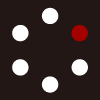
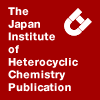
HETEROCYCLES
An International Journal for Reviews and Communications in Heterocyclic ChemistryWeb Edition ISSN: 1881-0942
Published online by The Japan Institute of Heterocyclic Chemistry
e-Journal
Full Text HTML
Received, 15th September, 2011, Accepted, 14th October, 2011, Published online, 21st October, 2011.
DOI: 10.3987/COM-11-12361
■ Studies on the Synthesis of Nicotinamide Nucleoside and Nucleotide Analogues and Their Inhibitions towards CD38 NADase
Zhe Chen, Anna Ka Yee Kwong, Zhenjun Yang, Liangren Zhang,* Hon Cheung Lee, and Lihe Zhang
National Laboratory of Natural and Biomimetic Drugs, School of Pharmaceutical Sciences, Peking University, No. 38, Xueyuan Road, Beijing 100083, China
Abstract
Nicotinamide adenine dinucleotide (NAD) analogues inhibit the NADase activity of CD38. In the current study, efficient protocols for the synthesis of substituted-nicotinamide nucleosides and nucleotides were developed. The one-pot phosphorylation–esterification strategy provides a convenient way of obtaining nicotinamide nucleoside phosphodiesters from the corresponding nucleosides. Structure–activity relationship information revealed that replacement of 3’-hydroxy group with F or N3 led to the considerably decrease of activity as compared with ara-F NMN. Phosphodiesterification of nicotinamide nucleosides lowers their inhibitory activities in some extent.INTRODUCTION
Nicotinamide adenine dinucleotide (NAD) analogues are gaining importance in the discovery of therapeutic agents because of their broad spectrum of pharmacological and biological activities. Thiazole-4-carboxamide adenine dinucleotide, benzamide adenine dinucleotide, and mycophenolic adenine dinucleotide have been reported to be potent inhibitors of inosine monophosphate dehydrogenase.1–3 Nicotinamide hypoxanthine dinucleotide and nicotinamide guanine dinucleotide are potent inhibitors of ADP-ribosyl cyclase.4 Carba-NAD has been shown to have inhibitory activities against NAD-dependent histone deacetylase.5 Our research aimed to discover inhibitors of CD38 which is the main mammalian ADP-ribosyl cyclase and signaling enzyme responsible for catalyzing the synthesis of two Ca2+ messengers, i.e., cyclic ADP-ribose (cADPR) and nicotinic acid adenine dinucleotide phosphate (NAADP).6 CD38 inhibitors can be classified as covalent and noncovalent types based on their modes of binding to CD38. The former often displays stronger activity than the latter. Among the covalent inhibitors (1, 2) (Figure 1),7–9 fluorinated NAD analogues are the most intensively studied because derivatization with fluorine enhances the activity, liphophilicity, metabolic stability, and so on.10–13 1-(2’-Deoxy-2’-fluoro-β-D-arabinofuranosyl) nicotinamide 5’-phosphate (2a, ara-F NMN) inhibits the NADase activity of CD38 in the nanomolar range.8 2’-Deoxy-2’-fluoro-NAD (2b, ara-F NAD) was used to capture the enzymatic reaction intermediates of mutated CD38 and label the extracellular domain of human CD38.14,15
The synthesis of nucleotides that carry positively charged constituents still remains challenging even though many strategies have been developed to assemble nucleotides using either P(III) or P(V) reagents in nucleic acid chemistry.16–19 The existing strategies often resulted in tedious purification work and cleavage of the anomeric C-N glycosidic bond. Oppenheimer et al. firstly synthesized the 2’-substituted arabino-NAD analogues using 1,2:5,6-di-O-isopropylidine-α-D-allofuranose as a starting material, which allowed the stereospecific introduction of the fluoro and azido substituents.20 Recently, Sauve et al. described a strategy for diastereocontrolled electrophilic fluorination to synthesize 2’-fluoro-substituted NAD analogues.21 Esterification of nucleotide enhances its lipophilicity and enzymatic stability. The esterification of ribonofuranosyl nicotinamide mononucleotide was investigated by using N,N’-dicyclohexylcarbodiimide (DCC)/4-dimethylaminopyridine (DMAP)22 or the 2,4,6-triisopropyl- benzenesulfonyl chloride (TIPS-Cl)/pyridine23 coupling system. However, the research of the phosphoesters of nicotinamide nucleoside either on the structural diversity or on the biological property is far from well studied.
In this study, for providing compounds to investigate the effect of 3’-substitution on biological activity, the synthesis of 3’-substituted NAD analogues (3) is reported for the first time. A simple and efficient method of preparing nicotinamide nucleosides phosphodiesters (20-23) is provided, in which phosphorylation and esterification of nucleosides were carried out in a one-pot reaction. These structurally diverse NAD analogues were used to study the inhibition to NADase of CD38. Findings in this study help in providing compounds to elucidate the structure-activity relationships of NAD analogues and investigate the physiological properties of human CD38.
RESULTS AND DISCUSSION
Treatment of 424 with trifluoromethanesulfonic anhydride (Tf2O) in pyridine at -10 °C followed by fluorination gave fluoroxylose 5 in 64% in two steps. The fluorination using tetrabutylammonium fluoride (TBAF) afforded fluororibose via SN2 reaction and resulted in the change of configuration of C-3. This fluorinating condition is milder than those using HF, DAST or other fluorinating reagents. The configuration of C-3 was confirmed by 1H and 19F NMR spectra, which are in accordance with the previous reports.24 After hydrolysis of 5 with aqueous formic acid and the following acetylation, an anomeric mixture (α:β = 1:1) of compound 6 was obtained. Condensation of 6 with trimethylsilylated nicotinamide,25 and the subsequent deprotection of the condensation product with NH3/MeOH provided 3’-deoxy-3’-fluoro-β-D-xylofuranosylnicotinamide nucleoside (7) (Scheme 1). 3’-β-D-azido substituted ribofuranosylnicotinamide nucleoside (9) was synthesized via directed coupling of 826 with trimethylsilylated nicotinamide, followed by deprotection using a similar procedure as that of nucleoside 7. Treatment of ketone 1027 with DAST afforded difluorodeoxyfuranoside 11 in 44% yield. Condensation of difluorosugar 11 with trimethylsilylated nicotinamide in the presence of TMSOTf afforded the corresponding nucleoside which was followed by deprotection using K2CO3/MeOH to provide 2’,3’-dideoxy-3’,3’-difluoro-D-glycero-pentofuranosylnicotinamide nucleoside (12) in 47% yield (Scheme 1). Compound 12 was obtained as an anomeric mixture that could be separated into the α- and β-isomers (α:β = 1:1). 3’-Azido-2’,3’-dideoxy-D-thero-furanosylnicotinamide nucleoside (15), an anomeric mixture (α:β = 1:1), was obtained using a similar procedure starting with azidofuranoside 14 which was prepared from the azidation of 13.28 Nucleoside 15 was not stable and decomposed during purification, which resulted in low yields (33%).
The phosphorylation of nicotinamide nucleoside analogues (7, 9, 12 and 15) was performed based on a modification of the procedure developed by Yoshikawa, which is generally used to synthesize nucleoside 5’-monophosphates (Scheme 2).29 These nucleosides were dissolved in trimethyl phosphate and reacted with phosphoryl chloride, and then hydrolyzed to yield the corresponding mononucleotides 16-19 in 76-80% yield. The synthesis of ara-F NMN (2a) and its nucleoside moiety, 2’-deoxy-2’-fluoro-β-D-arabinofuranosylnicotinamide (3a), was carried out according to the reported method.20
Mononucleotides can be esterified by the DCC/DMAP22 or the TIPS-Cl/pyridine23 strategy. However, the process often requires a long reaction time and results in low yields. In the current study, a one-pot strategy was used to synthesize nicotinamide nucleoside phosphodiesters. The nucleoside 9 was phosphorylated at 5’-OH with POCl3 at 0 °C. Afterward, butanol or benzyl alcohol was added to the reaction mixture, which was then stirred for 1-6 h, and then quenched with water and neutralized with 1 N NaOH, the corresponding nicotinamide nucleoside phosphodiesters 20 and 21 were obtained, respectively. The butyl and benzyl esters (22, 23) could be also prepared under the similar conditions in 56-62% yield.
The synthesized compounds have been tested for their inhibitory activities towards CD38 NADase (Table 1). The results show that nicotinamide nucleosides (2a, 3a, 7, 9, 12, 15) do not have or only have very weak inhibitory activities. Phosphorylation of nicotinamide nucleosides improves activities as compounds 16, 17 and 19 show, but in general the nicotinamide nucleoside monophosphates with modification at 3’-position only give very weak inhibition to CD38 NADase. By comparing with ara-F NMN (2a), it clearly shows that the modification at 3’-position will dramatically lower inhibitory activity. Esterification of nicotinamide nucleotide also leads to obvious decrease of inhibitory activity (20-23). Considering the increase of stability towards the hydrolysis by phosphatase and the increase of membrane permeability after esterification, it may provide probe for investigating the physiological property of CD38.
CONCLUSION
In summary, efficient protocols for the synthesis of nicotinamide 3’-substituted nuleosides and nucleotides have been developed. The one-pot phosphorylation–esterification strategy provides a convenient way of producing nicotinamide nucleoside phosphodiesters from the corresponding nucleosides. Biological investigation indicates that the 3’-hydroxy group of D-ribose is crucial for the inhibitory activity. Phosphodiesterification of nicotinamide nucleosides obviously lowers their inhibitory activities. The newly synthesized compounds and related findings help to understand the structure–activity relationship of NAD analogues for the inhibition to CD38 NADase. The active compounds may be used as probes to elucidate the physiological properties of CD38.
EXPERIMENTAL
HR-ESI-MS (electrospray ionization) were performed with Bruker BIFLEX III. 1HNMR and 13C NMR were recorded with a Bruker AVANCE III 400 or a JEOLAL300 spectrometer, CDCl3 or D2O was used as a solvent. Chemical shifts are reported in parts permillion downfield from TMS (1H and 13C). 31P NMR spectra were recorded at room temperature by use of JEOL AL300 spectrometer (122 MHz); Orthophosphoric acid (85%) was used as an external standard. 19F NMR spectra were recorded on a Varian VXR-500 spectrometer (470 MHz). Chemical shifts of 19F NMR are reported in ppm with reference to CF3CO2H (-75.6 relative to CF3) as an external standard. Most of the target compounds were purified twice on an Alltech preparative C18 reversed-phase column (2.2 × 25 cm) using a Gilson HPLC buffer system: H2O/MeOH, 0.1% CF3CO2H (pH 2-3).
5-O-Benzoyl-3-deoxy-3-fluoro-1, 2-O-(isopropylidene)-α-D-xylofuranose (5)
Compound 4 (5.50 g, 18.69 mmol) was dissolved in 50 mL DCM which contains pyridine (6.30 mL, 7.53 mmol) and cooled to -30 °C. Tf2O (6.32 mL, 37.38 mmol) was added dropwise and then was stirred at -10 °C for 2 h. 100 mL DCM was added to the reaction solution, and was washed with dilute HCl solution and saturated aqueous NaHCO3 solution, respectively. After dried with anhydrous MgSO4 and evaporated, the residue was dissolved in 80 mL THF and then TBAF⋅3H2O (11.79 g, 37.37 mmol) was stirred. The mixture was stirred at 60 °C for 10 h, and then was evaporated and purified by silica gel column chromatography (PE:EtOAc = 3:1) to give compound 5 as a colorless oil (3.54 g, 64% yield). 1H NMR (400 MHz, CDCl3) δ 8.06 (d, 2H, J = 8.4 Hz, Ar-H), 7.59-7.56 (m, 1H, Ar-H), 7.45 (t, 2H, J = 8.4 Hz, Ar-H), 6.04 (d, 1H, J1,2 = 3.6 Hz, H-1), 5.05 (dd, 1H, J3,4 = 2.0, J3,F = 50.8 Hz, H-3), 4.76-4.70 (m, 1H, H-2), 4.65-4.52 (m, 3H, H-4, H-5), 1.51 (s, 3H, -CH3), 1.34 (s, 3H, -CH3). 13C NMR (75 MHz, CDCl3) δ 166.1, 133.2, 129.8, 129.6, 128.4, 112.4, 105.1, 94.0 (d, JC-3-F = 138.0 Hz, C-3), 82.5 (d, JC-4-F = 24.0 Hz, C-4), 77.7 (d, JC-2-F = 14.3 Hz, C-2), 61.3 (C-5), 26.7, 26.2. 19F NMR (470 MHz, CDCl3, CF3Ph): δ -209.7 (m, CF). ESI: [M + Na]+ 319.1, [M + K]+ 335.0. MS (ESI-TOF+) m/z 319.06 (M + Na)+, 335.03 (M + K)+.
1,2-Di-O-acetyl-5-O-benzoyl-3-deoxy-3-fluoro-α,β-D-xylofuranose (6)
Compound 5 (1.87 g, 5.50 mmol) in 88% HCO2H (10 mL) was reacted at 80 °C for 2 h, and then the solvent was removed under reduced pressure. The residue was dissolved in pyridine (10 mL), and was added Ac2O (5.20 mL, 55 mmol) dropwise at 0 °C. The reaction mixture was stirred at rt for 24 h and then cooled to 0 °C. The mixture was quenched by MeOH (1 mL) and partitioned between H2O and DCM. The organic layer was washed with cold dilute HCl, saturated aqueous NaHCO3, and brine, respectively, dried over Na2SO4, filtered and evaporated. The residue was purified by silica gel column chromatography (20–30% EtOAc in PE) to give compound 6 as a colorless oil (1.74 g, 81%). 1H NMR (400 MHz, CDCl3) δ 8.07-8.03 (m, 2H, Ar-H), 7.58 (t, J = 7.2 Hz, 1H, Ar-H), 7.46 (t, J = 7.2 Hz, 2H, Ar-H), 6.57-6.20 (m, 1H, H-1), 5.57-5.08 (m, 2H, H-2, H-3), 4.69-4.49 (m, 3H, H-4, H-5), 2.13-2.05 (m, 6H, -COMe).
1-(3’-Deoxy-3’-fluoro-β-D-xylofuranosyl) nicotinamide (7)
To a solution of dry nicotinamide (0.49 g, 4.01 mmol) in HMDS (4 mL) was added TMSCl (1.03 mL, 8.02 mmol) under argon, and the mixture was stirred at 120 °C for approximately 6 h, then the reaction mixture was evaporated to dryness at 90 °C under vacuum. A solution of 6 (1.36 g, 4.01 mmol) in DCE (6 mL) was added to the above residue at 45 °C and then TMSOTf (1.85 mL, 8.02 mmol) was added portionwise over 5 min. The resulting mixture was stirred at 45 °C for 2 h, and then was cooled to 0 °C and MeOH (2 mL) was added. The mixture was concentrated in vacuo, and then dissolved in methanolic ammonium solution (30 mL) and stirred at -10 °C for 24 h. After filtration of the solution, the filtrate was neutralized with cold dilute HCl and evaporated.The residue was partitioned between CHCl3 and H2O. The aqueous layer was evaporated, and the residue was dissolved in 0.1% CF3CO2H buffer (1.0 mL), which was applied to a C18 reversed-phase column (2.2 × 25 cm). The column was developed using a linear gradient of 0–40% MeOH in CF3CO2H buffer (pH 3.5) within 38 min to give 7 as a colorless oil. Yield: 62%. 1H NMR (400 MHz, D2O) δ 9.39 (s, 1H, H-2), 9.17 (d, 1H, J5,6 = 6.0 Hz, H-6), 8.97 (d, 1H, J4,5 = 8.0 Hz, H-4), 8.27 (dd, 1H, H-5), 6.36 (s, 1H, H-1’), 5.15 (d, 1H, J3’,F = 50.4 Hz, H-3’), 4.75 (d, 1H, J4’,F = 11.6 Hz, H-4’), 4.67-4.56 (m, 1H, H-2’), 3.93-3.91 (m, 2H, H-5’). 13C NMR (100 MHz, D2O) δ 162.3, 145.1, 142.2, 141.0, 133.8, 128.1, 100.8 (C-1’), 94.5 (d, JC-3’-F = 181.0 Hz, C-3’), 86.0 (d, JC-4’-F = 7.2 Hz, C-4’), 79.9 (d, JC-2’-F = 10.8 Hz, C-2’), 57.7 (d, JC-5’-F = 3.6 Hz, C-5’). 19F NMR (470 MHz, D2O, CF3CO2H): δ -201.1 (m, C-F). HRMS (ESI-TOF+) calcd for C11H14N2O4F (M+), 257.0932; found, 257.0928.
1-(3’-Azido-3’-deoxy-β-D-ribofuranosyl) nicotinamide (9)
9 was synthesized by a procedure similar to that for 7, colorless oil. Yield: 62%. 1H NMR (400 MHz, D2O) δ 9.48 (s, 1H, H-2), 9.14 (d, 1H, J5,6 = 6.4 Hz, H-6), 8.87 (d, 1H, J4,5 = 8.0 Hz, H-4), 8.16 (dd, 1H, H-5), 6.15 (d, 1H, J1’,2’ = 4.4 Hz, H-1’), 4.67 (t, 1H, J2’,1’ = 4.4 Hz, H-2’), 4.41-4.38 (m, 1H, H-3’), 4.22 (t, 1H, J = 5.2 Hz, H-4’), 3.96 (dd, 1H, H-5’, J = 13.2 Hz, H-5’), 3.78 (dd, 1H, H-5’). 13C NMR (100 MHz, D2O) δ 166.1, 146.3, 143.1, 140.9, 134.5, 128.9, 100.1, 86.2, 78.2, 61.4, 60.7; HRMS (ESI-TOF+) calcd for C11H14N5O4 (M + H)+, 280.1040; found, 280.1037.
Methyl 5-O-benzoyl-2,3-dideoxy-3,3-difluoro-α,β-D-glycero-pentofuranoside (11)
To a solution of compound 10 (6.90 g, 27.6 mmol) in dry DCM (120 mL) were added DAST (15 mL, 113.2 mmol). The reaction mixture was stirred under reflux for 2 days and then cooled to 0 °C. The mixture was added to saturated aqueous NH4Cl portionwise and stirred. Layers were separated, and the aqueous solution was extracted with DCM. The combined organic layers were washed with a saturated aqueous solution of NaHCO3 and then with brine, dried over Na2SO4, and concentrated to dryness. The residue was purified by silica gel column (10–15% EtOAc in PE) to give compound 11 as a colorless oil. Yield: 44%. 1H NMR (400 MHz, CDCl3) δ 8.07 (m, 2H, Ar-H), 7.56 (m, 1H, Ar-H), 7.44 (m, 2H, Ar-H), 5.16 (t, 1H, H-1), 4.62-4.41 (m, 3H, H-4, H-5), 3.42 and 3.40 (2×s, 3H, -OCH3), 2.74-2.41 (m, 2H, H-2). MS (ESI-TOF+) m/z 295.12 (M + Na)+.
1-(2’, 3’-Dideoxy-3’,3’-difluoro-α,β-D-glycero-pentofuranosyl) nicotinamide (12)
12 was synthesized by a procedure similar to that for 7, colorless oil. Yield: 47%. 12(α): 1H NMR (400 MHz, D2O) δ 9.46 (s, 1H, H-2), 9.14 (t, 1H, J5,6 = 6.4 Hz, H-6), 8.85 (d, 1H, J4,5 = 8.0 Hz, H-4), 8.14 (dd, 1H, H-5), 6.62 (dd, 1H, J1’,2’ = 4.8, J1’,F = 6.8 Hz, H-1’), 4.53-4.46 (m, 1H, H-4’), 3.98-3.89 (m, 2H, H-5’), 3.48-3.35 (m, 1H, H-2’), 3.00-2.88 (m, 1H, H-2’). 13C NMR (100 MHz, D2O) δ 166.2, 146.3, 143.5, 141.5, 134.4, 128.9, 94.9 (d, JC-1’-F = 5.0 Hz, C-1’), 83.8 (t, JC-4’-F = 25.0 Hz, C-4’), 58.3 (C-5’), 42.3 (t, JC-2’-F = 25.0 Hz, C-2’). HRMS (ESI-TOF+) calcd for C11H13N2O3F2 (M+), 259.0889; found, 259.0895. 12(β): 1H NMR (400 MHz, D2O) δ 9.29 (s, 1H, H-2), 9.06 (d, 1H, J5,6 = 6.0 Hz, H-6), 8.85 (d, 1H, J4,5 = 8.0 Hz, H-4), 8.16 (dd, 1H, H-5), 6.74 (d, 1H, J = 7.2 Hz, H-1’), 4.80-4.76 (m, 1H, H-4’), 3.88-3.79 (m, 2H, H-5’), 3.46-3.31 (m, 1H, H-2’), 3.08-3.00 (m, 1H, H-2’). 13C NMR (100 MHz, D2O) δ 166.1, 146.0, 142.8, 140.6, 134.5, 129.0, 96.0 (d, JC-1’-F = 8.0 Hz, C-1’), 84.8 (dd, JC-4’-F = 30.0, 25.0 Hz, C-4’), 59.1 (t, JC-5’-F = 5.0 Hz, C-5’), 42.7 (t, JC-2’-F = 25.0 Hz, C-2’). HRMS (ESI-TOF+) calcd for C11H13N2O3F2 (M+), 259.0888; found, 259.0886. 19F NMR (470 MHz, D2O, CF3CO2H): δ -93.9 and -115.7 (m, C-F).
Methyl 3-azido-5-O-benzoyl-2,3-dideoxy-α,β-D-threo-pentofuranoside (14)
To a solution of compound 13 (4.50 g, 13.62 mmol) in dry DMF (150 mL) was added NaN3 (4.43 g, 68.1 mmol). The reaction mixture was stirred at 100 °C for 6 h and then cooled to rt. The mixture was filtered and the filtrate was evaporated to dryness. The residue was purified by silica gel column (10–30% EtOAc in PE) to give compound 14 as a yellow syrup. Yield: 90%. 14α: 1H NMR (400 MHz, CDCl3) δ 8.09-8.03 (m, 2H, Ar-H), 7.57 (t, 1H, J = 7.2 Hz, Ar-H), 7.45 (m, 2H, J = 7.6 Hz, Ar-H), 5.19 (dd, 1H, J = 2.8, 5.2 Hz, H-1), 4.60-4.50 (m, 2H, H-3, H-4), 4.39 (dd, 1H, J = 5.2 Hz, 10.8 Hz, H-5), 4.27-4.23 (m, 1H, H-5), 3.38 (s, 3H, -OCH3), 2.39-2.25 (m, 2H, H-2). MS (ESI- TOF+) m/z 300.1 (M + Na)+. 14β: 1H NMR (400 MHz, CDCl3) δ 8.07 (t, 2H, J = 7.2 Hz), 7.57 (m, 1H, J = 7.6 Hz), 7.45 (t, 2H, J = 7.6 Hz), 5.08 (t, 1H, J = 4.8 Hz), 4.62-4.51 (m, 2H), 4.44 (dd, 1H, J = 5.6, 12.4 Hz), 4.31-4.28 (m, 1H), 3.40 (s, 3H), 2.39-2.42 (m, 2H). 13C NMR (100 MHz, D2O) δ 166.2, 133.1, 129.9, 129.7, 128.4, 128.4, 104.5, 79.3, 64.5, 60.7, 55.4, 38.5. MS (ESI-TOF+) m/z 300.1 (M + Na)+.
1-(3’-Azido-2’,3’-deoxy-α,β-D-threo-pentofuranosyl) nicotinamide (15)
15 was synthesized by a procedure similar to that for 12, colorless oil. Yield: 33%. 15α: 1H NMR (400 MHz, D2O) δ 9.37 (s, 1H, H-2), 9.12 (d, 1H, J5,6 = 6.0 Hz, H-6), 8.81 (d, 1H, J4,5 = 8.0 Hz, H-4), 8.11 (dd, 1H, H-5), 6.47 (t, 1H, J1’,2’ = 6.0 Hz, H-1’), 4.51-4.46 (m, 2H, H-3’, H-4’), 4.00-3.99 (m, 2H, H-5’), 3.11-3.04 (m, 1H, H-2’), 2.73-2.69 (m, 1H, H-2’). 13C NMR (100 MHz, D2O) δ 163.3, 145.5, 143.0, 141.0, 134.0, 128.5, 97.3, 87.0, 60.7, 60.2, 41.1. 15β: 1H NMR (400 MHz, D2O) δ 9.24 (s, 1H, H-2), 9.00 (d, 1H, J5,6 = 6.0 Hz, H-6), 8.82 (d, 1H, J4,5 = 8.0 Hz, H-4), 8.13 (dd, 1H, H-5), 6.56 (t, 1H, J = 6.0 Hz, H-1’), 4.63-4.60 (m, 1H, H-3’), 4.53-4.49 (m, 1H, H-4’), 3.84-3.83 (m, 2H, H-5’), 3.14-3.08 (m, 1H, H-2’), 2.69-2.62 (m, 1H, H-2’). 13C NMR (100 MHz, D2O) δ 163.5, 145.7, 140.3, 134.4, 129.0, 97.4, 85.4, 61.9, 60.5, 41.3. HRMS (ESI-TOF+) calcd for C11H14N5O3 (M+), 264.1091; found, 264.1092.
General procedure for preparing 2’- and 3’-substituted nicotinamide-5’-mononucleotides (2a, 16-19)
To a solution of 3’-substituted nicotinamide nucleoside 9 (0.36 mmol) in PO(OMe)3 (2.0 mL) at 0 °C was added POCl3 (1.78 mmol), and the mixture was stirred at the same temperature for 1-2 h, then neutralized with 1N NaOH at 0 °C till pH = 7. The crude product was separated from the reaction mixture by addition of Et2O, and the precipitated salt was further purified using a C18 reversed-phase column (2.2 × 25 cm). The column was eluted with a linear gradient of 15-30% MeOH in TFA buffer (pH 3.0) within 40 min to give 16 as colorless foam. Compound 2a was synthesized according to the reported literature.20 Mononucleotides 17, 18 and 19 were synthesized by a procedure similar to that for 16.
1-(3’-Azido-3’-deoxy-β-D-ribofuranosyl) nicotinamide 5’-phosphate (16)
Yield: 80%. 1H NMR (400 MHz, D2O) δ 9.25 (s, 1H, H-2), 9.06 (d, 1H, J5,6 = 6.0 Hz, H-6), 8.81 (d, 1H, J4,5 = 7.6 Hz, H-4), 8.11 (dd, 1H, H-5), 6.05 (d, 1H, J1’,2’ = 5.2 Hz, H-1’), 4.63 (t, 1H, H-2’), 4.46 (d, 1H, J = 2.4 Hz, H-3’), 4.29 (dd, 1H, J = 3.6, 5.6 Hz, H-4’), 4.22-4.17 (m, 1H, H-5’), 4.04-4.00 (m, 1H, H-5’). 13C NMR (100 MHz, D2O) δ 166.1, 146.6, 142.9, 140.3, 134.5, 129.1, 99.9, 85.3, 78.3, 65.1, 62.6. 31P NMR (122 MHz, D2O) δ -0.87(s). HRMS (ESI-TOF+) calcd for C11H14N5O7P (M + H)+, 360.0704; found, 360.0701.
1-(3’-Deoxy-3’-fluoro-β-D-xylofuranosyl) nicotinamide 5’-phosphate (17)
Yield: 79%. 1H NMR (400 MHz, D2O) δ 9.27 (s, 1H, H-2), 9.06 (d, 1H, J5,6 = 6.0 Hz, H-6), 8.78 (d, 1H, J4,5 = 7.6 Hz, H-4), 8.09 (dd, 1H, H-5), 6.23 (s, 1H, H-1’), 5.14 (dt, 1H, J3’,F = 50.4 Hz, H-3’), 4.82-4.73 (m, 2H, H-2’, H-4’), 4.33-4.22 (m, 2H, H-5’). 13C NMR (100 MHz, D2O) δ 166.1, 146.2, 142.9, 140.8, 134.3, 128.9, 100.9 (C-1’), 94.5 (d, JC-3’-F = 183 Hz, C-3’), 84.1 (d, JC-4’-F = 19 Hz, C-4’), 80.3 (d, JC-2’-F = 28.0 Hz, C-2’), 62.5 (d, JC-5’-F = 10 Hz, C-5’). 19F NMR (470 MHz, D2O, CF3CO2H) δ -201.6 (m, C-F). 31P NMR (122 MHz, D2O) δ 0.54 (s). HRMS (ESI- TOF+) calcd for C11H14N2O7F (M + H)+, 337.0595; found, 337.0598.
1-(2’, 3’-Dideoxy-3’,3’-difluoro-α,β-D-glycero-pentofuranosyl) nicotinamide 5’-phosphate (18)
Yield: 71%. 18(α): 1H NMR (400 MHz, D2O) δ 9.34 (s, 1H, H-2), 9.16 (d, 1H, J5,6 = 6.4 Hz, H-6), 8.84 (d, 1H, J4,5 = 8.0 Hz, H-4), 8.16 (dd, 1H, N-5), 6.61 (t, 1H, J = 6.4 Hz, H-1’), 4.71-4.63 (m, 1H, H-4’), 4.22-4.09 (m, 2H, H-5’), 3.52-3.39 (m, 1H, H-2’), 3.07-2.90 (m, 1H, H-2’). 13C NMR (100 MHz, D2O) δ 166.3, 146.4, 143.4, 141.0, 134.4, 128.9, 94.7 (d, JC-1’-F = 5.0 Hz, C-1’), 82.8 (dd, JC-4’-F = 26.0, 9.0 Hz, C-4’), 62.4 (C-5’), 42.2 (t, JC-2’-F = 25.0 Hz, C-2’). 31P NMR (122 MHz, D2O) δ -0.11 (s). 19F NMR (470 MHz, D2O, CF3CO2H): δ -97.9 and -113.5 (m, C-F). HRMS (ESI-TOF+) calcd for C11H13N2O6F2P (M + H)+, 339.0552; found, 339.0550. 18(β): 1H NMR (400 MHz, D2O) δ 9.31 (s, 1H, H-2), 9.08 (d, 1H, J5,6 = 6.0 Hz, H-6), 8.85 (d, 1H, J4,5 = 8.0 Hz, H-4), 8.16 (dd, 1H, H-5), 6.80 (d, 1H, J = 7.6 Hz, H-1’), 4.94 (d, 1H, J = 15.2 Hz, H-4’), 4.11-4.05 (m, 2H, H-5’), 3.51-3.36 (m, 1H, H-2’), 3.09-2.94 (m, 1H, H-2’). 13C NMR (100 MHz, D2O) δ 166.2, 146.1, 142.9, 140.8, 134.5, 129.0, 96.3 (d, JC-1’-F = 10.0 Hz, C-1’), 83.7 ( C-4’), 63.0 (C-5’), 42.6 (t, JC-2’-F = 25.0 Hz, C-2’). 31P NMR (122 MHz, D2O) δ 0.49 (s). 19F NMR (470 MHz, D2O, CF3CO2H): δ -92.3 and -116.1 (m, C-F). HRMS (ESI-TOF+) calcd for C11H13N2O6F2P (M + H)+, 339.0552; found, 339.0546.
1-(3’-Azido-2’,3’-dideoxy-α,β-D-threo-pentofuranosyl) nicotinamide 5’-phosphate (19)
Yield: 78%. 19(α): 1H NMR (400 MHz, D2O) δ 9.32 (s, 1H, H-2), 9.15 (d, 1H, J5,6 = 6.0 Hz, H-6), 8.81 (d, 1H, J4,5 = 7.6 Hz, H-4), 8.11 (dd, 1H, H-5), 6.46 (t, 1H, J = 7.2 Hz, H-1’), 4.60-4.53 (m, 2H, H-3’, H-4’), 4.26-4.21 (m, 2H, H-5’), 3.12-3.05 (m, 1H, H-2’), 2.72-2.68 (m, 1H, H-2’). 13C NMR (100 MHz, D2O) δ 166.3, 145.6, 143.1, 140.8, 134.0, 128.5, 97.2, 85.3, 63.8, 60.6, 40.9. 31P NMR (122 MHz, D2O) δ 0.70 (s). HRMS (ESI-TOF+) calcd for C11H13N5O6P (M + H)+, 344.0755; found, 344.0755. 19(β): 1H NMR (400 MHz, D2O) δ 9.25 (s, 1H, H-2), 9.03 (d, 1H, J5,6 = 6.4 Hz, H-6), 8.82 (d, 1H, J4,5 = 8.4Hz, H-4), 8.13 (dd, 1H, H-5), 6.58 (t, 1H, J1’,2’ = 6.4 Hz, H-1’), 4.78-4.74 (m, 1H, H-3’), 4.58-4.52 (m, 1H, H-4’), 4.10-4.04 (m, 2H, H-5’), 3.14-3.08 (m, 1H, H-2’), 2.68-2.61 (m, 1H, H-2’). 13C NMR (100 MHz, D2O) δ 166.2, 145.8, 142.7, 140.3, 134.4, 129.0, 97.4, 83.9, 64.0, 61.8, 41.1. 31P NMR (243 MHz, D2O) δ 0.76 (s). HRMS (ESI-TOF+) calcd for C11H14N5O6P (M + H)+, 344.0755; found, 344.0754.
1-(2’-Deoxy-2’-fluoro-β-D-arabinofuranosyl) nicotinamide 5’-phosphate (2a)
Yield: 76%. 1H NMR (400 MHz, D2O) δ 9.25 (s, 1H, H-2), 9.08 (t, 1H, J5,6 = 5.6 Hz, H-6 ), 8.81 (d, 1H, J4,5 = 8.0 Hz, H-4), 8.10 (dd, 1H, H-5), 6.56 (dd, 1H, J1’,2’ = 4.4, J1’,F =10.4 Hz, H-1’), 5.36 (dd, 1H, J2’,F = 61.2 Hz, H-2’), 4.43 (dt, 1H, J = 17.2 Hz, H-3’), 4.28-4.27 (m, 1H, H-4’), 4.21-4.04 (m, 2H, H-5’). 13C NMR (100.6 MHz, D2O) δ 165.5, 146.3, 143.6, 141.4, 133.7, 128.2, 94.6 (d, JC-2’-F = 196.0 Hz, C-2’), 94.1 (d, JC-1’-F = 16Hz, C-1’), 83.9 (t, JC-4’-F = 5.0Hz, C-4’), 71.4 (d, JC-3’-F = 25.0Hz, C-3’), 63.4 (C-5’); 19F NMR (D2O, CF3CO2H): δ -199.2 (m, C-F). 31PNMR (243.0 MHz, D2O) δ 0.76 (s). HRMS (ESI- TOF+) calcd for C11H15N2O7FP (M + H+), 337.0601; found (M + H+), 337.0591.
General procedure for preparing 2’- and 3’-substituted nicotinamide nucleoside phosphodiesters (20-23)
To a solution of nicotinamide nucleoside 9 (0.36 mmol) in PO(OMe)3 (2.0 mL) at 0 °C was added POCl3 (1.78 mmol), and the mixture was stirred at the same temperature for 1-2 h. Then corresponding alcohol (3.6 mmol) was added and stirred for 1-2 h, then neutralized with 1N NaOH at 0 °C till pH = 7. The crude product was separated from the reaction mixture by addition of Et2O, and the precipitated salt was further purified using a C18 reversed-phase column (2.2 × 25 cm). The column was eluted with a linear gradient of 15-60% MeOH in TFA buffer (pH 3.0) within 30-80 min to give phosphodiesters (20 and 21) as a colorless foam. Compound 22 and 23 were synthesized using a similar method as 20 and 21.
1-(3’-Azido-3’-deoxy-β-D-ribofuranosyl) nicotinamide 5’-(butyl phosphate) (20)
Yield: 62%. 1H NMR (400 MHz, D2O) δ 9.32 (s, 1H, H-2), 9.13 (d, 1H, J5,6 = 6.4 Hz, H-6), 8.87 (d, 1H, J4,5 = 8.4 Hz, H-4), 8.18 (dd, 1H, H-5), 6.11 (d, 1H, J1’,2’ = 5.6 Hz, H-1’), 4.70 (t, 1H, J = 5.6 Hz, H-2’), 4.52 (t, 1H, J = 2.4 Hz, H-3’), 4.36 (dd, 1H, J = 3.2, 5.2 Hz, H-4’), 4.22-4.18 (m, 1H, H-5’), 4.05-4.00 (m, 1H, H-5’), 3.76-3.71 (m, 2H, -CH2-), 1.46-1.41 (m, 2H, -CH2-), 1.23-1.18 (m, 2H, -CH2-), 0.75 (t, 3H, J = 7.2 Hz, -CH3). 13C NMR (100 MHz, D2O) δ 166.1, 146.7, 142.9, 140.2, 134.5, 129.0, 99.8, 85.6, 78.4, 66.8, 65.0, 62.9, 32.4, 18.8, 13.4. 31P NMR (122 MHz, D2O) δ -0.49 (s). HRMS (ESI-TOF+) calcd for C15H22N5O7P (M+), 416.1330; found, 416.1327.
1-(3’-Azido-3’-deoxy-β-D-ribofuranosyl) nicotinamide 5’-(benzyl phosphate) (21)
Yield: 56%. 1H NMR (400 MHz, D2O) δ 9.18 (s, 1H, H-2), 8.99 (d, 1H, J5,6 = 6.4 Hz, H-6), 8.74 (d, 1H, J4,5 = 8.4 Hz, H-4), 8.03 (dd, 1H, H-5), 7.24-7.20 (m, 5H, Ar-H), 5.99 (d, 1H, J1’,2’ = 5.6 Hz, H-1’), 4.75 (d, 1H, J = 8.8 Hz, PhCH2-), 4.50 (t, 1H, J = 5.6 Hz, H-2’), 4.42-4.39 (m, 1H, H-3’), 4.13-4.09 (m, 1H, H-4’), 3.97-3.94 (m, 1H, H-5’), 3.91-3.87 (m, 1H, H-5’). 13C NMR (100 MHz, D2O) δ 165.9, 146.5, 142.7, 140.0, 134.3, 129.3, 129.0, 128.9, 128.2, 99.7, 85.5, 78.3, 68.4, 65.2, 62.8. 31P NMR (122 MHz, D2O) δ -0.70 (s). HRMS (ESI-TOF+) calcd for C18H20N5O7P (M+), 450.1173; found, 450.1173.
1-(2’-Deoxy-2’-fluoro-β-D-arabinofuranosyl) nicotinamide 5’-(butyl phosphate) (22)
Yield: 61%. 1H NMR (400 MHz, D2O): δ 9.32 (s, 1H, H-2), 9.17 (d, 1H, J5,6 = 6.4 Hz, H-6), 8.87 (d, 1H, J4,5 = 8.0 Hz, H-4), 8.17 (dd, 1H, H-5), 6.62 (dd, 1H, J1’,2’ = 4.4, J1’,F = 12.8 Hz, H-1’), 5.42 (dt, 1H, J2’,F = 50.8 Hz, H-2’), 4.50 (dt, 1H, J = 5.2, 17.2 Hz, H-3’), 4.32-4.31 (m, 1H, H-4’), 4.18-4.17 (m, 1H, H-5’), 4.10-4.04 (m, 1H, H-5’), 3.77 (dd, 2H, J = 6.8, 13.6 Hz, -CH2-), 1.45 (t, 2 H, J = 7.2 Hz, -CH2-), 1.16-1.22 (m, 2H, -CH2-), 0.71 (t, 3H, J = 7.6 Hz, -CH3). 13C NMR (100 MHz, D2O) δ 166.0, 146.9, 144.1, 141.9, 134.2, 128.8, 95.2 (d, JC-2’-F = 197.0 Hz, C-2’), 94.6 (d, JC-1’-F = 15 Hz, C-1’), 84.5 (C-4’), 71.9 (d, JC-3’-F = 24.0 Hz, C-3’), 66.9, 63.6 (C-5’), 32.4, 18.8, 13.4. 31P NMR (122 MHz, D2O): δ 0.05 (s). 19F NMR (470 MHz, D2O, CF3CO2H): δ -198.8 (m, C-F). HRMS (ESI-TOF+): calcd for C15H23N2O7FP (M + H)+ 393.1221, found, 393.1224.
1-(2’-Deoxy-2’-fluoro-β-D-arabinofuranosyl) nicotinamide 5’-(benzyl phosphate) (23)
Yield: 60%. 1H NMR (400 MHz, D2O): δ 9.30 (s, 1H, H-2), 9.12 (t, 1H, J5,6 = 6.4 Hz, H-6), 8.84 (d, 1H, J4,5 = 8.0 Hz, H-4), 8.08 (dd, 1H, H-5), 7.31-7.20 (m, 5H, Ar-H), 6.62 (dd, 1H, J1’,2’ = 4.8, J1’,F = 10.4 Hz, H-1’), 5.45 (dt, 1H, J2’,F = 50.8 Hz, H-2’), 4.86 (d, 2H, J = 7.6 Hz, PhCH2-), 4.42 (dt, 1H, J = 4.8, 17.6 Hz, H-3’), 4.32-4.30 (m, 1H, H-4’), 4.20-4.02 (m, 2H, H-5’). 13C NMR (100 MHz, D2O): δ 165.8, 146.8, 144.0, 141.8, 137.6, 133.9, 129.2, 129.1, 128.8, 128.7, 128.3, 95.1 (d, JC-2’-F = 196.0 Hz, C-2’), 94.5 (d, JC-1’-F = 16.0 Hz, C-1’), 84.6 (C-4’), 71.9 (d, JC-3’-F = 24.0 Hz, C-3’), 68.4, 63.8 (C-5’). 19F NMR (470 MHz, D2O, CF3CO2H): δ -198.7 (m, C-F). 31P NMR (122 MHz, D2O): δ 0.89 (s). HRMS (ESI-TOF+): calcd for C18H20N2O7FP (M + H)+ 427.1065, found 427.1047.
Antagonist Assay
NADase activity was performed by recombinant CD38 prepared by a yeast expression system as described in reference.30 Recombinant CD38 (0.015 µg/mL) and BSA (50 µg/ml) were added to different concentrations of antagonist and incubated for 2 h at room temperature in dark. NAD (2 µM) was added to initiate the reactions and aliquots of samples were collected at T = 0, 4, 8 and 12 mins respectively. Samples were immediately stopped with equal volume of 0.6 M HCl followed by neutralization with 2 times volume of 0.5 M sodium phosphate buffer (pH = 8). The amounts of NAD were measured by cycling assay.31 NADase activity was showed as the percentage of inhibition as compared to control without antagonist.
ACKNOWLEDGEMENTS
This work was supported by the National Natural Sciences Foundation of China (20910094, 90713005, 81172917), the Research Fund for the Doctoral Program of Higher Education (200800010078), and the NSFC/RGC grant N_HKU 722/08 and General Research Fund of Hong Kong: 769107, 768408, 769309, 770610 (to H.C.L.).
References
1. K. Lesiak, K. A. Watanabe, A. Majumdar, M. Seidman, K. Vanderveen, B. M. Goldstein, and K. W. Pankiewicz, J. Med. Chem., 1997, 40, 2533. CrossRef
2. D. Rejman, M. Olesiak, L. Q. Chen, S. E. Patterson, D. Wilson, H. N. Jayaram, L. Hedstrom, and K. W. Pankiewicz, J. Med. Chem., 2006, 49, 5018. CrossRef
3. K. W. Pankiewicz, S. E. Patterson, P. L. Black, H. N. Jayaram, D. Risal, B. M. Goldstein, L. J. Stuyver, and R. F. Schinazi, Curr. Med. Chem., 2004, 11, 887. CrossRef
4. M. E. Migaud, R. L. Pederick, V. C. Bailey, and B. V. L. Potter, Biochemistry, 1999, 38, 9105. CrossRef
5. J. Landry, J. T. Slama, and R. Sternglanz, Biochem. Biophys. Res. Commun., 2000, 278, 685. CrossRef
6. J. B. Yue, W. J. Wei, C. M. C. Lam, Y. J. Zhao, M. Dong, L. R. Zhang, L. H. Zhang, and H. C. Lee, J. Biol. Chem., 2009, 284, 29335. CrossRef
7. A. A. Sauve and V. L. Schramm, Biochemistry, 2002, 41, 8455. CrossRef
8. A. A. Sauve, H. T. Deng, R. H. Angeletti, and V. L. Schramm, J. Am. Chem. Soc., 2000, 122, 7855. CrossRef
9. H. M. Muller-Steffner, O. Malver, L. Hosie, N. J. Oppenheimer, and F. Schuber, J. Biol. Chem., 1992, 267, 9606.
10. J. A. Ma and D. Cahard, Chem. Rev., 2008, 108, PR1. CrossRef
11. W. K. Hagmann, J. Med. Chem., 2008, 51, 4359. CrossRef
12. K. L. Kirk, Org. Proc. Res. Dev., 2008, 12, 305. CrossRef
13. D. O’Hagan, Chem. Soc. Rev., 2008, 37, 308. CrossRef
14. H. M. Zhang, R. Graeff, Z. Chen, L. R. Zhang, L. H. Zhang, H. C. Lee, and Q. Hao, J. Mol. Biol., 2011, 405, 1070. CrossRef
15. H. Jiang, J. Congleton, Q. Liu, P. Merchant, F. Malavasi, H. C. Lee, Q. Hao, A. Yen, and H. N. Lin, J. Am. Chem. Soc., 2009, 131, 1658. CrossRef
16. A. V. Nikolaev, I. V. Botvinko, and A. J. Ross, Carbohydr. Res., 2007, 342, 297. CrossRef
17. J. Stawinski and A. Kraszewski, Acc. Chem. Res., 2002, 35, 952. CrossRef
18. A. Winqvist and R. Strömberg, Eur. J. Org. Chem., 2008, 10, 1705. CrossRef
19. R. Zain and J. Stawinski, J. Org. Chem., 1996, 61, 6617. CrossRef
20. P. R. Sleath, A. L. Handlon, and N. J. Oppenheimer, J. Org. Chem., 1991, 56, 3608. CrossRef
21. Y. Cen and A. A. Sauve, J. Org. Chem., 2009, 74, 5779. CrossRef
22. H. C. Lo, C. Leiva, O. Buriez, J. B. Kerr, M. M. Olmstead, and R. H. Fish, Inorg. Chem., 2001, 40, 6705. CrossRef
23. W. J. Liu, S. G. Wu, S. H. Hou, and Z. B. Zhao, Tetrahedron, 2009, 65, 8378. CrossRef
24. C. J. W. Mort, M. E. Migaud, A. Galione, and B. V. L. Potter, Bioorg. Med. Chem., 2004, 12, 475. CrossRef
25. P. Franchetti, M. Pasqualini, R. Petrelli, M. Ricciutelli, P. Vita, and L. Cappellacci, Bioorg. Med. Chem., 2004, 14, 4655. CrossRef
26. M. W. Chun, H. W. Lee, A. Y. Kim, M. J. Kim, H. O. Kim, Z. G. Gao, K. A. Jacobson, and L. S. Jeong, Nucleosides, Nucleotides Nucleic Acids, 2005, 24, 1119. CrossRef
27. Y. Chong, G. Gumina, J. S. Mathew, R. F. Schinazi, and C. K. Chu, J. Med. Chem., 2003, 46, 3245. CrossRef
28. M. Krecmerova, H. Hrebabecky, M. Masojidkova, and A. Holy, Collect. Czech. Chem. Commun., 1996, 61, 478. CrossRef
29. M. Yoshikawa, T. Kato, and T. Takenishi, Tetrahedron. Lett., 1967, 8, 5065. CrossRef
30. C. B. Munshi, K. B. Fryxell, H. C. Lee, and W. D. Branton, Meth. Enzymol., 1997, 280, 318. CrossRef
31. R. Graeff and H. C. Lee, Biochem. J., 2002, 361, 379.