 416.5 (5.06, Soret). MS (FD), m/z (% rel. int.): 788 (7), 787 (26), 786 (70), 785 (100) [isotope M+]. HR-MS (FD) calcd for C52H43N5O3 [M+]: 785.3366, found: 785.3334.
Chlorin 3h: This compound was obtained in small amounts (below 1 mg); its structure was proposed on the basis of MS and UV-Vis spectra. UV-Vis (CHCl3), λmax [nm]: 647, 594.5, 548.5, 521, 419 (Soret). MS (FD), m/z (% rel. int.): 762 (4), 761 (18), 760 (59), 759 (100) [isotope M+]. HR-MS (FD) calcd for C50H41N5O3 [M+]: 759.3209, found: 759.3179. The molecular formula was also confirmed by comparing the theoretical and experimental isotope patterns for the M+ ion (C50H41N5O3); it was found to be identical within the experimental error limits.
ACKNOWLEDGEMENTS
This work was supported by the Ministry of Science and Higher Education, Grants N N204 218535 (2008-2012) and N N204 135438 (2010-2011).
References
1. (a) J. G. Moser, Ed., Photodynamic Tumor Therapy; Harwood Academic Publishers: Amsterdam, 1998; (b) E. D. Sternberg, D. Dolphin, and Ch. Brückner, Tetrahedron, 1998, 54, 4151; CrossRef (c) L. Bourre, G. Simonneaux, Y. Ferrand, S. Thibaut, Y. Lajat, and T. Patrice, J. Photochem. Photobiol. B, 2003, 69, 179; CrossRef (d) E. S. Nyman and P. H. Hynninen, J. Photochem. Photobiol. B, 2004, 73, 1. CrossRef
2. For example: (a) reduction: H. W. Whitlock Jr., R. Hanauer, M. Y. Oester, and B. K. Bower, J. Am. Chem. Soc., 1969, 91, 7485; CrossRef (b) oxidation by OsO4: A. N. Kozyrev, T. J. Dougherty, and R. K. Pandey, Tetrahedron Lett., 1996, 37, 3781; CrossRef Ch. Brückner, S. J. Rettig, and D. Dolphin, J. Org. Chem., 1998, 63, 2094; CrossRef and refs. cited therein: (c) addition of Br2: M. K. Tse, Z.-Y. Zhou, T. C. W. Mak, and K. S. Chan, Tetrahedron, 2000, 56, 7779; CrossRef (d) Diels-Alder reaction: A. C. Tomé, P. S. S. Lacer¬da, M. G. P. M. S. Neves, and J. A. S. Cavaleiro, Chem. Commun., 1997, 1199; CrossRef M. G. H. Vicente, M. T. Cancilla, C. B. Lebrilla, and K. M. Smith, Chem. Commun., 1998, 2355; CrossRef A. M. G. Silva, A. C. Tomé, M. G. P. M. S. Neves, and J. A. S. Cavaleiro, Tetrahedron Lett., 2000, 41, 3065; CrossRef S. Zhao, M. G. P. M. S. Neves, A. C. Tomé, A. M. S. Silva, J. A. S. Cavaleiro, M. R. M. Domingues, and A. J. F. Correia, Tetrahedron Lett., 2005, 46, 2189; CrossRef S. Ostrowski and P. Wyrębek, Tetrahedron Lett., 2006, 47, 8437; CrossRef (e) 1,3-dipolar cycloaddition: J. A. S. Cavaleiro, M. G. P. M. S. Neves, and A. C. Tomé, Arkivoc, 2003 (xiv), 107; A. C. Tomé, M. G. P. M. S. Neves, and J. A. S. Cavaleiro, J. Porphyrins Phthalocyanines, 2009, 13, 408; CrossRef (f) cyclopropanation via carbene addition: H. J. Callot, F. Metz, and C. Piechocki, Tetrahedron, 1982, 38, 2365; and refs. cited therein. CrossRef
3. S. Ostrowski, P. Wyrębek, and A. Mikus, Heterocycles, 2006, 68, 885. CrossRef
4. They readily enter into dimerization to give furoxans, see: (a) T. Mukaiyama and T. Hoshino, J. Am. Chem. Soc., 1960, 82, 5339; CrossRef (b) G. Bryant-Bachman and L. E. Strom, J. Org. Chem., 1963, 28, 1150. CrossRef
5. (a) X. Li, J. Zhuang, Y. Li, H. Liu, S. Wang, and D. Zhu, Tetrahedron Lett., 2005, 46, 1555; CrossRef X. Liu, Y. Feng, X. Chen, F. Li, and X. Li, Synlett, 2005, 1030. In the recent past two additional papers have appeared; CrossRef (c) X. Liu, Y. Feng, X. Hu, and X. Li, Synthesis, 2005, 3632; CrossRef (d) M. M. Ebrahim, M. Moreau, and M. O. Senge, Heterocycles, 2011, 83, 627. CrossRef
6. R. Paul and S. Tchelitcheff, Bull. Soc. Chim. France, 1962, 7, 2215.
7. (a) A. M. G. Silva, A. C. Tomé, M. G. P. M. S. Neves, J. A. S. Cavaleiro, and C. O. Kappe, Tetrahedron Lett., 2005, 46, 4723; CrossRef (b) E. A. Makarova, G. V. Korolyova, O. L. Tok, and E. A. Lukyanets, J. Porphyrins Phthalocyanines, 2000, 4, 525. CrossRef
8. For example: (a) reaction with HI: G. W. Perold and F. V. K. von Reiche, J. Am. Chem. Soc., 1957, 79, 465; CrossRef (b) transfor¬mation to oximes: V. Jäger and H. Grund, Angew. Chem., 1976, 88, 27; CrossRef (c) reduction: A. P. Kozikowsky and M. Adamczyk, Tetrahedron Lett., 1982, 23, 3123; CrossRef (d) thermal cleavage: R. G. Shotter, D. Sesardic, and P. H. Wright, Tetrahedron, 1975, 31, 3069; CrossRef (e) via quarternization: S. Shatzmiller, E. Shalom, R. Lidor, and E. Tartkovski, Liebigs Ann. Chem., 1983, 897; CrossRef S. Kwiatkowski and S. Ostrowski, Bull. Soc. Chim. Belg., 1993, 102, 259; CrossRef For review see: R. Gandolfig and P. Grünanger, In The Chemistry of Heterocyclic Compounds; Part 2: ”Isoxazoles”; P. Grünanger and P. Vita-Finzi, Eds.; An Interscience, John Wiley & Sons, Inc.: New York – Chichester – Weinheim – Brisbane – Singapore – Toronto, 1999; pp. 671-739.
9. (a) T. Peters Jr., All about albumin; Academic Press: San Diego, CA, 1992; (b) D.-K. Han, G. Jin, B. Y. Chung, and Y. K. Shim, Bull Korean Chem. Soc., 1999, 20, 137.
10. A. Hassner and K. M. L. Rai, Synthesis, 1989, 57. CrossRef
11. (a) Ch. Matt, A. Wagner, and Ch. Mioskowski, J. Org. Chem., 1997, 62, 234; CrossRef (b) C. Egan, M. Clery, A. F. Hegarty, and A. J. Welch, J. Chem. Soc., Perkin Trans. 2, 1999, 249; (c) Ch. Matt, A. Gissot, A. Wagner, and Ch. Mioskowski, Tetrahedron Lett., 2000, 41, 1191. CrossRef
12. In the reaction of highly active meso-tetrakis(pentafluorophenyl)porphyrin with nitropropane (POCl3, NEt3, toluene, reflux, 16 h) we detected small amounts of the desired chlorin 6 by MS (<5%), however it was contaminated with products of SNAr substitution of fluorine in pentafluorophenyl rings. Data for product 6: MS (FD), m/z (% rel. int.): 1048 (6), 1047 (28), 1046 (56), 1045 (100) [isotope M+]. HR-MS (FD) calcd for C47H15N5OF20 [M+]: 1045.0957, found: 1045.0999. The molecular formula was also confirmed by comparing the theoretical and experimental isotope patterns for the M+ ion (C47H15N5OF20); it was found to be identical within the experimental error limits.
13. Y. Basel and A. Hassner, Synthesis, 1997, 309. CrossRef