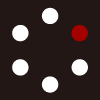
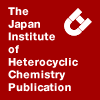
HETEROCYCLES
An International Journal for Reviews and Communications in Heterocyclic ChemistryWeb Edition ISSN: 1881-0942
Published online by The Japan Institute of Heterocyclic Chemistry
e-Journal
Full Text HTML
Received, 3rd October, 2011, Accepted, 21st November, 2011, Published online, 28th November, 2011.
DOI: 10.3987/REV-11-718
■ Recent Advances in the Chemistry and Synthetic Uses of Magnesium Carbenoids
Tsuyoshi Satoh*
Department of Chemistry, Faculty of Science, Tokyo University of Science, Kagurazaka 1-3, Shinjuku-ku, Tokyo 162-8601, Japan
Abstract
Carbenes and carbenoids have long been recognized to be a highly reactive species and are frequently used as intermediates in organic synthesis. However, most of the carbenes and carbenoids are relatively short-lived and are too reactive to control. Recently, the author’s group found that the treatment of aryl α-chloroalkyl sulfoxides and α-chlorovinyl p-tolyl sulfoxides with a Grignard reagent at low temperature gave magnesium carbenoids via the sulfoxide-magnesium exchange reaction. Magnesium carbenoids, cyclopropylmagnesium carbenoids, cyclobutylmagnesium carbenoids, magnesium alkylidene carbenoids, and magnesium β-oxido carbenoids were generated from the corresponding α-chloro sulfoxides with a Grignard reagent at low temperature. The generated magnesium carbenoids were proved to be relatively stable and they showed very interesting reactivity with several nucleophiles to afford many unprecedented new reactions. The present review is written for the purpose of reflecting recent achievements in the areas of chemistry and synthetic application of above-mentioned magnesium carbenoids. This review mainly covers literature from 2006 to late 2011.CONTENTS
1. Introduction
2. Magnesium Carbenoids
2-1. Reaction with N-Lithio Arylamines
2-2. 1,3-Carbon Hydrogen (1,3-CH) Insertion Reaction
2-3. 1,2-CH Insertion and 1,2-Carbon Carbon (1,2-CC) Insertion Reaction
2-4. SN2-type Reaction
3. Cyclopropylmagnesium Carbenoids
3-1. Alkylation of Cyclopropylmagnesium Carbenoids with a Grignard Reagent
3-2. Reaction with N-Lithio Arylamines
3-3. Reaction with O-Lithio Naphthols and Phenols
3-4. Doering-LaFlamme-type Rearrangement
3-5. 1,5-CH Insertion Reaction
4. Cyclobutylmagnesium Carbenoids
4-1. Alkylation of Cyclobutylmagnesium Carbenoids with a Grignard Reagnet
4-2. Reaction of Cyclobutylmagnesium Carbenoids with Lithium α-Sulfonyl Carbanions
4-3. 1,2-CC Insertion Reaction Giving Alkylidenecyclopropanes
5. Magnesium Alkylidene Carbenoids
5-1. Reaction of Magnesium Alkylidene Carbenoids with Nitrogen-containing Heterocycles
5-2. Reaction of Magnesium Alkylidene Carbenoids with Thiphenes and Furans
5-3. Reaction of Magnesium Alkylidene Carbenoids with Lithium Ester Enolates
6. Magnesium β-Oxido Carbenoids
7. Conclusion
1. INTRODUCTION
Synthetic organic chemistry is a base of medicinal chemistry and a variety of chemical sciences. The exploitation of new methods for carbon-carbon and carbon-heteroatom bond-formation is of most importance in synthetic organic chemistry. Carbenes 1 and carbenoids 2a have long been known to be highly reactive carbon species that show a variety of unique reactions such as dimerization to give olefins, addition to olefins to give cyclopropanes, ylide formation, and rearrangement.1 The carbon-hydrogen insertion (CH insertion) reaction and carbon-carbon insertion (CC insertion) reaction are one of the most striking reactions of carbenes and carbenoids.2 The CH and CC insertion reactions are very interesting and highly important for the construction of molecules, because the reactions enable the formation of carbon-carbon bonds between a carbene (or carbenoid) carbon and an unactivated carbon.
As mentioned above, carbenes 1 and carbenoids 2a are quite unique and important reactive carbon species in carbon-carbon and carbon-heteroatom bond-formation; however, those reactive species are not fully used in organic synthesis. The reasons are as follows. One is that the precursors for the generation of carbenes and carbenoids are quite limited. The other is that the reactivity of the species is usually too high to control. In order to solve the problem mentioned above, we used aryl α-haloalkyl (or alkenyl) sulfoxides 2d as the precursors, instead of haloalkanes 2b, and used sulfoxide-magnesium exchange reaction3 for generation of much mild (in contrast to lithium carbenoid 2c) magnesium carbenoids 3. It is worth noting that many aryl α-chloroalkyl (or alkenyl) sulfoxides 2d are quite easily synthesized in high overall yields. Magnesium carbenoids 4, cyclopropylmagnesium carbenoids 5, cyclobutylmagnesium carbenoids 6, magnesium alkylidene carbenoids 7, and magnesium β-oxido carbenoids 8 were generated at low temperature from the corresponding sulfoxides with a Grignard reagent in high to quantitative yields. They were found to be stable usually at below -60 °C for at least 30 min. These magnesium carbenoids have their own unique reactivity and we have found many unprecedented reactions from the reactive species. Recent advances in the chemistry and synthetic uses of magnesium carbenoids are described in this review.
2. MAGNESIUM CARBENOIDS
2-1. Reaction with N-Lithio Arylamines
In 2004, the author’s group reported the generation of magnesium carbenoids 10 from 1-chloroalkyl phenyl sulfoxides 9 with a Grignard reagent in THF at low temperature in quantitative yields (Scheme 1).5 Magnesium carbenoids 10 were proved to be stable at lower than -60 °C for over 30 min. When this reaction was conducted with excess Grignard reagent with warming the temperature of the reaction mixture to -30 °C, magnesium carbenoids 10 reacted with the Grignard reagent to afford alkylated alkylmagnesium chlorides 11, which gave alkanes 12 in good yields by treatment with water. This result showed us that magnesium carbenoid 10 is highly electrophilic species. Treatment of magnesium carbenoids 10 with lithium α-sulfonyl carbanions gave olefins 14 in good to high yields via alkylated intermediates 13.5
Very interesting result was obtained from the reaction of magnesium carbenoid 10 with N-lithio arylamines. Thus, the reaction of 10 with N-lithio N-methylaniline initially afforded N-alkylaniline having carbanion at the α-position 15 in over 80% yield. This carbanion was able to be trapped with ethyl chloroformate to give α-amino acid ester 16 in good yield.6 It is worth noting that α-amino acid esters 16 were synthesized in over 70% yields starting from 9 in one-pot.
In continuation of our interest in the synthesis, including asymmetric synthesis, of α-amino acids, above-mentioned chemistry was extended to intramolecular reaction and the results are summarized in Scheme 2. Thus, treatment of 1-chloroalkyl p-tolyl sulfoxide having a 2-aminophenyl group at the ω-position 17 with i-PrMgCl followed by ethyl chloroformate gave cyclic α-amino acid derivative 19 (n=1 and 2) through α-amino-substituted alkylmagnesium intermediate 18 in up to 68% yield. On the other hand, treatment of 1-chloroalkyl p-tolyl sulfoxide having an arylamino group at the ω-position 20 with i-PrMgCl followed by ethyl chloroformate gave proline derivative 22 (n=1) in 59% yield and pipecolic acid derivative 22 (n=2; 60% yield) through α-amino-substituted alkylmagnesium intermediates 21 (Scheme 1).7
In order to investigate if the above-mentioned procedure could be expanded to an asymmetric synthesis of cyclic α-amino acid derivatives and to investigate the stereochemistry of the key reaction, we tried the procedure with optically active starting material (Scheme 3).8
At first, enantiopure starting material, (1S,RS)-1-chloro-3-(2-methylaminophenyl)propyl p-tolyl sulfoxide 23 was synthesized from oxindole. 1-Chloroalkyl p-tolyl sulfoxide 23 is a crystalline compound and the absolute configuration was determined by X-ray crystallographic analysis. Enantiopure 1-chloroalkyl-p-tolyl sulfoxide 23 was treated with t-BuMgCl followed by i-PrMgCl and ethyl chloroformate as described above to give the optically active pipecolic acid derivative 24 in 66% yield (Scheme 3). The optical yield was mearsured by chiral stationary column and found that it was over 99%. The high enantiomeric excess of product 24 means that almost no racemization occurred throughout the reactions. The absolute configuration of 24 was determined to be (R)-1-methyl-1,2,3,4- tetrahydroquinoline-2-carboxylic acid ethyl ester.8 With absolute configuration of 24 in hand, the whole stereochemistry of the reaction can be inferred as shown in Scheme 3. At first, treatment of 23 with t-BuMgCl resulted in the formation of magnesium amide 25. As sulfoxide-magnesium exchange reaction is known to take place with retention of the configuration of the carbon bearing the sulfinyl group, sulfoxide 25 gave magnesium carbenoid having S-absolute configuration 26. The key intramolecular reaction proceeds with inversion of the carbenoid carbon to afford α-amino carbanion having S-absolute configuration 27. Finally, the anion was trapped by ethyl chloroformate with retention of the configuration of the carbanion to afford the final product 24.
2-2. 1,3-Carbon Hydrogen (1,3-CH) Insertion Reaction
As mentioned in the introduction, CH insertion reaction is quite interesting and useful reaction for construction of molecules.2 Author’s group studied the CH insertion reaction of magnesium carbenoids starting with 1-chloroalkyl phenyl sulfoxides 28 as representative examples (Scheme 4). Thus, 28 was treated with three equiv of i-PrMgCl in THF at -78 °C and the reaction mixture was slowly allowed to warm to 0 °C to result in the formation of cyclopropanes 30 in good to high yields.9 The intermediate of this reaction was proved to be magnesium carbenoid 29 and 1,3-CH insertion reaction exclusively took place between methyl-carbon and the carbenoid carbon to afford 30 as the sole product. As recognized from the results, the 1,3-CH insertion reaction of magnesium carbenoids gives high yield of cyclopropanes and the conditions of the reaction are quite mild.
The same 1,3-CH insertion reaction was found to proceed with sulfoxides 32, which were derived from vinyl sulfoxides 31 with lithium enolate of carboxylic acid tert-butyl ester in high yields.10 Thus, treatment of sulfoxides 32 with 2.5 equiv of i-PrMgCl in toluene resulted in the formation of bicyclo[n.1.0]alkanes 33 in high to quantitative yields. This type of reaction can be realized not only with tert-butyl ester but also with amides.11 Asymmetric synthesis of enantiopure bicyclo[4.1.0]hept-2-ene having acetic ester moiety on the bridgehead carbon 36 was realized from enantiopure α-chloro vinyl sulfoxide 34, which was synthesized from 2-cyclohexenone and (R)-chloromethyl p-tolyl sulfoxide.11 The addition reaction of lithium enolate of tert-butyl acetate to 34 gave (-)-35 in almost quantitative yield with perfect selectivity. Treatment of 35 with i-PrMgCl in toluene resulted in the formation of enantiopure 36 in 90% yield. The 1,3-CH insertion reaction proceeded only on sp3 carbon.
The 1,3-CH insertion of magnesium carbenoids bearing tert-butyl ester at the β-position was found to be highly regiospecific.12 Thus, geometrical isomers of vinyl sulfoxides, E-37 and Z-37, were synthesized form 4-phenyl-2-butanone in high overall yields. Addition reaction of these vinyl sulfoxides with lithium enolate of tert-butyl acetate gave adducts 38 and 39, respectively, in quantitative yields. Adducts 38 and 39 were both single isomers and diastereomers to each other. The adducts were treated with i-PrMgCl in toluene at -78 °C and the temperature of the reaction mixture was slowly allowed to warm to 0 °C. Interestingly, the treatment of 38 with i-PrMgCl gave cyclopropane 42 in 90% yield without any contamination of cyclopropane 43. On the contrary, the same reaction of 39 afforded cyclopropane 43 in 89% yield without any trace of cyclopropane 42. Namely, the 1,3-CH insertion of adduct 38 took place between the carbenoid carbon of 40 and the methyl carbon to give 42. The 1,3-CH insertion reaction of the adduct derived from Z-37 took place between the carbenoid carbon and the methylene carbon to give 43 with high regiospecificity via magnesium carbenoid 41 (Scheme 5). The stereochemistry of 38 and 39, and the mechanism and origin of this regiospecificity are discussed in the original paper.12,13
Asymmetric synthesis of bicyclo[n.1.0]alkanes by the enantioselective 1,3-CH insertion reaction of chiral magnesium carbenoids was also realized (Scheme 6).14 Thus, optically pure 1-chlorovinyl p-tolyl sufoxide 44, derived from cyclohexanone and (R)-chloromethyl p-tolyl sulfoxide, was treated with lithium enolate of tert-butyl acetate. This reaction gave adduct 45 in quantitative yield; however, about 7% yield of diastereomer was observed. The main adduct was separated and treated with i-PrMgCl to afford optically active bicyclo[4.1.0]heptane bearing a tert-butyl acetate moiety 47 in 74% yield. Absolute stereochemistry and enantiomeric excess were determined from 3,6-dibromocarbazole derivative of 47 and ee was found to be 92%. With both absolute configuration of 46 and 47 in hand, it was concluded that the 1,3-CH insertion reaction proceeded between the C-Hb bond. From the results, we concluded that the 1,3-CH insertion reaction of chiral magnesium carbenoid bearing a tert-butyl carboxylate proceeds with high enantioselectivity.
A plausible mechanism of this enantioselective magnesium carbenoid 1,3-CH insertion reaction is proposed as shown in Figure 2.14 Because the sulfoxide-magnesium exchange reaction is known to take place with retention of the configuration of the carbon bearing the sulfinyl group, treatment of 45 with i-PrMgCl gives magnesium carbenoid having S-configuration 46. The magnesium and carbonyl oxygen atom of the tert-butyl ester group make six-membered intermediate, in which the bulky tert-butoxy group would occupy equatorial position. Carbon-hydrogen(Hb) bond of the methylene attacks from rear of the carbon-chlorine bond to give 47. On the other hand, as the carbon-hydrogen(Ha) bond of the methylene is placed almost vertically to the carbon-chlorine bond, the 1,3-CH insertion reaction hardly takes place and Ent-47 is rarely produced.
2-3. 1,2-CH Insertion and 1,2-Carbon Carbon (1,2-CC) Insertion Reaction
As described in section 2-2, magnesium carbenoids generated from 1-chloroalkyl p-tolyl sulfoxides (which were synthesized from ketones and carboxylic acid tert-butyl esters) gave cyclopropanes by the 1,3-CH insertion as the key reaction. In continuation of this chemistry, we investigated the property of magnesium carbenoid 50, generated from 1-chloroalkyl p-tolyl sulfoxides 49, which were derived from aldehydes via 1-chlorovinyl p-tolyl sulfoxide 48, with a Grignard reagent (Scheme 7).15,16 Contrary to our expectation, magnesium carbenoids 50 gave β,γ-unsaturated esters 51 or 52, instead of the cyclopropanes, depending on the nature of the substituent R, in moderate to high yields. This procedure offers a novel method for a synthesis of β,γ-unsaturated esters from aldehydes.
Thus, 1-chloroalkyl p-tolyl sulfoxide 54 derived from 3-phenylpropanal via 53 was treated with i-PrMgBr to afford β,γ-unsaturated ester 56 via 1,2-CH insertion of magnesium carbenoid intermediate 55 as the key reaction in up to 85% yield. In contrast to this, 1-chloroalkyl p-tolyl sulfoxide 58 derived from furfural and 2-formylthiophene via 57 was treated with EtMgCl to afford β,γ-unsaturated esters 59 or 60 via 1,2-CC insertion (rearrangement of aryl group) of magnesium carbenoid intermediates as the key reaction in up to 92% yield.16
In contrast to the 1,3-CH insertion reaction giving bicyclo[n.1.0]alkanes described in section 2-2 (Scheme 4 and 6), magnesium carbenoids 63 generated from 62 (which were synthesized from cyclobutanones through 1-chlorovinyl p-tolyl sulfoxides 61) resulted in 1,2-CC insertion reaction to afford one-carbon ring-expanded cyclopentene derivatives in good to high yields (Scheme 8).17 When enantiopure chloromethyl p-tolyl sulfoxide and carboxylic acid esters were used in this procedure, optically active cyclopentenes 64 were obtained in high ee.18
1,2-CC insertion reaction was observed in the reaction of magnesium carbenoids bearing an alkynyl group at the β-position (Scheme 9).19,20 Thus, 1-chlorovinyl p-tolyl sulfoxide 65, derived from 1,4-cyclohexanedione mono ethylene acetal, was treated with lithium acetylides to give adducts 66 in moderate to good yields. Treatment of this adducts with i-PrMgCl or cyclopentylmagnesium chloride resulted in the formation of conjugated enynes 68 in up to 99% yields by the 1,2-CC insertion reaction (migration of the acetylenic groups) of generated magnesium carbenoid intermediates 67.
2-4. SN2-Type Reaction
Very interesting intramolecular SN2 reaction of magnesium carbenoid was observed.21 Thus, addition reaction of 1-chlorovinyl p-tolyl sulfoxide 65 with cyanomethyllithium afforded adduct 69. Treatment of 69 with excess i-PrMgCl resulted in the formation of spiro-type cyanocyclopropane 70 in high yield. Quite interestingly, quenching of this reaction with CH3OD afforded deuterated 70 with 99% deuterium incorporation. This result showed that this reaction is not the 1,3-CH insertion but SN2 reaction (Scheme 10). The mechanism of this reaction is as follows. Treatment of 69 with i-PrMgCl results in the formation of magnesium carbenoid 71 by the sulfoxide-magnesium exchange reaction. The acidic hydrogen on the carbon bearing a cyano group was eliminated with the excess i-PrMgCl to produce cyano-stabilized carbanion 72. Intramolecular SN2 reaction of the magnesium carbenoid with the cyano-stabilized carbanion occurs to give cyclopropylmagnesium chloride intermediate bearing a cyano group 73. Quenching of 73 with CH3OD gives a cyanocyclopropane bearing deuterium at 2-position 70 (D) with high deuterium incorporation. Intermediate 73 was found to be able to be trapped with several electrophiles other than proton. The whole procedure becomes a new method for the synthesis of multi-substituted cyanocyclopropanes. Thus, after treatment of 69 with 5 equivalents of i-PrMgCl in THF at 0 °C, Cu(I) iodide (20 mol%) followed by benzoyl chloride (8 equiv) were added to the reaction mixture and the whole was stirred at 0 °C for 1 h. This procedure gave the desired benzoylated products 74 in 65% yield.
3. CYCLOPROPYLMAGNESIUM CARBENOIDS
3-1. Alkylation of Cyclopropylmagnesium Carbenoids with a Grignard Reagnet
In 2001, the author’s group reported for the first time the generation of cyclopropylmagnesium carbenoids 76 from 1-chlorocyclopropyl phenyl sulfoxides 75 with i-PrMgCl by the sulfoxide-magnesium exchange reaction at low temperature.22 The generated magnesium carbenoids 76 were found to be stable at below -60 °C for at least 3 h. Quenching of this reaction with water or CH3OD gave chlorocyclopropanes 77 in high yields. When this reaction was conducted at 0 °C, allenes 78 were obtained in high yield via the Doering-Moore-Skattebol reaction (Scheme 11).
Later, cyclopropylmagnesium carbenoids were found to be highly electrophilic.23 Thus, 1-chlorocyclopropyl p-tolyl sulfoxide 79 was treated with excess i-PrMgCl in THF in the presence of HMPA gave alkylated cyclopropylmagnesium chloride 81 via cyclopropylmagnesium carbenoid 80 in good yield. The intermediate 81 was proved to be reactive with several electrophiles to give substituted cyclopropanes 82 in up to 84% yield. It is worth noting that above-mentioned alkylation is stereospecific reaction and two carbon-carbon bond-formations is carried out in one-pot to give multi-substituted cyclopropanes 82.23
3-2. Reaction with N-Lithio Arylamines
The reactions of cyclopropylmagnesium carbenoids with N-lithio arylamines gave quite interesting results (Scheme 12).24 Treatment of 1-chlorocyclopropyl phenyl sulfoxide 83 with i-PrMgCl resulted in the formation of cyclopropylmagnesium carbenoid 84. To this reaction mixture, N-lithio N-methyl p-anisidine was added to give N-cyclopropylated alkyl magnesium chloride 85. Quenching of this reaction with CH3OD gave deuterated N-cyclopropylated N-methyl p-anisidine 86 in 82% yield with almost perfect deuterium incorporation. Quenching of this reaction with aryl aldehydes gave adduct 87 in up to 53% yield.
Very interestingly, treatment of cyclopropylmagnesium carbenoid 89, generated from 1-chloro-2,2-dimethylcyclopropyl phenyl sulfoxide 88, with N-lithio N-methyl p-anisidine gave ortho-cyclopropylated N-methyl p-anisidine 90 in 60% yield without N-cyclopropylated compound.25 N-Lithio indoline 91 also reacted with 89 to give ortho-cyclopropylated product 93 without N-cyclopropylated compound via the reaction of 89 with resonance form 92. In general, 1-chlorocyclopropyl phenyl sulfoxides bearing at least two substituents on the cyclopropane ring gave ortho-cyclopropylated products.26
3-3. Reaction with O-Lithio Naphthols and Phenols
The reaction of cyclopropylmagnesium carbenoid 89 with O-lithio naphthols and phenols gave quite interesting one-carbon ring-expanded spiro[2.6]nonadienone derivatives 94 and 98 in variable yields (Scheme 13).27
Thus, treatment of cyclopropylmagnesium carbenoid 89 with 3 equiv of O-lithio 1-naphthols gave unexpected product, spiro[2.6]nonadienones 94 in up to 82% yield. A plausible mechanism of this reaction is as follows. Intermolecular SN2 reaction of carbenoid carbon with α-carbon of naphtholate would afford cyclopropylmagnsium chloride intermediate 95. Intramolecular alkylation of the carbanion to the carbonyl carbon would proceed to afford highly strained spiro[2.2]pentane intermediate 96. Carbon-carbon bond-cleavage of the cyclopropanolate moiety, depicted in 96, would take place easily because of the ring-strain to afford the final ring-expanded intermediate 97. Protonolysis of 97 will give the product 94. In order to confirm the final intermediate of this reaction to be 97, deuteriolysis of this reaction with MeOD was carried out and indeed deuterated product 94 (D) was obtained with perfect deuterium incorporation. The reaction of 84 with O-lithio phenol gave similar product 98 with somewhat lower yield. The mechanism of this reaction must be different compared with that for the reaction of O-lithio naphthols.27
A similar reaction was found to be able to carry out with the lithium enolate of β-tetralone and the reaction was proved to be highly stereospecific (Scheme 14).28 Thus, cyclopropylmagnesium carbenoids 101 and 102 were generated from sulfoxides 99 and 100, respectively, with i-PrMgCl. Reaction of 101 with excess lithium enolate of β-tetralone gave one-carbon ring-expanded spiro[2.6]nonanone derivative 103 in 81% yield as a sole product. The same reaction of 102 gave 104 in 82% yield as a sole product. The products 103 and 104 are diastereomers to each other. Namely, these reactions are highly stereospecific. The mechanism and origin of this stereospecificity are described in the original paper.28
3-4. Doering-LaFlamme-type Rearrangement
As shown in Scheme 11, cyclopropyl carbenoids rearranged to allenes (Doering-LaFlamme allene synthesis) at around 0 °C. This reaction was applied to a short synthesis of α-allenic alcohols from α,β-unsaturated carbonyl compounds (Scheme 15).29
Thus, treatment of α,β-unsaturated ester 105 with dichloromethyl p-tolyl sulfoxide in the presence of a base gave 1-chlorocyclopropyl p-tolyl sulfoxide 106 stereoselectively as a single product.30 The ester group was reduced to a hydroxymethyl group to give 107 in quantitative yield. Finally, alcohol 107 was treated with excess i-PrMgCl in toluene at 0 °C to give α-allenic alcohol 109 via cyclopropylmagnesium carbenoid 108 in 77% yield. A short synthesis of secondary α-allenic alcohol 111 was also realized. Thus, adduct 106 was reduced directly to aldehyde 110 with lithium diisobutyl-tert-butoxyaluminum hydride (LDBBA) in good yield. Treatment of 110 with excess Grignard reagent resulted in the formation of secondary α-allenic alcohol 111 with nucleophilic addition of the Grignard reagent to the aldehyde and, at the same time, the sulfoxide-magnesium exchange reaction. It is worth noting that 111 was synthesized from 105 in only three steps in moderate overall yield.
This procedure was extended to an asymmetric synthesis of α-allenic alcohols (Scheme 16).31 The reaction of α,β-unsaturated ester 112 with enantiopure (R)-dichloromethyl p-tolyl sulfoxide32 was carried out with LiHMDS to give cyclopropane 113 in high optical yield with moderate chemical yield. The ester group was reduced to a hydroxymethyl group to afford alcohol 114. After the optical purity was improved to 99% by recrystallization, 114 was treated with i-PrMgCl to give optically active (R)-116 via cyclopropylmagnesium carbenoid intermediate 115. Absolute configuration, optical purity, and the mechanism of the asymmetric induction are described in the original paper.31
3-5. 1,5-CH Insertion Reaction
Cyclopropylmagnesium carbenoid generated from 1-chlorocyclopropyl p-tolyl sulfoxides bearing an alkoxymethyl group at the 2-position was found to take place 1,5-CH insertion reaction to give 3-oxabicyclo[3.1.0]hexanes in good yield (Scheme 17).33 Treatment of ethyl methacrylate with dichloromethyl p-tolyl sulfoxide in the presence of NaHMDS gave cyclopropane 117 in quantitative yield. Reduction followed by alkylation of 117 gave 1-chlorocyclopropyl p-tolyl sulfoxides bearing an alkoxymethyl group at the 2-position 119 via alcohol 118. Sulfoxide 119 was treated with i-PrMgCl to result in the generation of cyclopropylmagnesium carbenoid 120. 1,5-CH insertion reaction of 120 proceeded to afford 3-oxabicyclo[3.1.0]hexane 121 in 82% yield along with protonated product 122. This procedure offers a short synthesis of 3-oxabicyclo[3.1.0]hexanes from α,β-unsaturated carbonyl compounds with dichloromethyl p-tolyl sulfoxide in good overall yield.
This procedure was applied to the asymmetric synthesis of 3-oxabicyclo[3.1.0]hexanes. As an example, asymmetric synthesis of 2-oxatricyclodecane 127 is described (Scheme 18).34 Thus, treatment of α,β-unsaturated ketone 123 with (R)-dichloromethyl p-tolyl sulfoxid in the presence of LDA afforded enantiopure spiro-cyclopropane 124 in quantitative yield with perfect asymmetric induction from the sulfur chiral center. The ketone group was reduced and the resulting hydroxyl group was alkylated to give ether 126 in high overall yield via alcohol 125. Absolute configuration of 125 was determined from X-ray analysis. Finally, 126 was treated with i-PrMgCl to give enantiopure (1S,3R,4S,5S,6R)-3-phenyl-5-(2-phenylethyl)-2-oxatricyclo[4.4.0.04,6]decane 127 in 51% yield through the 1,5-CH insertion reaction of the generated cyclopropylmagnesium carbenoid intermediate.34
4. CYCLOBUTYLMAGNESIUM CARBENOID
4-1. Alkylation of Cyclobutylmagnesium Carbenoids with a Grignard Reagent
Similar to the cyclopropylmagnesium carbenoids described in chapter 3, cyclobutylmagnesium carbenoids, for example 129 in Scheme 19, can be generated from 1-chlorocyclobutyl p-tolyl sulfoxides with a variety of Grignard reagents at low temperature.35 Cyclobutylmagnesium carbenoid 129 was proved to be stable at -78 °C for at least 30 min. When this reaction was conducted with excess Grignard reagent, for example EtMgCl, ethylated cyclobutylmagnesium chloride 130 was obtained. This result indicates that cyclobutylmagnesium carbenoids are quite electrophilic. Quenching of this intermediate 130 with several electrophiles, multi-substituted cyclobutanes 131 were obtained in moderate to good yield. It is noteworthy that multi-substituted cyclobutanes 131 were obtained from 128 in one-pot reaction with two carbon-carbon bond-formation.
A novel method for the synthesis of alkylidenecyclobutanes was realized by the reaction of cyclobutylmangesium carbenoids with lithium α-sulfonyl carbanions (Scheme 20).36 For example, Treatment of 1-chlorocyclobutyl p-tolyl sulfoxide 128 with 2.5 equiv of EtMgCl in THF at -78 °C resulted in the formation of cyclobutylmagnesium carbenoid 129. To this was added 3 equiv of lithium α-sulfonyl carbanion and the temperature of the reaction mixture was slowly allowed to warm to -20 °C to give alkylidenecyclobutanes 132 in up to 83% yield.36 When this reaction was carried out with three equiv of lithium α-sulfonyl carbanions generated from vinyl sulfones (for example, the vinyl sulfones shown in Scheme 20) with n-BuLi, allylidenecyclobutanes 133 or 134 were obtained in good yields. Actual reactive species of this reaction was proved to be lithium α-sulfonyl carbanion of allyl sulfones.37
4-3. 1,2-CC Insertion Reaction Giving Alkylidenecyclopropanes
Very interesting 1,2-CC insertion reaction of cyclobutylmagnesium carbenoid giving alkylidenecyclopropanes was found and this reaction was applied to the synthesis of enatniopure alkylidenecyclopropanes (Scheme 21).38 Thus, optically active 1-chlorovinyl p-tolyl sulfoxide 44 was treated with lithium enolate of tert-butyl 3-phenylpropionate to afford adduct 135 in quantitative yield.
Stereochemistry of the adduct 135 (syn-relationship between the chlorine atom and the benzyl group) was determined based on our previous study. The adduct was treated with trifluoroacetic acid in dichloromethane to give a carboxylic acid, which was reduced with BH3-THF in THF at 0 °C to give alcohol 136 in 91% overall yield from 135. The hydroxyl group in 136 was converted to iodide group under the conventional reaction and the resultant iodide was treated with two equivalents of KHMDS to give the desired 1-chlorocyclobutyl p-tolyl sulfoxide 137 in 94% overall yield from 136 as a single product. The key reaction was carried out with cyclopentylmagnesium chloride at -40 °C to give optically active alkylidenecyclopropane 139 in 87% yield with 97% ee via the 1,2-CC insertion reaction of cyclobutylmagnesium carbenoid intermediate 138.38
5. MAGNESIUM ALKYLIDENE CARBENOIDS
5-1. Reaction of Magnesium Alkylidene Carbenoids with Nitrogen-containing Heterocycles
Previously, the author’s group reported, for the first time, the generation of magnesium alkylidene carbenoids 140 from 1-chlorovinyl p-tolyl sulfoxide 65 with a Grignard reagent. The magnesium alkylidene carbenoids were found to be quite electrophilic (Scheme 22).39 For example, treatment of 65 with excess phenylmagnesium bromide at low temperature resulted in the formation of magnesium alkylidene carbenoid 140, which reacted with the used phenylmagnesium bromide electrophilically to afford phenylated alkenylmagnesium bromide intermediate 141. The intermediate 141 was proved to be reactive with a variety of electrophiles to afford tetra-substituted olefin 142 in moderate to good yields with continuous two carbon-carbon bond-formation in one-pot. Later, magnesium alkyliene carbenoid 140 was found to be stable at -78 °C for at least 30 min.40
As described above, magnesium alkylidene carbenoids were proved to be quite electrophilic. We found that the reaction of carbenoid 140 with N-lithio nitrogen-containing heterocycles gave N-alkenylated nitrogen-containing heterocycles 143 (Scheme 23).41 For example, treatment of 65 in toluene with i-PrMgCl followed by N-lithio indole afforded N-alkenylindole 143 in 57% yield. The reaction with N-lithio phenothiazine and phenoxazine gave better yields of 70%. This reaction provides unique way for the direct N-alkenylation of nitrogen-containing heterocycles.
The intermediate of this reaction was proved to be alkenylmagnesium compounds, which could be trapped with several electrophiles to give nitrogen-containing heterocycles bearing a fully substituted olefin on the nitrogen (Scheme 24).41
Thus, reaction of 65 with i-PrMgCl gave magnesium alkylidene carbenoid 140. To this reaction mixture was added three equiv of N-lithio phenothiazine and temperature of the reaction mixture was slowly allowed to warm to -10 °C to give alkenylmagnesium intermediate 144. Finally, excess electrophile was added to the reaction mixture to give phenothiazine bearing a fully substituted olefin 145 in moderate yield in one-pot reaction.41
5-2. Reaction of Magnesium Alkylidene Carbenoids with Thiophenes and Furans
Magnesium alkylidene carbenoids were found to be reactive with 2-lithiothiophenes in good yields (Scheme 25).42 Thus, treatment of magnesium alkylidene carbenoid 140, derived from 65 with i-PrMgCl in a mixture of toluene-THF, with three equiv of 2-lithiothiophene afforded 2-alkenylthiophene 147 (R=H) in 78% yield via alkenylmagnesium chloride intermediate 146. As shown in Scheme 25, various kinds of 2-lithiothiophenes reacted with 140 to give 2-alkenylthiophenes 147 in good yields.
The alkenylmagnesium chloride intermediates 146 were proved to be reactive with several electrophiles to give thiophenes bearing a fully substituted olefin at the 2-position in good yields. Representative examples are shown in Scheme 26.42 Thus, the reaction of magnesium alkylidene carbenoids 140 with 2-lithiothiophene gave alkenylmagnesium chloride intermediate 148. To this reaction mixture was added excess amount of electrophiles to give thiophenes bearing a fully substituted olefin 149 in up to 72% yield. This reaction provides a unique way for the synthesis of thiophenes having a fully substituted olefin at the 2-position in relatively short steps.
A similar reaction can be conducted with 2-lithiofurans (Scheme 27).43 Thus, 1-chlorovinyl p-tolyl sulfoxide 65 was treated with t-BuMgCl followed by i-PrMgCl in THF at -78 °C to give magnesium alkylidene carbenoid 140. A solution of 2-lithiofuran (generated from furan with n-BuLi in THF; 3 equivalents) was added to the solution of 140 and the temperature of the reaction mixture was slowly allowed to warm to -10 °C. This reaction gave the desired 2-alkenylfuran 150 (R=H) in 82% yield. The results for the reaction of 140 with various kinds of 2-lithiofurans to give 2-alkenylfurans are summarized in Scheme 27.
The intermediate of this reaction was again found to be the alkenylmagnesium 151 (Schene 28). Thus, quenching the reaction of 140 with 2-lithiofuran with MeOD gave deuterated alkenylfuran 152 (E=D) in 78% yield with 96% deuterium content. In the same way described above, the intermediate 151 could be trapped with various electrophiles to afford furans bearing a fully substituted alkene at the 2-position 152 in good yields.
Very interesting reaction was found from the reaction of magnesium alkylidene carbenoid 140 with 2-lithio-5-methoxyfuran (Scheme 29).43 Reaction of 140 with 2-lithio-5-methoxyfuran under the same conditions as described above gave an unexpected product 156a in 67% yield. From the spectral data, an allene conjugated with α,β-unsaturated methyl ester was determined to be the structure of the product 156a. A plausible mechanism for the reaction is shown in Scheme 29. Thus, 2-lithio-5-methoxyfuran attacks the carbenoid carbon to give alkenylmagnesium intermediate 153. The anion would cleave the C-O bond of the furan ring to afford 1,2,3-triene conjugated with enolate 154. This triene intermediate must be unstable and transfer of the charge on the oxygen to the allenic carbon gives intermediate 155, which is protonated to give product 156a. When this reaction was quenched with MeOD, deuterated allene 156b was obtained with perfect deuterium incorporation.
5-3. Reaction of Magnesium Alkylidene Carbenoids with Lithium Ester Enolates
Magnesium alkylidene carbenoids were found to be reactive with lithium ester enolates to afford β,γ-unsaturated esters in moderate yields (Scheme 30).44 Thus, the treatment of magnesium alkylidene carbenoids 158 derived from 1-chlorovinyl p-tolyl sulfoxides 157 with lithium enolate of tert-butyl acetate gave β,γ-unsaturated esters 159 in up to 63% yield. Although the yields are not always good at the present, this method is an unprecedented way and contributes to a synthesis of various β,γ-unsaturated esters. When lithium ester enolate of tert-butyl chloroacetates were used in this reaction, allenic esters 161 were obtained in up to 54% yield. Thus, treatment of magnesium alkylidene carbenoid 140 with lithium enolate of tert-butyl chloroacetate under the conditions shown in Scheme 30 gave allenic ester 161a in 54% yield. This reaction is presumed to proceed as follows. The lithium ester enolate attacks the carbenoid carbon of 140 to give alkenylmagnesium intermediate 160 with inversion of the configuration of the carbenoid carbon. β-Elimination of both the magnesium chloride and the chlorine atom in intermediate 160 took place simultaneously to afford allenic ester 161.
6. MAGNESIUM β-OXIDO CARBENOIDS
β-Oxido carbenoid rearrangement is one of the most useful reactions for the homologation and/or elongation of carbonyl compounds. Several methods for the homologation of carbonyl compounds by using the β-oxido carbenoid rearrangement as the key reaction have been reported.45 We recently reported an interesting one-carbon homologation of ketones by using the magnesium β-oxido carbenoid rearrangement as the key reaction (Scheme 31).46 Thus, the reaction of the lithium α-sulfinyl carbanion of 1-chloroethyl p-tolyl sulfoxide with cyclohexanone derivative 162 gave two adducts 163 (60%) and 164 (35%) as easily separable two diastereomers. The stereochemistry of each adduct was determined by X-ray crystallographic analysis. Main adduct 163 was treated with t-BuMgCl (1.2 equiv) followed by i-PrMgCl (4 equiv) in THF at 0 °C. The sulfoxide-magnesium exchange reaction took place smoothly to give ring-expanded product 166 in 83% yield with trace amount of isomer 167. Significant difference in the reactivity of 163 and 164 with i-PrMgCl was observed. Thus, when the minor adduct 164 was treated with t-BuMgCl (1.2 equiv) followed by i-PrMgCl (4 equiv) in THF, the reaction was rather slow and equiv) and prolonging the reaction time gave
completed reaction and the same cycloheptanone 166 was obtained in 64% yield. Namely, these two reactions are highly selective. The rational explanation of this selectivity and the difference of the reactivity are described in the original paper.47 The intermediate of this β-oxido carbenoid rearrangement was proved to be magnesium enolate 165, which could be trapped with electrophiles to give 2,2,7-trisubstituted cycloheptanones 168 in good yields from 163 in one-pot.47
In continuation of the study for one-carbon homologation of carbonyl compounds using β-oxido carbenoid rearrangement as the key reaction, we recently investigated the synthesis of aryl(chloro)methyl p-tolyl sulfoxides and uses them in the synthesis of α-aryl ketones (Scheme 32).48 A representative example is shown in Scheme 32. Thus, the addition reaction of lithium α-sulfinyl carbanion derived from chloro(phenyl)methyl p-tolyl sulfoxide with cyclohexanone derivative gave adduct 169 in quantitative yield. Sequential treatment of 169 with tert-BuMgCl and i-PrMgCl resulted in the one-carbon ring-expanded magnesium enolate intermediate via the magnesium β-oxido carbenoid. Finally, trapping of this intermediate with various electrophiles afforded 9-substituted-9-phenyl-2,4- dioxaspiro[4.6]undecane-8-one 170 in up to 85% yield from adduct 169. Although there are some limitations in this procedure, our results contribute to the synthesis of α-aryl ketones from aldehydes and ketones by one-carbon homologation and/or elongation.
7. CONCLUSION
As magnesium carbenoids, outlined in this review, are relatively stable compared with the corresponding traditional lithium carbenoids, we can manage the magnesium carbenoids in a similar way as the usual reactants with a little precaution. Concerning the generation of the magnesium carbenoids, starting from sulfoxides bearing chlorine atom on the α-position with Grignard reagents via the sulfoxide-magnesium exchange reaction gives much higher versatility compared with the halogen-magnesium exchange reaction. The magnesium carbenoids show both nucleophilic and electrophilic properties; however, the electrophilic reaction with nucleophiles is far more interesting from the synthetic viewpoint. Many new and very interesting results will be forthcoming from this field.
References
1. Some monographs and reviews concerning chemistry of carbenes and carbenoids: W. Kirmse, Carbene Chemistry; Academic: New York, 1971; F. Z. Dorwald, Metal Carbenes in Organic Synthesis; Wiley-VCH: Weinheim, 1999; Carbene Chemistry; ed. by G. Bertrand, Marcel Dekker: New York, 2002; G. Kobrich, Angew. Chem., Int. Ed. Engl., 1972, 11, 473; CrossRef P. J. Stang, Chem. Rev., 1978, 78, 383; CrossRef S. D. Burke and P. A. Grieco, Org. React., 1979, 26, 361; H. F. Schaefer, III, Acc. Chem. Res., 1979, 12, 288; CrossRef H. Wynberg and E. W. Meijer, Org. React., 1982, 29, 1; K. G. Taylor, Tetrahedron, 1982, 38, 2751; CrossRef A. Oku and T. Harada, J. Syn. Org. Chem. Jpn., 1986, 44, 736; CrossRef A. Oku, J. Syn. Org. Chem. Jpn., 1990, 48, 710; CrossRef J. Adams and D. M. Spero, Tetrahedron, 1991, 47, 1765; CrossRef A. Padwa and M. D. Weingarten, Chem. Rev., 1996, 96, 223; CrossRef F. Zaragoza, Tetrahedron, 1997, 53, 3425; CrossRef W. Kirmse, Angew. Chem., Int. Ed. Engl., 1997, 36, 1164; CrossRef M. Braun, Angew. Chem. Int. Ed., 1998, 37, 430; CrossRef G. Boche and J. C. Lohrenz, Chem. Rev., 2001, 101, 697; CrossRef G. Mehta and S. Muthusamy, Tetrahedron, 2002, 58, 9477; CrossRef P. Muller, Acc. Chem. Res., 2004, 37, 243; CrossRef R. Knorr, Chem. Rev., 2004, 104, 3795; CrossRef Z. Zhang and J. Wang, Tetrahedron, 2008, 64, 6577. CrossRef
2. Some reviews concerning CH insertion reaction: A. Padwa and K. E. Krumpe, Tetrahedron, 1992, 48, 5385; CrossRef G. A. Sulikowski, K. L. Cha, and M. M. Sulikowski, Tetrahedron: Asymmetry, 1998, 9, 3145; CrossRef H. M. L. Davies and R. E. J. Beckwith, Chem. Rev., 2003, 103, 2861; CrossRef H. M. L. Davies and O. Loe, Synthesis, 2004, 2595; CrossRef H. M. L. Davies, Angew. Chem. Int. Ed., 2006, 45, 6422; CrossRef Z. Zhang and J. Wang, Tetrahedron, 2008, 64, 6577. CrossRef
3. T. Satoh, Chem. Soc. Rev., 2007, 36, 1561; CrossRef T. Satoh, In The Chemistry of Organomagnesium Compounds; ed. by Z. Rappoport and I. Marek, John Wiley and Sons: Chichester, 2008, pp. 717-769; T. Satoh, J. Syn. Org. Chem. Jpn., 2009, 67, 381; CrossRef T. Satoh, YAKUGAKU ZASSHI, 2009, 129, 1013. CrossRef
4. Some reviews concerning carbon-hydrogen insertion reaction: A. Padwa and K. E. Krumpe, Tetrahedron, 1992, 48, 5385; CrossRef G. A. Sulikowski, K. L. Cha, and M. M. Sulikowski, Tetrahedron: Asymmetry, 1998, 9, 3145; CrossRef H. M. L. Davies and R. E. J. Beckwith, Chem. Rev., 2003, 103, 2861; CrossRef H. M. L. Davies and O. Loe, Synthesis, 2004, 2595; CrossRef H. M. L. Davies, Angew. Chem. Int. Ed., 2006, 45, 6422; CrossRef Z. Zhang and J. Wang, Tetrahedron, 2008, 64, 6577. CrossRef
5. T. Satoh, A. Kondo, and J. Musashi, Tetrahedron, 2004, 60, 5453. CrossRef
6. T. Satoh, A. Osawa, and A. Kondo, Tetrahedron Lett., 2004, 45, 6703; CrossRef T. Satoh, A. Osawa, T. Ohbayashi, and A. Kondo, Tetrahedron, 2006, 62, 7892. CrossRef
7. T. Ohbayashi, S. Mitsunaga, and T. Satoh, Tetrahedron Lett., 2007, 48, 7829. CrossRef
8. S. Mitsunaga, T. Ohbayashi, S. Sugiyama, T. Saitou, M. Tadokoro, and T. Satoh, Tetrahedron: Asymmetry, 2009, 20, 1697. CrossRef
9. T. Satoh, J. Musashi, and A. Kondo, Tetrahedron Lett., 2005, 46, 599. CrossRef
10. T. Satoh, S. Ogata, and D. Wakasugi, Tetrahedron Lett., 2006, 47, 7249. CrossRef
11. S. Ogata, H. Saitoh, D. Wakasugi, and T. Satoh, Tetrahedron, 2008, 64, 5711. CrossRef
12. S. Ogata, S. Masaoka, K. Sakai, and T. Satoh, Tetrahedron Lett., 2007, 48, 5017. CrossRef
13. H. Watanabe, S. Ogata, and T. Satoh, Tetrahedron, 2010, 66, 5675. CrossRef
14. T. Satoh, T. Kuramoto, S. Ogata, H. Watanabe, T. Saitou, M. Tadokoro, and T. Satoh, Tetrahedron: Asymmetry, 2010, 21, 1. CrossRef
15. T. Satoh, H. Yamashita, and J. Musashi, Tetrahedron Lett., 2007, 48, 7295. CrossRef
16. H. Yamashita and T. Satoh, Tetrahedron, 2009, 65, 613. CrossRef
17. T. Satoh, Y. Awata, S. Ogata, S. Sugiyama, M. Tanaka, and M. Tori, Tetrahedron Lett., 2009, 50, 1961. CrossRef
18. T. Satoh, Y. Awata, Y. Kato, S. Ogata, M. Ishigaki, S. Sugiyama, and H. Saitoh, Tetrahedron, 2011, 67, 1102. CrossRef
19. H. Saitoh, N. Ishida, and T. Satoh, Tetrahedron Lett., 2010, 51, 633. CrossRef
20. N. Ishida, H. Saitoh, S. Sugiyama, and T. Satoh, Tetrahedron, 2011, 67, 3081. CrossRef
21. H. Saitoh and T. Satoh, Tetrahedron Lett., 2010, 51, 3380. CrossRef
22. T. Satoh, T. Kurihara, and K. Fujita, Tetrahedron, 2001, 57, 5369. CrossRef
23. M. Yajima, R. Nonaka, H. Yamashita, and T. Satoh, Tetrahedron Lett., 2009, 50, 4754. CrossRef
24. T. Satoh, M. Miura, K. Sakai, and Y. Yokoyama, Tetrahedron, 2006, 62, 4253. CrossRef
25. Y. Yamada, M. Miura, and T. Satoh, Tetrahedron Lett., 2008, 49, 169. CrossRef
26. Y. Yamada, M. Mizuno, S. Nagamoto, and T. Satoh, Tetrahedron, 2009, 65, 10025. CrossRef
27. T. Satoh, S. Nagamoto, M. Yajima, Y. Yamada, Y. Ohata, and M. Tadokoro, Tetrahedron Lett., 2008, 49, 5431. CrossRef
28. T. Satoh, G. Kashiwamura, S. Nagamoto, Y. Sasaki, and S. Sugiyama, Tetrahedron Lett., 2011, 52, 4468. CrossRef
29. T. Satoh, T. Noguchi, and T. Miyagawa, Tetrahedron Lett., 2008, 49, 5689. CrossRef
30. T. Miyagawa, T. Tatenuma, M. Tadokoro, and T. Satoh, Tetrahedron, 2008, 64, 5279. CrossRef
31. H. Momochi, T. Noguchi, T. Miyagawa, N. Ogawa, M. Tadokoro, and T. Satoh, Tetrahedron Lett., 2011, 52, 3016. CrossRef
32. T. Noguchi, T. Miyagawa, and T. Satoh, Tetrahedron: Asymmetry, 2009, 20, 2073. CrossRef
33. T. Satoh, S. Ikeda, T. Miyagawa, and T. Noguchi, Tetrahedron Lett., 2010, 51, 1955. CrossRef
34. T. Satoh, T. Tsuru, S. Ikeda, T. Miyagawa, H. Momochi, and T. Kimura, Tetrahedron, submitted.
35. T. Satoh, T. Kasuya, T. Miyagawa, and N. Nakaya, Synlett, 2010, 286. CrossRef
36. T. Satoh, T. Kasuya, M. Ishigaki, M. Inumaru, T. Miyagawa, N. Nakaya, and S. Sugiyama, Synthesis, 2011, 397. CrossRef
37. M. Ishigaki, M. Inumaru, and T. Satoh, Tetrahedron Lett., 2011, 52, 5563. CrossRef
38. N. Nakaya, S. Sugiyama, and T. Satoh, Tetrahedron Lett., 2009, 50, 4212. CrossRef
39. T. Satoh, K. Takano, H. Ota, H. Someya, K. Matsuda, and M. Koyama, Tetrahedron, 1998, 54, 5557. CrossRef
40. T. Satoh, T. Sakamoto, M. Watanabe, and K. Takano, Chem. Pharm. Bull., 2003, 51, 966. CrossRef
41. J. Sakurada and T. Satoh, Tetrahedron, 2007, 63, 3806. CrossRef
42. T. Satoh, N. Mori, and K. Obuchi, Tetrahedron Lett., 2007, 48, 6453. CrossRef
43. N. Mori, K. Obuchi, T. Katae, J. Sakurada, and T. Satoh, Tetrahedron, 2009, 65, 3509. CrossRef
44. T. Satoh, H. Kaneta, A. Matsushima, and M. Yajima, Tetrahedron Lett., 2009, 50, 6280. CrossRef
45. T. Satoh, J. Syn. Org. Chem. Jpn., 2009, 67, 381. CrossRef
46. T. Satoh, S. Tanaka, and N. Asakawa, Tetrahedron Lett., 2006, 47, 6769. CrossRef
47. S. Tanaka, T. Anai, M. Tadokoro, and T. Satoh, Tetrahedron, 2008, 64, 7199. CrossRef
48. S. Fukuda, K. Tsuji, J. Musashi, R. Nonaka, T. Kimura, and T. Satoh, Synthesis, 2011, 3615. CrossRef