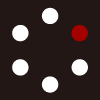
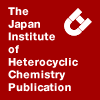
HETEROCYCLES
An International Journal for Reviews and Communications in Heterocyclic ChemistryWeb Edition ISSN: 1881-0942
Published online by The Japan Institute of Heterocyclic Chemistry
e-Journal
Full Text HTML
Received, 7th September, 2011, Accepted, 6th October, 2011, Published online, 12th October, 2011.
DOI: 10.3987/COM-11-12357
■ Dihydroazamacrosphelides: Synthesis and Apoptosis Inducing Activities
Kenji Sugimoto, Yuta Kobayashi, Takashi Kondo, Naoki Toyooka, Hideo Nemoto, and Yuji Matsuya*
Graduate School of Medicines and Pharmaceutical Sciences for Research, University of Toyama, Sugitani 2630, Toyama, 930-0194, Japan
Abstract
Dihydroazamacrosphelides, which were aza-analogues of 3-epi macrosphelide L, were prepared according to ring-closing metathesis strategy. Their biological assay revealed that the oxidized diketo-analogues showed stronger apoptosis inducing activity than their dihydroxy precusors.INTRODUCTION
Macrosphelides are well known 16-membered macrolides having potent biological activities such as a dose-dependent inhibition against the adhesion of HL-60 to human-umbilical-vein endothelial cells (HUVECs) without an affection on various mammalian cell lines nor microorgasms in vitro, and an immunosuppressant activity equal to that of rapamycin.1 Considering those novel biological profiles as a new lead for pharmaceuticals, macrosphelides have kindled much interest of organic chemists as attractive synthetic targets and numerous creative total syntheses of natural congeners have been established2–9 since the pioneering work by Omura and Smith.2 We are also interested in their potency as an antitumor agent and developed total syntheses of macrosphelides A (1), B (2), E (7), I (4), and L (5) utilizing ring-closing metathesis (RCM) strategy.7 Furthermore, based on the RCM strategy, we furnished natural-type and ‘non-natural’ macrosphelides to reveal that the diketomacrosphelide 3, oxidized derivative of macrosphelides A (1) and B (2), could induce apoptotic cell death more effectively than their parent compounds. Notably, among the macrosphelide analogues we reported, 12,13-dihydro-diketomacrosphelide 6 derived from macrosphelides I (4) and L (5) exhibited comparable potency with 3.7c,10 On the other hand, novel aza-macrosphelides (azaMSs), which were designed by the replacement of the lactone oxygen with nitrogen, were found to be a potential apoptosis-inducer and N-phenylethyl 10-aza congener (8; R = phenylethyl, X = Y = O) demonstrated the best biological property.10g With these findings, next we focused our attention on the aza-analogues of dihydromacrosphelides, 12,13-dihydro-10-azaMSs 9 and herein we describe the syntheses of such derivatives and their biological activities toward human lymphoma cell (U937) by the measurement of early apoptosis and secondary necrosis.
RESULTS AND DISCUSSION
Considering our previous result, we started the syntheses of N-phenylethyl-aza-analogues of dihydromacrosphelides as shown in Scheme 1.
According to our preceding synthesis of aza-MSs,10g RCM precursor 13 was assembled from the corresponding homoallyl alcohol 10, β-hydroxycarboxylic acid 11, β-amino acid derivative 12, and acryloyl chloride, and then RCM with Grubbs’ catalyst second generation successfully afforded the macrolactam 14. Catalytic hydrogenation with Rh/Al2O3 selectively reduced the olefin adjacent to the free hydroxyl group to furnish the desired MEM-protected 12,13-dihydro-10-azaMS 15 quantitatively. The conformational analysis of 14 with Monte Carlo simulation well rationalized the observed chemoselectivity as that the outer periferal face of the olefin adjacent to the MEM ether was hindered with the MEM group to be prevented from the approach of the reagents (Figure 2). Acidic deacetalization11 followed by Dess-Martin oxidation established the corresponding diketo-10-azaMS 17 with further oxidized 18.10g
Next, we made an attempt on the preparation of the N-benzyl-12,13-dihydro-10-azaMSs under similar way. The MEM ether 1910g was exposed to several typical hydrogenation conditions, however, desired dihydro-derivative was not observed at all (Scheme 2).
Because the 1H NMR analysis on the resultant crude mixture indicated that debenzylation of the substrate was inevitable under the conditions for hydrogenation of this olefin, we gave up this late stage hydrogenation and turned our attention on the alternative approach using the carboxylic acid 237c for the saturated C11–O16 fragment as depicted in Scheme 3. The synthesis commenced with the protected allyl alcohol 21,10g an intermediate of formerly reported our macrosphelides syntheses. The protecting group on the nitrogen was switched to benzyl group then dehydrative condensation of resulting amine 22 with the carboxylic acid 237c furnished the C7–O16 segment 24. After twice of the selective desilylation and esterification sequence, the corresponding RCM precursor 30 was uneventfully prepared. In the presence of Grubbs’ catalyst second generation, the requisite macrolactam 31 was smoothly constructed with satisfactory yield. Acidic deacetalization afforded N-benzyl-12,13-dihydro-azaMS 32 then finally Dess-Martin oxidation established desired diketone 33.
Having prepared novel four analogues of dihydro-azaMSs, their apoptosis-inducing activities were evaluated by the assay on human lymphoma cell line (U937) (Table 1).10
The cells were exposed to 10 μM of each candidate and incubated for 12 h, then the percentages of the early apoptotic and the secondary necrotic cell death were measured with flow cytometry. Disappointingly, the dihydroderivatives 16 and 32 never induced the desired apoptotic cell death. However, despite the activities were lower than that of previously reported aza-MS 8, diketo-derivatives 17 and 33 were found to be significant apoptosis-inducers on lymphoma cells with relatively higher secondary necrosis inducing activity.
In conclusion, we proved that RCM approach could be applicable for the frequent preparation of various types of macrosphelide analogues. Additionally, the chemoselective reduction of the functionally resembled two olefins in our azamacrocycle was found to be possible because of its structural rigidity. Furthermore, it was revealed that the structurally more flexible dihydro-analogue of the aza-MSs induced not only the desired apoptosis but also the undesired necrosis. Now the relevance between the biological activities and the three dimensional structures is under investigation through conformational analyses on natural and designed macrosphelides.
EXPERIMENTAL
Materials were obtained from commercial suppliers and used without further purification unless otherwise noted. Anhydrous THF was purchased from Kanto Chemical Co., Inc. Anhydrous Et2O, CH2Cl2, dioxane, DMF, DMSO, toluene, and MeCN were purchased from Wako Pure Chemical Industries. Anhydrous MeOH, EtOH, iPrOH, NEt3 were dried and distilled according to the standard protocols. Otherwise noted, all reactions were performed using oven-dried glassware, sealed with a rubber septum under a slight positive pressure of argon. Flash column chromatography was carried out using Kanto silica gel 60N (spherical, neutral, 40–50 µm). Analytical TLC was performed on Merck 60 F254 glass plates precoated with a 0.25 mm thickness of silica gel. Optical rotations were measured on a JASCO DIP-1000 digital polarimeter. All melting points were determined on Yanagimoto micro melting point apparatus and uncorrected. IR spectra were measured on a JNM FT/IR-660 spectrometer. NMR spectra were measured on a VARIAN Gemini 300 spectrometer. For 1H spectra, chemical shifts are expressed in parts par million (ppm) downfield from internal tetramethylsilane (δ 0) or relative internal CHCl3 (δ 7.26). The following abbreviations are used for spin multiplicity: s = singlet, d = doublet, t = triplet, q = quartet, m = multiplet, and br = broad. J values are given in hertz. For 13C spectra, chemical shifts are expressed in ppm, relative to the central line of a triplet at 77.0 ppm for internal CDCl3. Mass spectra were recorded on a JEOL D-200, JEOL JMS-GCmate II, SHIMADZU GCMS-QP 500, or JEOL AX 505 spectrometer.
(3S,6E,8R,9S,14R,15S)-14-Hydroxy-8-(2-methoxyethoxy)methoxy-3,9,15-trimethyl-10-phenethyl-10- aza-4,16-dioxa-6-cyclohexadecene-1,5,11-trione (15). Under an H2 atmosphere, to a solution of cyclic compound 14 (26.7 mg, 0.0500 mmol) in EtOH (2.0 mL) was added Rh/Al2O3 (20.0 mg), and the mixture was stirred for 1 h at rt. The reaction mixture was diluted with CH2Cl2, and filtered through Celite. The filtrate was concentrated in vacuo, and the residue was identified as the 15 (26.8 mg, 0.0500 mmol, quant.) by 1H-NMR, and used for next reaction without further purification.
1H-NMR (300 MHz, CDCl3): δ 7.32–7.17 (5H, m), 6.67 (1H, dd, J = 15.7 Hz, 9.1 Hz), 6.01 (1H, d, J = 15.7 Hz), 5.39–5.11 (1H, m), 5.00–4.80 (2H, m), 4.79–4.60 (2H, m), 3.80–3.51 (5H, m), 3.43–3.23 (5H, m), 3.09–3.01 (1H, m), 2.99–2.76 (2H, m), 2.63–2.24 (5H, m), 2.19–2.04 (1H, m), 1.55–1.20 (9H, m); 13C-NMR (75 MHz, CDCl3): δ 172.95, 171.21, 164.66, 145.36, 137.59, 128.65, 128.56, 126.64, 124.50, 94.12, 78.17, 74.44, 73.62, 71.58, 68.60, 67.29, 61.55, 59.04, 53.65, 41.53, 35.89, 30.92, 28.61, 20.13, 15.93, 15.68; IR (CHCl3): 3432 cm–1 (O–H), 1732 cm–1 (C=O), 1627 cm–1 (C=O); MS (EI) m/z 535 (M+); HRMS (EI) Calcd for C28H41NO9: 535.2781 (M+), found: 535.2768; [α]D24 –12.2 (c 1.375, CHCl3).
(3S,6E,8R,9S,14R,15S)-8,14-Dihydroxy-3,9,15-trimethyl-10-phenethyl-10-aza-4,16-dioxa-6-cyclohexa-decene-1,5,11-trione (16). Under an Ar atmosphere, to a solution of the MEM ether 15 (26.8 mg, 0.0500 mmol) in CH2Cl2 (0.50 mL) was added TFA (0.50 mL) at 0 °C, and the mixture was stirred for 4 h at rt. The reaction mixture was concentrated in vacuo, and the residue was purified by column chromatography on silica gel (CH2Cl2:AcOEt = 3:2) to afford the diol 16 (6.4 mg, 0.0143 mmol, 29%, mixture of rotamers) as a colorless oil.
1H-NMR (300 MHz, CDCl3): δ 7.34–7.08 (5H, br), 6.91–6.59 (3H, br), 6.11–5.94 (1H, br), 5.44–5.28 (1H, br), 5.16–4.68 (1H, br), 4.36–4.08 (1H, br), 3.82–3.10 (4H, br), 2.86–2.18 (8H, br), 1.59–1.25 (9H, br); IR (CHCl3): 3418 cm–1 (O–H), 1731 cm–1 (C=O), 1715 cm–1 (C=O), 1647 cm–1 (C=O); MS (EI) m/z 447 (M+); HRMS (EI) Calcd for C24H33NO7: 447.2257 (M+), found: 447.2241; [α]D24 +33.3 (c 0.270, CHCl3).
(3S,6E,9S,15S)-3,9,15-Trimethyl-10-phenethyl-10-aza-4,16-dioxa-6-cyclohexadecene-1,5,8,11,14-pentaone (17). Under an Ar atmosphere, to a solution of the diol 16 (7.2 mg, 0.0160 mmol) in CH2Cl2 (1.0 mL) was added Dess-Martin periodinane (33.9 mg, 0.080 mmol) at rt and the mixture was stirred for 1 h. The reaction mixture was directly purified by column chromatography on silica gel (hexane:AcOEt = 7:1) to afford the diketone 17 (4.0 mg, 0.0090 mmol, 56%, as a colorless oil) and diketone 1810g (2.2 mg, 0.0050 mmol, 31%, as a colorless oil).
17: 1H-NMR (300 MHz, CDCl3): δ 7.34–7.18 (5H, m), 7.03 (1H, d, J = 15.8 Hz), 6.54 (1H, d, J = 15.8 Hz), 5.41–5.35 (1H, m), 5.22 (1H, q, J = 7.1 Hz), 4.67–4.65 (1H, m), 3.82–3.72 (1H, m), 3.52–3.34 (1H, m), 3.03–2.87 (3H, m), 2.69–2.35 (5H, m), 1.50 (3H, d, J = 7.1 Hz), 1.45 (3H, d, J = 6.3 Hz), 1.42 (3H, d, J = 6.6 Hz); 13C-NMR (75 MHz, CDCl3): δ 204.79, 197.25, 171.96, 169.52, 164.77, 137.33, 137.12, 130.36, 128.79, 128.50, 126.91, 74.93, 68.44, 60.24, 49.17, 40.69, 36.86, 29.79, 26.54, 20.02, 16.34, 13.29; IR (CHCl3): 1742 cm–1 (C=O), 1719 cm–1 (C=O), 1636 cm–1 (C=O); MS (EI) m/z 443 (M+); HRMS (EI) Calcd for C24H29NO7: 443.1944 (M+), found: 443.1970; [α]D24 –129.4 (c 0.185, CHCl3).
N-Benzyl-N-[(1S,2R)-2-tert-butyldiphenylsilyloxy-1-methyl-3-butenyl]amine (22). Under an Ar atmosphere, to a solution of the TBDPS ether 2110g (439.7 mg, 1.00 mmol) in CH2Cl2 (10.0 mL) was dropped TFA (5.0 mL), and the mixture was stirred for 15 min at 0 °C. The reaction was quenched with sat. NaHCO3 aq., and the mixture was extracted with CH2Cl2. The organic layer was dried over MgSO4, filtered, and concentrated in vacuo.
To a solution of the residue in MeOH/AcOH (10:1, 10.0 mL) were added benzaldehyde (0.10 mL, 1.50 mmol) and 2-picoline·BH3 (106.9 mg, 1.00 mmol) at 0 °C under an Ar atmosphere, and the mixture was stirred for 30 min at rt. The reaction was quenched with 10% HCl aq., and the mixture was alkalinized with sat. NaHCO3 aq., extracted with CH2Cl2. The organic layer was dried over MgSO4, filtered, and concentrated in vacuo. The resulting oil was purified by column chromatography on silica gel (hexane:AcOEt = 5:2) to afford the amine 22 (335.2 mg, 0.78 mmol, 78% in 2 steps) as a colorless oil.
1H-NMR (300 MHz, CDCl3): δ 7.64–7.61 (4H, m), 7.45–7.21 (11H, m), 5.89 (1H, ddd, J = 17.3 Hz, 10.4 Hz, 6.9 Hz), 5.03 (1H, ddd, J = 10.4 Hz, 1.1 Hz, 0.8 Hz), 4.92 (1H, ddd, J = 17.3 Hz, 1.1 Hz, 1.1 Hz), 4.13–4.09 (1H, m), 3.80 (1H, d, J = 13.4 Hz), 3.62 (1H, d, J = 13.4 Hz), 2.75–2.67 (1H, m), 1.90 (1H, br), 1.07 (9H, s), 0.97 (3H, d, J = 6.6 Hz); 13C-NMR (75 MHz, CDCl3): δ 140.41, 137.16, 135.93, 135.76, 134.68, 133.83, 133.68, 129.50, 129.42, 128.21, 127.98, 127.58, 127.40, 127.22, 126.54, 116.54, 78.15, 56.76, 51.16, 27.15, 19.47, 15.94; IR (CHCl3): 3421 cm–1 (N–H), 1112 cm–1 (C–N); MS (EI) m/z 372 (M+–57); HRMS (EI) Calcd for C24H26NOSi: 372.1784 (M+–57), found: 372.1791; [α]D24 +49.1 (c 0.865, CHCl3).
(4R,5S)-N-Benzyl-N-[(1S,2R)-2-tert-butyldiphenylsilyloxy-1-methyl-3-butenyl]-5-tert-butyldimethyl-silyloxy-4-(2-methoxyethoxy)methoxyhexanamide (24). Under an Ar atmosphere, to a solution of the amine 22 (344.6 mg, 0.80 mmol) and carboxylic acid 237c (396.1 mg, 1.13 mmol) in CH2Cl2 (7.5 mL) were added EDC·HCl (216.6 mg, 1.13 mmol) and DMAP (18.3 mg, 0.15 mmol) at 0 °C, and the mixture was stirred for 24 h at rt. The reaction mixture was concentrated in vacuo, and the residue was purified by column chromatography on silica gel (CH2Cl2:AcOEt = 15:1) to afford the amide 24 (588.6 mg, 0.77 mmol, 99%, mixture of rotamers) as a colorless oil.
1H-NMR (300 MHz, CDCl3): δ 7.70–7.60 (4H, m), 7.48–7.14 (9H, m), 7.11–7.03 (2H, m), 5.82–5.59 (1H, m), 4.91–4.39 (6H, m), 4.24–3.97 (2H, m), 3.87–3.67 (2H, m), 3.57–3.45 (1H, m), 3.43–3.23 (6H, m), 2.59–2.16 (1H, m), 2.00–1.45 (3H, m), 1.15–1.01 (15H, m), 0.89–0.82 (9H, m), 0.06–0.03 (6H, m); IR (CHCl3): 1648 cm–1 (C=O); MS (EI) m/z 761 (M+); HRMS (EI) Calcd for C44H67NO6Si2: 761.4507 (M+), found: 761.4475; [α]D25 +1.3 (c 0.595, CHCl3).
(4R,5S)-N-Benzyl-N-[(1S,2R)-2-tert-butyldiphenylsilyloxy-1-methyl-3-butenyl]-5-hydroxy-4-(2-methoxyethoxy)methoxyhexanamide (25). A solution of the TBS ether 24 (571.6 mg, 0.75 mmol) in AcOH/THF/H2O (3:1:1, 3.0 mL) was stirred for 12 h at 60 °C. The reaction mixture was diluted with Et2O, washed with sat. NaHCO3 aq., dried over MgSO4, filtered, and concentrated in vacuo. The residue was purified by column chromatography on silica gel (CH2Cl2:AcOEt = 10:1) to give the alcohol 25 (346.2 mg, 0.53 mmol, 71%, mixture of rotamers) as a colorless oil.
1H-NMR (300 MHz, CDCl3): δ 7.71–7.61 (4H, m), 7.48–7.15 (9H, m), 7.06–7.04 (2H, m), 5.83–5.60 (1H, m), 4.93–4.86 (2H, m), 4.81–4.39 (4H, m), 4.17–3.99 (2H, m), 3.86–3.33 (9H, m), 2.19–2.09 (1H, m), 1.96–1.65 (4H, m), 1.16–1.06 (15H, m); IR (CHCl3): 3445 cm–1 (O–H), 1634 cm–1 (C=O); MS (EI) m/z 647 (M+); HRMS (EI) Calcd for C38H53NO6Si: 647.3642 (M+), found: 647.3607; [α]D25 –60.2 (c 0.740, CHCl3).
(1S,2R)-4-{N-Benzyl-N-[(1S,2R)-2-tert-butyldiphenylsilyloxy-1-methyl-3-butenyl]carbamoyl}-2-(2-methoxyethoxy)methoxy-1-methylbutyl (S)-3-tert-Butyldimethylsilyloxybutyrate (27). Under an Ar atmosphere, to a solution of the carboxylic acid 2610g (147.4 mg, 0.675 mmol) in toluene (4.5 mL) were added NEt3 (0.11 mL, 0.81 mmol) and 2,4,6-trichlorobenzoyl chloride (0.11 mL, 0.675 mmol) at 0 °C, and the mixture was stirred for 30 min at the same temperature. To this solution were added a solution of the alcohol 25 (324.0 mg, 0.50 mmol) in toluene (4.5 mL) and DMAP (82.5 mg, 0.675 mmol), and the mixture was stirred for 1 h at rt. The reaction mixture was diluted with Et2O, washed with 10% HCl aq. and sat. NaHCO3 aq., dried over MgSO4, filtered, and concentrated in vacuo. The residue was purified by column chromatography on silica gel (CH2Cl2:AcOEt = 10:1) to give the ester 27 (381.0 mg, 0.45 mmol, 90%, mixture of rotamers) as a colorless oil.
1H-NMR (300 MHz, CDCl3): δ 7.71–7.60 (4H, m), 7.47–7.11 (9H, m), 7.04–7.01 (2H, m), 5.85–5.61 (1H, m), 4.94–4.89 (2H, m), 4.84–4.39 (4H, m), 4.31–3.97 (3H, m), 3.76–3.69 (1H, m), 3.56–3.42 (2H, m), 3.40–3.21 (6H, m), 2.54–2.08 (4H, m), 1.99–1.64 (2H, m), 1.27–1.12 (9H, m), 1.06 (9H, s), 0.89–0.80 (9H, m), 0.09–0.03 (6H, m); IR (CHCl3): 1734 cm–1 (C=O), 1648 cm–1 (C=O); MS (EI) m/z 790 (M+–57); HRMS (EI) Calcd for C44H64NO8Si2: 790.4170 (M+–57), found: 790.4208; [α]D24 –0.09 (c 0.705, CHCl3).
(1S,2R)-4-{N-Benzyl-N-[(1S,2R)-2-tert-butyldiphenylsilyloxy-1-methyl-3-butenyl]carbamoyl}-2-(2-methoxyethoxy)methoxy-1-methylbutyl (S)-3-Hydroxybutyrate (28). A solution of the TBS ether 27 (373.2 mg, 0.44 mmol) in AcOH/THF/H2O (3:1:1, 1.75 mL) was stirred for 12 h at 50 °C. The reaction mixture was diluted with Et2O, washed with sat. NaHCO3 aq., dried over MgSO4, filtered, and concentrated in vacuo. The residue was purified by column chromatography on silica gel (Et2O only) to give the alcohol 28 (264.3 mg, 0.36 mmol, 82%, mixture of rotamers) as a colorless oil.
1H-NMR (300 MHz, CDCl3): δ 7.71–7.60 (4H, m), 7.46–7.14 (9H, m), 7.04–7.02 (2H, m), 5.84–5.61 (1H, m), 5.14–4.71 (3H, m), 4.65–4.38 (4H, m), 4.19–3.97 (2H, m), 3.74–3.71 (1H, m), 3.56–3.25 (6H, m), 2.73 (1H, br), 2.57–2.29 (3H, m), 2.20–1.67 (5H, m), 1.25–1.10 (9H, m), 1.06 (9H, s); IR (CHCl3): 3446 cm–1 (O–H), 1732 cm–1 (C=O), 1646 cm–1 (C=O); MS (EI) m/z 733 (M+); HRMS (EI) Calcd for C42H59NO8Si: 733.4010 (M+), found: 733.3970; [α]D24 –2.4 (c 0.540, CHCl3).
(1S,2R)-4-{N-Benzyl-N-[(1S,2R)-2-tert-butyldiphenylsilyloxy-1-methyl-3-butenyl]carbamoyl}-2-(2-methoxyethoxy)methoxy-1-methylbutyl (S)-3-Acryloyloxybutyrate (29). Under an Ar atmosphere, to a solution of the alcohol 28 (256.9 mg, 0.35 mmol) in CH2Cl2 (3.5 mL) were added diisopropylethylamine (0.15 mL, 0.84 mmol) and acryloyl chloride (56.9 µL, 0.700 mmol) at 0 °C, and the mixture was stirred for 1 h at rt. The reaction mixture was diluted with CH2Cl2, successively washed with 10% HCl aq. and sat. NaHCO3 aq., dried over MgSO4, filtered, and concentrated in vacuo. The residue was purified by column chromatography on silica gel (CH2Cl2:AcOEt = 6:1) to give the ester 29 (229.6 mg, 0.29 mmol, 83%, mixture of rotamers) as a colorless oil.
1H-NMR (300 MHz, CDCl3): δ 7.71–7.60 (4H, m), 7.46–7.14 (9H, m), 7.04–7.01 (2H, m), 6.40–6.32 (1H, m), 6.12–6.00 (1H, m), 5.84–5.58 (2H, m), 5.36–5.27 (1H, m), 5.10–4.70 (3H, m), 4.64–4.38 (4H, m), 4.19–3.97 (2H, m), 3.74–3.67 (1H, m), 3.55–3.47 (1H, m), 3.40–3.24 (6H, m), 2.71–2.42 (2H, m), 2.19–2.08 (1H, m), 1.91–1.62 (3H, m), 1.36–1.28 (3H, m), 1.21–1.14 (6H, m), 1.05 (9H, s); IR (CHCl3): 1728 cm–1 (C=O), 1645 cm–1 (C=O); MS (EI) m/z 787 (M+); HRMS (EI) Calcd for C45H61NO9Si: 787.4115 (M+), found: 787.4145; [α]D24 –6.1 (c 0.720, CHCl3).
(1S,2R)-4-{N-Benzyl-N-[(1S,2R)-2-hydroxy-1-methyl-3-butenyl]carbamoyl}-2-(2-methoxyethoxy)methoxy-1-methylbutyl (S)-3-Acryloyloxybutyrate (30). Under an Ar atmosphere, to a solution of the TBDPS ether 29 (220.6 mg, 0.280 mmol) in THF (2.2 mL) were added AcOH (0.38 mL, 6.7 mmol) and TBAF (1.0 M solution in THF, 5.6 mL, 5.6 mmol), and the mixture was stirred for 2.5 days at rt. The reaction mixture was diluted with Et2O, washed with sat. NaHCO3 aq., dried over MgSO4, filtered, and concentrated in vacuo. The residue was purified by column chromatography on silica gel (CH2Cl2:AcOEt = 5:1) to give the alcohol 30 (126.3 mg, 0.23 mmol, 82%) as a colorless oil.
1H-NMR (300 MHz, CDCl3): δ 7.38–7.18 (5H, m), 6.37 (1H, dd, J = 17.3 Hz, 1.4 Hz), 6.06 (1H, dd, J = 17.3 Hz, 10.2 Hz), 5.78 (1H, dd, J = 10.2 Hz, 1.6 Hz), 5.74 (1H, ddd, J = 17.3 Hz, 10.4 Hz, 6.9 Hz), 5.35–5.28 (1H, m), 5.21 (1H, dd, J = 17.3 Hz, 1.6 Hz), 5.08 (1H, dd, J = 10.4 Hz, 1.4 Hz), 5.07–5.00 (1H, m), 4.74 (1H, d, J = 7.0 Hz), 4.64 (1H, d, J = 17.6 Hz), 4.62 (1H, d, J = 7.0 Hz), 4.50 (1H, d, J = 17.6 Hz), 4.35–4.33 (1H, m), 3.87–3.80 (1H, m), 3.66–3.61 (1H, m), 3.57–3.52 (2H, m), 3.50–3.35 (2H, m), 3.32 (3H, s), 2.63 (1H, dd, J = 15.4 Hz, 7.7 Hz), 2.51 (1H, dd, J = 15.4 Hz, 5.5 Hz), 2.49–2.40 (2H, m), 1.99–1.84 (1H, m), 1.81–1.74 (1H, m), 1.31 (3H, d, J = 6.3 Hz), 1.18 (3H, d, J = 6.9 Hz), 1.16 (3H, d, J = 5.5 Hz); 13C-NMR (75 MHz, CDCl3): δ 174.66, 169.34, 165.07, 138.26, 137.04, 130.70, 128.79, 128.40, 127.48, 126.19, 115.46, 94.99, 78.10, 75.53, 71.71, 71.66, 67.50, 67.41, 59.54, 59.04, 51.74, 41.11, 30.39, 25.58, 19.90, 15.21, 11.09; IR (CHCl3): 3432 cm–1 (O–H), 1727 cm–1 (C=O), 1632 cm–1 (C=O); MS (EI) m/z 549 (M+); HRMS (EI) Calcd for C29H43NO9: 549.2938 (M+), found: 549.2894; [α]D25 +5.4 (c 0.765, CHCl3).
(3S,6E,8R,9S,14R,15S)-10-Benzyl-8-hydroxy-14-(2-methoxyethoxy)methoxy-3,9,15-trimethyl-10-aza- 4,16-dioxa-6-cyclohexadecene-1,5,11-trione (31). Under an Ar atmosphere, to a solution of the cyclization precursor 30 (82.4 mg, 0.150 mmol) in CH2Cl2 (150 mL) was added Grubbs’ catalyst second generation (25.5 mg, 0.0300 mmol), and the mixture was stirred for 3 h at rt. The reaction mixture was concentrated in vacuo, and the residue was purified by column chromatography on silica gel (CH2Cl2:AcOEt = 3:1) to give the macrocyclic compound 31 (49.5 mg, 0.095 mmol, 63%) as a colorless oil.
1H-NMR (300 MHz, CDCl3): δ 7.37–7.21 (3H, m), 7.18–7.15 (2H, m), 6.50 (1H, dd, J = 15.7 Hz, 8.2 Hz), 5.90 (1H, d, J = 15.7 Hz), 5.41–5.28 (1H, m), 5.02–4.90 (2H, m), 4.80 (1H, d, J = 6.9 Hz), 4.68 (1H, d, J = 6.9 Hz), 4.32 (2H, s), 3.77–3.46 (5H, m) 3.39–3.28 (4H, m), 2.75 (1H, br), 2.63–2.25 (4H, m), 2.00–1.88 (1H, m), 1.75–1.66 (1H, m), 1.39 (3H, d, J = 6.6 Hz), 1.31 (3H, d, J = 6.3 Hz), 1.22 (3H, d, J = 6.3 Hz); 13C-NMR (75 MHz, CDCl3): δ 172.78, 169.77, 165.18, 147.87, 135.99, 128.66, 127.72, 127.59, 122.03, 94.36, 77.62, 72.96, 71.58, 69.87, 67.88, 67.37, 61.53, 59.01, 53.94, 41.29, 29.01, 25.23, 19.87, 16.65, 14.85; IR (CHCl3): 3433 cm–1 (O–H), 1732 cm–1 (C=O), 1641 cm–1 (C=O); MS (EI) m/z 521 (M+); HRMS (EI) Calcd for C27H39NO9: 521.2625 (M+), found: 521.2624.
(3S,6E,8R,9S,14R,15S)-10-Benzyl-8,14-dihydroxy-3,9,15-trimethyl-10-aza-4,16-dioxa-6-cyclohexadecene-1,5,11-trione (32). Under an Ar atmosphere, to the solution of the MEM ether 31 (14.1 mg,0.0270 mmol) in 0.0270 mmol) in CH2Cl2 (0.27 mL) was added TFA (0.27 mL) at 0 °C, and the mixture was stirred for 1 h at rt. The reaction mixture was concentrated in vacuo, and the residue was purified by column chromatography on silica gel (CH2Cl2:AcOEt = 3:2) to give the diol 32 (10.3 mg, 0.0238 mmol, 88%) as a colorless solid.
1H-NMR (300 MHz, CDCl3): δ 7.38–7.26 (3H, br), 7.18–7.16 (2H, br), 6.76–6.63 (1H, br), 6.00–5.95 (1H, br), 5.45–5.38 (1H, br), 4.99–4.62 (2H, br), 4.42–4.35 (2H, br), 3.49–3.31 (2H, br), 3.22–2.91 (3H, br), 2.66–2.38 (5H, br), 1.34–1.25 (9H, br); 13C-NMR (75 MHz, CDCl3): δ 170.76, 169.77, 165.02, 147.75, 128.78, 128.37, 127.42, 122.66, 74.31, 73.42, 73.07, 68.64, 53.75, 41.75, 31.10, 29.74, 29.29, 28.83, 20.31, 15.24, 15.02; IR (CHCl3): 3409 cm–1 (O–H), 1730 cm–1 (C=O), 1623 cm–1 (C=O); MS (EI) m/z 433 (M+); HRMS (EI) Calcd for C23H31NO7: 433.2101 (M+), found: 433.2082; [α]D25 +12.6 (c 0.515, CHCl3); mp. 58–61 °C.
(3S,6E,9S,15S)-10-Benzyl-3,9,15-trimethyl-10-aza-4,16-dioxa-6-cyclohexadecene-1,5,8,11,14-pentaone (33). Under an Ar atmosphere, to a solution of the diol 32 (13.0 mg, 0.0300 mmol) in CH2Cl2 (1.0 mL) was added Dess-Martin periodinane (30.5 mg, 0.0720 mmol) at rt, and the mixture was stirred for 1 h. The reaction mixture was directly purified by column chromatography on silica gel (CH2Cl2:AcOEt = 2:1) to give the diketone 33 (10.9 mg, 0.0254 mmol, 85%) as a colorless solid.
1H-NMR (300 MHz, CDCl3): δ 7.42–7.26 (5H, m), 6.98 (1H, d, J = 15.9 Hz), 6.47 (1H, d, J = 15.9 Hz), 5.43–5.38 (1H, m), 5.22 (1H, q, J = 7.1 Hz), 4.91 (1H, d, J = 17.0 Hz), 4.70–4.68 (1H, br), 4.33 (1H, d, J = 17.0 Hz), 3.02–2.93 (1H, m), 2.78–2.43 (5H, m), 1.50 (3H, d, J = 7.1 Hz), 1.47 (3H, d, J = 6.3 Hz), 1.29 (3H, d, J = 6.6 Hz); 13C-NMR (75 MHz, CDCl3): δ 204.79, 197.18, 172.48, 169.56, 164.74, 137.37, 136.48, 130.30, 128.94, 127.92, 126.87, 74.78, 68.41, 59.72, 50.60, 40.73, 33.95, 27.12, 20.08, 16.36, 12.62; IR (CHCl3): 1722 cm–1 (C=O), 1641 cm–1 (C=O), 1632 cm–1 (C=O); MS (EI) m/z 429 (M+); HRMS (EI) Calcd for C23H27NO7: 429.1788 (M+), found: 429.1809; [α]D26 –179.7 (c 0.545, CHCl3); mp. 37–40 °C.
Biological evaluation procedures
Cell line and culture: A human lymphoma cell line, U937, was obtained from the Human Sciences Research Resource Bank (Japan Human Sciences Foundation, Tokyo, Japan) and was maintained in RPMI 1640 medium supplemented with 10% heat-inactivated fetal bovine serum (FBS) at 37 °C in humidified air with 5% CO2.
Apoptosis assay by flow cytometry
Flow cytometry was performed with PI and FITC-labeled annexin V (Immunotech, Marselille, France) to detect phosphatidylserine externalization (on the surface of the cell membrane) as an endopoint indicator of early apoptosis. After treatment of the cells with test compounds, the remaining intact cells were incubated at 37 °C for 12 h, collected, washed with cold phosphate-buffered saline (PBS) at 4 °C, and centrifuged at 500 g for 3 min. FTIC-labeled annexin V (5 μL) and PI (5 μL) were added to the cell suspension (490 μL) and mixed in gently. After incubation at 4 °C for 10 min in the dark, the cells were analyzed by flow cytometry (Epics XL, Beckman-Coulter, Miami, FL).
ACKNOWLEDGEMENTS
This work was supported by the JST with grant ‘A Research for Promoting Technological Seed’ (for Y. M).
References
1. (a) M. Hayashi, Y.-P. Kim, H. Hiraoka, M. Natori, S. Takamatsu, T. Kawakubo, R. Masuma, K. Komiyama, and S. Omura, J. Antibiot., 1995, 48, 1435; (b) S. Takamatsu, Y.-P. Kim, M. Hayashi, H. Hiraoka, M. Natori, K. Komiyama, and S. Omura, J. Antibiot., 1996, 49, 95; (c) S. Takamatsu, H. Hiraoka, Y.-P. Kim, M. Hayashi, M. Natori, K. Komiyama, and S. Omura, J. Antibiot., 1997, 50, 878; (d) A. Fukami, Y. Taniguchi, T. Nakamura, M.-C. Rho, K. Kawaguchi, M. Hayashi, K. Komiyama, and S. Omura, J. Antibiot., 1999, 52, 501; (e) A. Numata, M. Iritani, T. Yamada, K. Minoura, E. Matsumura, T. Yamori, and T. Tsuruo, Tetrahedron Lett., 1997, 38, 8215; CrossRef (f) T. Yamada, M. Iritani, M. Doi, K. Minoura, T. Ito, and A. Numata, J. Chem. Soc., Perkin Trans. 1, 2001, 3046; CrossRef (g) T. Yamada, M. Iritani, K. Minoura, A. Numata, Y. Kobayashi, and Y.-G. Wang, J. Antibiot., 2002, 55, 147; (h) T. Yamada, K. Minoura, R. Tanaka, and A. Numata, J. Antibiot., 2007, 60, 370.
2. (a) T. Sunazuka, T. Hirose, Y. Harigaya, S, Takamatsu, M. Hayashi, K. Komiyama, S. Omura, P. A. Sprengeler, and A. B. Smith, III, J. Am. Chem. Soc., 1997, 119, 10247; CrossRef (b) T. Sunazuka, T. Hirose, N. Chikaraishi, Y. Harigaya, M. Hayashi, K. Komiyama, P. A. Sprengeler, A. B. Smith, III, and S. Omura, Tetrahedron, 2005, 61, 3789. CrossRef
3. (a) Y. Kobayashi, B. G. Kumar, and T. Kurachi, Tetrahedron Lett., 2000, 41, 1559; CrossRef (b) Y. Kobayashi, G. B. Kumar, T. Kurachi, H. P. Acharya, T. Yamazaki, and T. Kitazume, J. Org. Chem., 2001, 66, 2011; CrossRef (c) Y. Kobayashi and H. P. Acharya, Tetrahedron Lett., 2001, 42, 2817; CrossRef (d) Y. Kobayashi and Y.-G. Wang, Tetrahedron Lett., 2002, 43, 4381. CrossRef
4. (a) M. Ono, H. Nakamura, F. Konno, and H. Akita, Tetrahedron: Asymmetry, 2000, 11, 2753; CrossRef (b) H. Nakamura, M. Ono, Y. Shiba, and H. Akita, Tetrahedron: Asymmetry, 2002, 13, 705; CrossRef (c) H. Nakamura, M. Ono, M. Makino, and H. Akita, Heterocycles, 2002, 57, 327. CrossRef
5. G. V. M. Sharma and C. C. Mouli, Tetrahedron Lett., 2002, 43, 9159. CrossRef
6. (a) T. Takahashi, S.-i. Kusaka, T. Doi, T. Sunazuka, and S. Omura, Angew. Chem., Int. Ed., 2003, 42, 5230; CrossRef (b) S.-i. Kusaka, S. Dohi, T. Doi, and T. Takahashi, Tetrahedron Lett., 2003, 44, 8857. CrossRef
7. (a) Y. Matsuya, T. Kawaguchi, and H. Nemoto, Org. Lett., 2003, 5, 2939; CrossRef (b) T. Kawaguchi, N. Funamori, Y. Matsuya, and H. Nemoto, J. Org. Chem., 2004, 69, 505; CrossRef (c) Y. Matsuya, Y. Kobayashi, T. Kawaguchi, A. Hori, Y. Watanabe, K. Ishihara, K. Ahmed, Z.-L. Wei, D.-Y. Yu, Q.-L. Zhao, T. Kondo, and H. Nemoto, Chem. Eur. J. 2009, 15, 5799. CrossRef
8. (a) S. M. Paek, S.-Y. Seo, S.-H. Kim, J.-W. Jung, Y.-S. Lee, J.-K. Jung, and Y.-G. Suh, Org. Lett., 2005, 7, 3159; CrossRef (b) S.-M. Paek, H. Yun, N.-J. Kim, J.-W. Jung, D.-J. Chang, S. Lee, J. Yoo, H.-J. Park, and Y.-G. Suh, J. Org. Chem., 2009, 74, 554. CrossRef
9. K. R. Prasad and P. Gutala, Tetrahedron, 2011, 67, 4514. CrossRef
10. For recent examples of our medicinal research on macrosphelides, see: (a) Y. Matsuya, T. Kawaguchi, H. Nemoto, H. Nozaki, and H. Hamada, Heterocycles, 2003, 59, 481; CrossRef (b) Y. Matsuya, T. Kawaguchi, and H. Nemoto, Heterocycles, 2003, 61, 39; CrossRef (c) Y. Matsuya, K. Ishihara, N. Funamori, T. Kawaguchi, and H. Nemoto, Heterocycles, 2003, 61, 59; CrossRef (d) K. Ishihara, T. Kawaguchi, Y. Matsuya, H. Sakurai, I. Saiki, and H. Nemoto, Eur. J. Org. Chem., 2004, 3973; CrossRef (e) Y. Matsuya and H. Nemoto, Heterocycles, 2005, 65, 1741; CrossRef (f) Y. Matsuya, T. Kawaguchi, K. Ishihara, K. Ahmed, Q.-L. Zhao, T. Kondo, and H. Nemoto, Org. Lett., 2006, 8, 4609; CrossRef (g) K. Sugimoto, Y. Kobayashi, A. Hori, T. Kondo, N. Toyooka, H. Nemoto, and Y. Matsuya, Tetrahedron, 2011, 67, 7681. CrossRef
11. The reaction conditions were not optimized. This acidic deacetalization afforded the desired diol 16 with unidentifiable, highly polar material. Since the elongation of the reaction time (9 h) gave the similar result with 24% yield and analogous N-Bn 14-MEM ether 31 was uneventfully deprotected by TFA without the decomposition of diol 32, this unsuccessful result might to be caused by the instability of the substrate 15 under the acidic condition, not by the further undesired reaction of the diol 16.