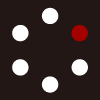
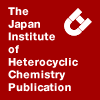
HETEROCYCLES
An International Journal for Reviews and Communications in Heterocyclic ChemistryWeb Edition ISSN: 1881-0942
Published online by The Japan Institute of Heterocyclic Chemistry
e-Journal
Full Text HTML
Received, 16th October, 2011, Accepted, 21st November, 2011, Published online, 28th November, 2011.
DOI: 10.3987/COM-11-12373
■ First Synthesis of 2-Heteroarylazulenes by the Electrophilic Substitution of Azulene with Triflate of N-Containing Heterocycles
Taku Shoji,* Yuta Inoue, Shunji Ito, Tetsuo Okujima, and Noboru Morita
Department of Chemistry, Faculty of Science, Shinshu University, Asahi, Matsumoto 390-8621, Japan
Abstract
An efficient synthesis of 2-heteroarylazulene derivatives was established via electrophilic substitution. The reaction of 6-dimethylamino-1,3-di(methylthio)azulene (1a) with the triflate of N-containing heteroarenes proceeded in the presence of excess heteroarenes to afford the corresponding 2-dihydroheteroarylazulene derivatives 3−7. The 2-dihydroheteroarylazulene derivatives were readily converted into the desired 2-heteroarylazulene derivatives 8−11 by the treatment with KOH in alcohols in excellent yields, except for 4 and 5.Electrophilic substitution reactions are a very important and general methodology for the functionalization of aromatic compounds. In azulene derivatives, there are numerous reports for the electrophilic substitution reactions at the 1- and 3-positions of the azulene ring.1 However, there are few reports on the functionalization of azulene derivatives at the 2-position utilizing electrophilic substitution reactions, because 2-position of azulene is inert site toward the electrophile. In 1962, Hafner and co-workers reported that 1,3-dialkyl-substituted azulene derivatives undergo the Vilsmeier formylation reaction using DMF and POCl3 to give corresponding 2-formylazulenes, but only with very low selectivity compared to ipso-substitution at the 1-position and electrophilic substitution at the 5-position.2 Thus, functionalization for 2-position of azulene by electrophilic reaction is remained as a subject of azulene chemistry.
In recent years, there has been intense interest in transition-metal-catalyzed carbon-carbon and carbon-heteroatom bond formation in aromatic compounds.3 In the chemistry of azulene, functionalization method for 2-position of azulene is mainly transition-metal-catalyzed because difficulty of electrophilic reaction. Thus, several applications of transition-mediated reactions, e.g., vinylation,4 ethynylation,5 and amination6 utilizing azulenyl halides have appeared in the literature. We have also reported the palladium-catalyzed synthesis of various arylazulene derivatives.7 However, as another problem, synthesis of 2-arylazulenes by the transition-metal-catalyzed cross-coupling reaction required synthetically difficult 2-haloazulene and/or instable 2-azulenyl metal reagent.8
More recently, we have demonstrated that the reaction of azulene derivatives with the triflate of N-containing heteroarenes, which are readily available from the reaction of N-containing heteroarenes with trifluoromethanesulfonic anhydride (Tf2O), gives the corresponding dihydroheteroarylazulene derivatives. Transformation of the dihydroheteroarylazulene derivatives to the corresponding heteroarylazulene derivatives opened a new, two-step strategy for the heteroarylation of azulene at the 1-, 3-, 5-, and 7-positions.9 If the synthetic strategy by electrophilic heteroarylation can be applicable to 2-position, a new and efficient synthetic route to the 2-heteroarylazulene derivatives should be established. For the success of this reaction, introduction of protecting groups at the highly reactive 1- and 3-positions and powerful electron-donation group at the 6-position of the azulene ring would be essential to proceed the electrophilic substitution reactions at the 2-position.
We report herein the reaction of 6-dimethylamino-1,3-di(methylthio)azulene (1a), which is an azulene derivative possessing the required protecting groups at these highly reactive 1- and 3-positions and powerful electron-donating dimethylamino group at the 6-position, with the triflates of the N-containing heteroarenes, and their transformation to the corresponding 2-heteroarylazulenes through an electrophilic substitution reaction.
Introduction of protecting groups at the highly reactive 1- and 3-positions of the azulene ring should be crucial to electrophilic substitution reactions at the 2-position of azulene. Thus, for the functionalization at the 2-position of the azulene, 6-dimethylamino-1,3-di(methylthio)azulene (1a), which is prepared by electrophilic sulfinylation of 6-dimethylaminoazulene10 with (CF3CO)2O/DMSO, following to the treatment with Et3N, was applied for the electrophilic substitution with the triflates of several N-containing heteroarenes. The methylthio substituents at the 1- and 3-positions would suppress the most reactive site for the azulene ring and also the substituents might be subjected to further functionalization by electrophilic ipso-substitution reaction reported by us.11 Desired compound 1a was obtained in 97% yield as the sole product when the reaction was carried out with 3.0 equiv. of (CF3CO)2O and excess DMSO toward the 6-dimethylaminoazulene (Scheme 1), although the generation of 1,3-di(methylthio)azulene derivatives was not observed in the case of the parent and 6-tert-butylazulene in same reaction condition.11 Thus, the dimethylamino group on the 6-position of azulene ring should enhance the reactivity of the azulene toward the electrophile.
As a preliminary experiment for the synthesis of 2-heteroarylazulenes, the Tf2O activated electrophilic substitution of 6-dimethylamino-1,3-di(methylthio)azulene (1a) with pyridine was examined in four different organic solvents (Scheme 1, Table 1).
As summarized in Table 1, yields of the product were significantly depended on the solvent employed. Despite the fact that the highly polar solvent acetonitrile was successful solvent in the Tf2O activated Reissert-Henze type electrophilic arylation of azulenes,12 the yield of 2 was quite low (8%) because the significant decomposition of the compound was observed in this reaction condition. The less polar solvents compared with acetonitrile, i.e., in chloroform and 1,2-dichloroethane, were also revealed to be insufficient for the reaction due to the low yield of the product, likewise a case of acetonitrile. Among the solvents tested, dichloromethane was found to be the best with respect to the yield of the product (33% yield). Presumed reaction mechanism of this reaction is also shown in Scheme 1. Namely, reaction of trifluoromethanesulfonic anhydride with pyridine forms 1-trifluoromethanesulfonylpyridinium trifluoromethanesulfonate (TPT). Generated TPT is attacked by 2-position of 1a to give dihydropyridine derivative 2.
A series of 6-substituted azulene derivatives were subjected to this electrophilic substitution under the optimized reaction conditions to explore the scope of this reaction. Examined azulene derivatives are shown in Chart 1.
The reaction of 1,3-di(methylthio)azulene (1b),11 6-tert-butyl-1,3-di(methylthio)azulene (1c)11 and 6-methoxy-1,3-di(methylthio)azulene (1d) with pyridine did not proceed and starting material was recovered quantitatively.13 These results indic ate that this electrophilic substitution required a highly electron-donating substituent on the 6-position.
To examine the generality of the reaction with the heteroarenes, we investigated the reaction of 1a with commercially available five heteroarenes under the optimized reaction conditions (Scheme 2, Table 2).14 Quinoline was reacted with 1a in the presence of Tf2O to give the 2-(dihydroquinoline-4-yl)azulene 3 in 49% yield, along with the generation of 2-(dihydroquinoline-2-yl)azulene 4 in 16% yield. The regio-isomers of 3 and 4 were readily isolated by reversed-phase chromatography (ODS gel with 85% methanol as eluent). The reaction of 1a with isoquinoline in the presence of Tf2O gave the presumed 2-(isoquinolin-1-yl)azulene 5 in 56% yield. Acridine and 1,10-phenanthroline were also reacted with 1a in the presence of Tf2O to give the corresponding dihydroheterocycles 6 and 7 in 11% and 19% yields, respectively, but the yields were relatively low. The low yield of 6 and 7 should be attributable to the instability of these compounds under the ambient condition.
For the purpose of transformation to the 2-(heteroaryl)azulenes, we investigated base induced aromatization of compounds 3−7 (Scheme 3).15 The yield of the products was summarized in Table 3. The reaction of 2 with KOH/methanol solution at reflux temperature afforded the corresponding 2-(4-pyridyl)azulene derivative (8) in 100% yield. Although the reaction of 3 with KOH refluxing in methanol was not proceeded, aromatization of 3 was established by treatment with KOH in isopropyl alcohol, which has higher boiling point than methanol, to afford 9 in 98% yield. For the aromatization of 4 and 5, we investigated the use of two bases, KOH and tert-BuOK in DMSO, following a similar procedure to that reported by us previously. However, base induced aromatization procedures of 4 and 5 led to decomposition of compound. Therefore, suitable aromatization condition of 4 and 5 to the corresponding heteroarene derivatives is currently investigated. When 6 and 7 were treated with KOH in methanol, aromatization of both 6 and 7 was also achieved to afford 10 and 11 in 91% and 100% yields, respectively.
Usually, transition-metal-catalyzed aryl-aryl cross-coupling is required both aryl halides and organometallic reagents for the construction of the biaryl compounds. In the azulene chemistry, although many transition-metal-catalyzed aryl-aryl cross-coupling reactions have been reported, the synthesis of 2-arylazulenes by such methods should be difficult because preparation of 2-haloazulenes required relatively long synthetic pathway. The advantageous feature of this synthetic methodology is that these reactions do not require any transition-metal catalyst, 2-haloazulene, and a coupling reagent. Moreover, this method can be selective synthesis of 2-heteroarylazulenes.
In conclusion, for the first time, an efficient synthesis of 2-heteroarylazulene derivatives through electrophilic substitution without using a transition-metal catalyst has been established. The intermediates, 2-(dihydroheteroaryl)azulene derivatives, were also obtained by reaction with N-containing heteroarenes in the presence of Tf2O under milder reaction conditions. Treatment of the 2-(dihydroheteroaryl)azulene derivatives with KOH gave the desired 2-heteroarylazulene derivatives, quantitatively, except for 4 and 5. Previously, we reported the 2-arylazulene derivatives exhibited significant electrochromic properties under electrochemical reaction basis on the multi-stage electron transfer.8c,16 Moreover, We also reported the 1,3-di(methylthio)azulenes exhibit reversible redox behavior on cyclic voltammetry, arising from the generation of stable radical anionic and cationic species.11 Thus, 1,3-di(methylthio)azulenes bearing a 2-heteroaryl groups might exhibit further stability in their electrochemical reaction. To evaluate the scope of this class of molecules investigated by this research, detail electrochemical and optical properties of reported 2-heteroarylazulene derivatives are currently investigating in our laboratory.
ACKNOWLEDGEMENTS
This work was supported by a Grant-in-Aid for Research Activity Start-up (Grant 22850007 to T.S.) from the Ministry of Education, Culture, Sports, Science, and Technology, Japan.
References
1. K.-P. Zeller, “Azulene”, in Houben-Weyl, Methoden der Organischen Chemie, 4th ed. (Ed.: H. Kropf), Georg Thieme, Stuttgart, Germany, 1985, vol. V, part 2c, pp. 127–418.
2. K. Hafner and K. L. Moritz, Justus Liebigs Ann. Chem., 1962, 656, 40. CrossRef
3. (a) J.-P. Corbet and G. Mignani, Chem. Rev., 2006, 106, 2651; CrossRef (b) D. Alberico, M. E. Scott, and M. Lautens, Chem. Rev., 2007, 107, 174; CrossRef (c) C.-L. Sun, B.-J. Li, and Z.-J. Shi, Chem. Rev., 2011, 111, 1293. CrossRef
4. H. Horino, T. Asao, and N. Inoue, Bull. Chem. Soc. Jpn., 1991, 64, 183. CrossRef
5. (a) K. H. H. Fabian, A. H. M. Elwahy, and K. Hafner, Tetrahedron Lett., 2000, 41, 2855; CrossRef (b) S. Ito, A. Nomura, N. Morita, C. Kabuto, H. Kobayashi, S. Maejima, K. Fujimori, and M. Yasunami, J. Org. Chem., 2002, 67, 7295; CrossRef (c) K. H. H. Fabian, A. H. M. Elwahy, and K. Hafner, Eur. J. Org. Chem., 2006, 791. CrossRef
6. R. Yokoyama, S. Ito, T. Okujima, T. Kubo, M. Yasunami, A. Tajiri, and N. Morita, Tetrahedron, 2003, 59, 8191. CrossRef
7. (a) S. Ito, H. Inabe, T. Okujima, N. Morita, M. Watanabe, and K. Imafuku, Tetrahedron Lett., 2000, 41, 8343; CrossRef (b) S. Ito, H. Inabe, T. Okujima, N. Morita, M. Watanabe, N. Harada, and K. Imafuku, Tetrahedron Lett., 2001, 42, 1085; CrossRef (c) S. Ito, H. Inabe, T. Okujima, N. Morita, M. Watanabe, N. Harada, and K. Imafuku, J. Org. Chem., 2001, 66, 7090; CrossRef (d) S. Ito, T. Okujima, and N. Morita, Tetrahedron Lett., 2002, 43, 1261; CrossRef (e) S. Ito, Terazono, T. Kubo, T. Okujima, N. Morita, T. Murafuji, Y. Sugihara, K. Fujimori, J. Kawakami, and A. Tajiri, Tetrahedron, 2004, 60, 5357; CrossRef (f) T. Shoji, S. Kikuchi, S. Ito and N. Morita, Heterocycles, 2005, 66, 91; CrossRef (g) T. Shoji, S. Ito, K. Toyota, T. Iwamoto, M. Yasunami, and N. Morita, Eur. J. Org. Chem., 2009, 4307. CrossRef
8. (a) S. Ito, T. Okujima and N. Morita, J. Chem. Soc., Perkin Trans. 1, 2002, 1896; CrossRef (b) K. Kurotobi, M. Miyauchi, K. Takakura, T. Murafuji, and Y. Sugihara, Eur. J. Org. Chem., 2003, 3663; CrossRef (c) S. Ito, T. Terazono, T. Kubo, T. Okujima, N. Morita, T. Murafuji, Y. Sugihara, K. Fujimori, J. Kawakami, and A. Tajiri, Tetrahedron, 2004, 60, 5357; CrossRef (d) M. Fujinaga, T. Murafuji, K. Kurotobi, and Y. Sugihara, Tetrahedron, 2009, 65, 7115. CrossRef
9. (a) T. Shoji, R. Yokoyama, S. Ito, M. Watanabe, K. Toyota, M. Yasunami, and N. Morita, Tetrahedron Lett., 2007, 48, 1099; CrossRef (b) T. Shoji, S. Ito, M. Watanabe, K. Toyota, M. Yasunami, and N. Morita, Tetrahedron Lett., 2007, 48, 3009; CrossRef (c) T. Shoji, S. Ito, K. Toyota, M. Yasunami, and N. Morita, Tetrahedron Lett., 2007, 48, 4999; CrossRef (d) J. Higashi, T. Shoji, S. Ito, K. Toyota, M. Yasunami, and N. Morita, Eur. J. Org. Chem., 2008, 5823; CrossRef (e) T. Shoji, S. Ito, T. Okujima, J. Higashi, R. Yokoyama, K. Toyota, M. Yasunami, and N. Morita, Eur. J. Org. Chem., 2009, 1554; CrossRef (f) T. Shoji, S. Ito, K. Toyota, and N. Morita, Eur. J. Org. Chem., 2010, 1059; CrossRef (g) T. Shoji, S. Ito, J. Higashi, and N. Morita, Eur. J. Org. Chem., 2011, 5311; CrossRef (h) S. Ito, T. Shoji, and N. Morita, Synlett, 2011, 16, 2279. CrossRef
10. W. Bauer and U. Müller-Westerhoff, Tetrahedron Lett., 1972, 1021. CrossRef
11. T. Shoji, J. Higashi, S. Ito, K. Toyota, T. Asao, M. Yasunami, K. Fujimori, and N. Morita, Eur. J. Org. Chem., 2008, 1242. CrossRef
12. T. Shoji, K. Okada, S. Ito, K. Toyota, and N. Morita, Tetrahedron Lett., 2010, 51, 5127. CrossRef
13. General procedure for the synthesis of 6-substituted-1,3-di(methylthio)azulenes 1b, 1c and 1d. To a solution of 6-substituted azulene derivatives and 5.0 equiv. DMSO in CH2Cl2 was added dropwise a solution of 2.4 equiv. Tf2O in CH2Cl2. The resulting solution was stirred for 30 min at room temperature. The solvent was removed under reduced pressure. EtOH and Et3N were added to the residue and the mixture was refluxed for 2 h. The solvent was removed under reduced pressure. The residue was purified by column chromatography on silica gel to give the corresponding 6-substituted-1,3-di(methylthio)azulenes 1b, 1c and 1d, respectively (R = H, 98% yield; R = tert-Bu, 96% yield; R = OMe, 97% yield).
14. General procedure for the synthesis of 6-dimethylamino-1,3-di(methylthio)- 2-(dihydroheteroaryl)azulenes 2–7. To a solution of -dimethylamino-1,3-di(methylthio)azulene and 5.0 equiv. heterocycles in CH2Cl2 was added dropwise a solution of 1.5 equiv. Tf2O in CH2Cl2. After the resulting solution was stirred for 1–3 h at 0 °C, the solvent was removed in vacuo. The products were purified by column chromatography on silica gel to give the corresponding 6-dimethylamino-1,3-di(methylthio)-2-(dihydroheteroaryl)azulenes 2–7 (yield of the products is shown in Table 2).
15. General procedure for the preparation of 6-dimethylamino-1,3-di(methylthio)-2-heteroarylazulenes 8–11. To a solution of 2–7 in MeOH (or i-PrOH) was added potassium hydroxide. After the resulting mixture was refluxed for 5–17 h, the reaction mixture was poured into brine and extracted with EtOAc. The crude product was purified by column chromatography on alumina to afford corresponding 6-dimethylamino-1,3-di(methylthio)-2-heteroarylazulenes 8–11 (the yield of the products is shown in Table 3).
16. S. Ito and N. Morita, Eur. J. Org. Chem., 2009, 4567. CrossRef