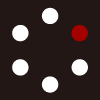
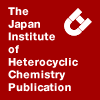
HETEROCYCLES
An International Journal for Reviews and Communications in Heterocyclic ChemistryWeb Edition ISSN: 1881-0942
Published online by The Japan Institute of Heterocyclic Chemistry
e-Journal
Full Text HTML
Received, 19th October, 2011, Accepted, 24th November, 2011, Published online, 30th November, 2011.
DOI: 10.3987/COM-11-12374
■ Polycyclic Quinolones (Part 1) — Thieno[2,3-b]benzo[h]quinoline Derivatives: Design, Synthesis, Preliminary in vitro and in silico Studies
Abeer Ahmed and Mohsen Daneshtalab*
School of Pharmacy, Memorial University of Newfoundland, St. John’s, NL, A1B 3V6, Canada
Abstract
Heterocyclic systems with a quinoline nucleus represent the most spectacular example of privileged molecules in medicinal chemistry, as their biological activities are surely affected by changes in structural features. Quinoline derivatives have been shown to display a wide spectrum of biological activities such as antibacterial, antifungal, antiparasitic, antiviral, cytotoxic and anti-inflammatory activities. In this study, several 7-hydroxy-8-oxo-8,9-dihydrobenzo[h]thieno[2,3-b]quinoline-9-carboxylic acids were designed, synthesized, and were further subjected to chemical reactions such as alkylation and annelation. The synthesized compounds were also subjected to docking study and biological evaluation. This work was mainly designed to shed light on the requirements for the quinoline nucleus to act as an anticancer agent. Unexpectedly, the synthesized derivatives showed weak or no cytotoxicity against cancer cell lines and the increase in the extent of aromatic/condensed rings did not increase the affinity toward the double stranded DNA. Our virtual screening demonstrated that the chelation with Mg2+ is a determining factor in the expected interaction with Topoisomerases. Key synthetic issues, crystallographic and docking studies have also been described.INTRODUCTION
Quinoline derivatives represent a major class of heterocycles. The quinoline ring system occurs in various natural products, especially in alkaloids. Its skeleton is often used for the design of many synthetic compounds with diverse pharmacological properties.1 The 4(1H)-quinolone-3-carboxylic acid is one of the most studied quinoline-originated multivalent scaffold that has been used in constructing a diverse series of biologically active compounds such as antibacterials, anticancers and antiviral agents.2,3
In a search for novel small molecule heterocycles with potential antineoplastic activity, a joint patent by the Japanese pharmaceutical companies Kyorin/Kyowa-Hakko, describing thiazoloquinolone carboxylic acids with an impressive anticancer profile (compound A) attracted our attention.4 The clinical candidate compound had exhibited favourable drug-like properties in different animal models in preclinical studies.
Compound A, like other quinolone carboxylic acid derivatives, was presumed to inhibit the topoisomerase II enzyme via its β-keto acid functional group and the chelation with the Mg2+ ion. Considering this mode of action,5 we hypothesized that a quinolone with a β-diketo functionality may be able to mimic the action of β-keto acid functionality, thus potentially providing the same level of complexation with the Mg2+ ion at the active site of the topoisomerase II.
The target compounds were designed using the Hyperchem-3TM molecular modeling program. By applying Molecular Mechanics Optimization (MMO) and Molecular Dynamic Option methods we were able to identify linear tricyclic quinolones with β-diketo components that matched the angular feature of Compound A. In this respect, compound B, 9-benzyl-7-fluoro-3-hydroxythieno[4,5-b]quinoline-4(9H)-one, at its optimized steric/energetic configuration, was found to have the best match with a perfect 3-point overlay with compound A.
Compound B and its derivatives were synthesized in our group using the Gould-Jacob method. This series of compounds displayed promising cytotoxic activity against several cancer cell lines.6
During our initial studies we found out that the thiazoloquinolonecarboxylic acid derivatives demonstrated anti-neoplastic activity even without the presence of the required substituent at position 7 (aromatic and aliphatic amine substituents for compounds targeting euokaryotic topoisomerase II),7-11 and also with the absence of mandatory fluorine atoms at C-6 and C-8 positions.9,11,12
Antitumor cytotoxic agents possessing DNA-intercalative properties are characterized by the presence of a planar pharmacophore, generally a tri- or tetracyclic ring system and one or two flexible substituent groups.13 Compounds possessing a large coplanar aromatic chromophore are likely to intercalate reversibly to the double helical DNA. Most derivatives are not obviously related in any way except in sharing the exhibition of coplanar chromophores that favor the intercalation; for this reason they are designated as DNA intercalators. Moreover, the cytotoxicity of most of the clinically useful DNA-intercalating agents involve the inhibition of the enzyme DNA-topoisomerase I or II.14
Considering the above discussion, our first target was annelation of a thieno ring to the benzo[h]quinoline nucleus in an angular pattern. In this context, we attempted the syntheses of benzo[h]thieno[2,3-b]quinoline derivatives.
As a starting point, the synthesis of ethyl 7-hydroxy-8-oxo-8,9-dihydrobenzo[h]thieno[2,3-b]quinoline-9-carboxylate (6a) was attempted, as illustrated in Scheme 1. Namely, 1-naphthylisothiocyanate was used as a main starting material which, by reacting with diethylmalonate and sodium hydride in acetonitrile, afforded the salt 1a. This intermediate was allowed to react with different reagents to give rise to intermediates 2. Thermal cyclization under vacuum enabled us to cyclize compounds 2 in very good yields (up to 80%). Dieckmann cyclization of compound 3a afforded compound 6a, saponification of which, gave rise to the carboxylic acid derivative 6b.
O-ALKYLATED PRODUCTS
Alkylation of compounds 3a and 3b using either traditional nucleophilic substitution or Mitsunobu reaction afforded only the corresponding O-alkylated products 3g-3i, as illustrated in Scheme 2.
Preparation of the 2-cyano analogue of 6a (compound 8) was proceeded via dealkylation of 3b to afford compound 4, which was then subjected to alkylation reaction with bromoacetonitrile to yield compound 3k. This compound was then subjected to Dieckmann cyclization to give rise to the target compound 8, as depicted in Scheme 3.
Alkylation reactions were also attempted on compound 6a using diethyl/dimethyl sulfate in different proportions, and 1,2-dibromoethane. Interestingly, all the reactions produced the O-alkylated derivatives (6c-6f) as illustrated in Scheme 4.
In order to explore the effect of isosteric replacement of thiophene ring with other 5-membered heterocycles on the biological activity of thienoquinolines, we attempted annelation of the isothiazole ring onto the quinoline moiety constructed in previous steps. In this context, we tried the synthesis of isothiazolobenzo[h]quinoline using the reported transamination of 2-sulfenamoyl-benzoates with various amines. Among several reported procedures for preparation of –SNH2 derivative,15-18 the only successful method was reaction of thiol intermediate 4 with hydroxylamine-O-sulfonic acid to yield the corresponding thioamino derivative 5, which upon Dieckman cyclization afforded compound 7, as shown in Scheme 5.
In another attempt to increase the hydrophilicity of the quinoline system, we allowed compound 6a to react with hydrazine hydrate to yield the pentacyclic pyrazoloquinoline 9. The pentacyclic benzo[h]quinoline 9 was formed as a result of reaction of 6a with hydrazine hydrate and cyclization in acetic anhydride solution as illustrated in Scheme 6. The structure of compound 9 was confirmed by spectral analysis and X-ray crystallography, as shown in Figure 2.
The synthesis of compound 9 prompted us to further explore the effect of annelating different ring systems with quinoline-based building blocks which may possibly result in identification of novel polycyclic molecules with potential bioactivity. In this context, we attempted the synthesis of 1H-3-thia-11c-azaazuleno[1,8,7,6-cdef]phenanthrene-1,6(5H)-dione (11). In this structure, a thiazoloazepinone moiety was annelated to the benzoquinoline system.
The synthesis of compound 11 was achieved via the reaction of 1 with 4-chloroethylacetoacetate, followed by thermal cyclization under vacuum to afford compound 10. This compound was then subjected to 3 h cyclization reaction using PPA to yield the novel phenanthrene derivative 11 as shown in Scheme 7. The X-ray structure of compound 10 is illustrated in Figure 3.
The synthesized compounds were screened for toxicity using Brine Shrimp Lethality Assay method according to Meyer’s procedure,19 and the most toxic compounds were subjected to MTT cytotoxicity assay.20,21
The MTT assay revealed that the most toxic compounds (6a, 7, 8) in the Brine Shrimp Lethality Assay did not demonstrate acceptable cytotoxicity and can not be considered as potential lead compounds to pursue its anticancer profile. This conclusion prompted us to explore the possible causes of of weak cytotoxic profile of these compounds by carrying out an in silico study.
MOLECULAR MODELING
METHODS
Docking calculations were carried out according to the DockingServer methodology.22 The MMFF94 force field23 was used for energy minimization of the ligand molecule using DockingServer. PM6 semiempirical charges calculated by MOPAC2009 (J. P. Stewart, Computer code MOPAC2009, Stewart Computational Chemistry, 2009) were added to the ligand atoms. Non-polar hydrogen atoms and rotatable bonds were defined.
Docking calculations were carried out on topoisomerase II structure with the pdb code 1QZR. Essential hydrogen atoms, Kollman united atom type charges, and solvation parameters were added with the aid of AutoDock tools.24 Affinity (grid) maps with 25×25×25 Å grid points and 0.375 Å spacing were generated using the Autogrid program. AutoDock parameter set- and distance-dependent dielectric functions were used in the calculation of the van der Waals and the electrostatic terms, respectively.
Docking simulations were performed using the Lamarckian genetic algorithm (LGA) and the Solis & Wets local search method.25 Initial position, orientation, and torsions of the ligand molecules were set randomly. Each docking experiment was derived from 100 different runs that were set to terminate after a maximum of 2,500,000 energy evaluations. The population size was set to 150. During the search, a translational step of 0.2 Å, and quaternion and torsion steps of 5 were applied.
DOCKING EXPERIMENT
In order to define possible binding sites in the protein, the known human topoisomerase II inhibitor, compound A, was docked into the crystal structure. First, blind docking procedure was applied. The docking result showed no cluster possessing significantly higher frequency or lower energy as compared to the other clusters. Thus, blind docking in this case was not able to clearly define the possible binding site. Therefore, biochemical knowledge was used for binding site identification. The cluster possessing the highest interaction surface between the docked ligand and the protein indicating strong binding was located at the ATP binding site. Thus, focused docking calculations were carried out at the ATP binding site of the protein.
FOCUSED DOCKING RESULTS
Compound A was subjected to focused docking calculations at the ATP binding site of the protein with docking energy equal to -7.39 kcal/mol.
All compounds were proved to bind at the ATP binding site with reasonable affinity. Figure 4 shows the binding of compound 6a which possesses the lowest docking energy. It is noted that in the X-ray structure Mg2+ is coordinated by 3 oxygen atoms coming from ANP. Compound A is also able to donate non-bonding electrons to the Mg2+ ion. Figure 5 illustrates overlapping image of compounds 6a (green colour) and A (blue colour) at the ATP binding site of 1QZR. Table 1 summarizes the docking energies of the investigated compounds at the ATP binding site
The estimated binding energies can be used to rank the ligands according to their binding affinity.
Compound 6a indicates a hydrogen bonding interaction with ASN70 and LYS147. Namely, the amino group of ASN70 donates a hydrogen bond to the aromatic nitrogen and the protonated nitrogen of LYS147 donates a hydrogen bond to the ester carbonyl of 6a. The aromatic planar ring system of 6a is stabilized by a number of hydrophobic interactions with surrounding hydrophobic amino acid residues at the binding site: ILE67, ILE120, ALA71, VAL197 and with the aromatic side chain of PHE121. It is important to note that none of the compounds in this series are involved in interaction with Mg2+ in contrast to compound A.
Focused docking calculations at the ATP binding site revealed that the investigated compounds are able to bind to human topoisomerase II with a reasonable binding affinity. The main difference between compound A and the investigated compounds is in its ability to coordinate with Mg2+ ion and that explains the potent cytotoxic and topoisomerase II inhibitory effect of compound A, compared to weak cytotoxic effects demonstrated by the compounds synthesized in our study.
CONCLUSION
Quinoline system is considered as an attractive scaffold to build chemical libraries with promising bioactivity potentials. Its unique structural feature has prompted scientists to design, synthesize, and evaluate different quinoline-based structures which exhibit diverse biological activities.
In this study, we attempted designing novel polycyclic quinoline-based molecules with structural relationship to a molecule that is under clinical investigation as an antineoplastic agent. The structure-based molecular modeling approach led us to ethyl 7-hydroxy-8-oxo-8,9-dihydrobenzo[h]- thieno[2,3-b]quinoline-9-carboxylate (6a). We successfully synthesized a series of 6a analogues and tested them for cyctotoxicity against different cancer cell lines. The test results revealed the lack of cytotoxic potency by these compounds. In order to rationalize the non-toxic profile of these compounds, we attempted docking experiments with topisomerase II protein structure, using compound A, as a standard-reference topoisomerase II inhibitor. The docking experiments revealed that while the synthesized compounds interact with the protein carrying ATP binding region, they do not coordinate with Magnesium ion. While, compound A makes a stable coordination with Mg2+ ion. This may explain the possible weak cytotoxicity demonstrated by the synthesized compounds. Further bioassays of these compounds to find other potential bioactivities are ongoing in our group.
EXPERIMENTAL
GENERAL
Solvents and reagents were obtained from commercial suppliers and were used without further purification. Melting points were not optimized, 1H and 13C NMR spectra, HSQC, and COSY spectra were recorded on a Bruker AVANCE-500 MHz NMR spectrometer using TMS as an internal standard. Mass spectra were obtained on an Agilent 1100 series LC/MSD chromatographic system. High-resolution mass spectra (EI or ESI) were obtained on Waters GCT Premier Micromass Spectrometer. X-Ray structures were measured with on Rigaku Saturn 70 instrument, equipped with a CCD area detector and a SHINE optic, using Mo Kα radiation. Silicycle Ultrapure silica gel (0–20 μm) G and F-254 was used for the preparative-layer TLC, and Silicycle Silia-P Ultrapure Flash silica gel (40–63 μm) was used for flash column chromatography. TLC was conducted on Polygram SIL G/UV254 precoated plastic sheets.
Sodium 3-ethoxy-2-(ethoxycarbonyl)-1-(naphthalen-1-ylamino)-3-oxoprop-1-ene-1-thiolate (1a). To a suspension of sodium hydride (0.6 g, 25 mmol) in MeCN (50 mL) at 5–10 ºC was added dropwise diethyl malonate (4 mL, 26.34 mmol) over a period of 15 min. The mixture was stirred at 5–10 ºC for 30 min, then 1-naphthylisothiocyanate (5.0 g, 26.99 mmol) was added portionwise at the same temperature, and stirring was continued for extra 30 min. Evaporation of MeCN yielded a yellowish solid which was washed with Et2O; mp 118–120 ºC; 1H-NMR: (500 MHz, DMSO-d6): δ = 12.33 (s, NH), 8.57 (d, J = 7.1 Hz, 1H), 8.16 (t, J = 7.7 Hz, 1H), 7.91–7.86 (m,1H), 7.52 (ddd, J = 4.7, 11.1, 8.8 Hz, 3H), 7.46–7.38 (m, 1H), 4.02 (q, 2 X OCH2CH3, J = 7.0 Hz, 4H), 1.16 (t, 2 X OCH2CH3, J = 7.0 Hz, 6H); 13C NMR (175 MHz, DMSO-d6): δ = 181.65, 166.45, 136.18, 132.58, 127.07, 126.67, 124.36, 124.27, 123.99, 121.21, 120.66, 119.49, 116.89, 95.27, 56.88 (2CH2), 13.37 (2CH3); APCI-MS: 368.40 (M++1, 100).
Ethyl 2-[(ethoxymethyl)thio]-4-hydroxybenzo[h]quinoline-3-carboxylate (3a). To the above yellow solid (1a, 2.0 g, 5.44 mmol) in THF (50 mL) was added BrCH2CO2Et (0.6 mL, 5.44 mmol) dropwise at 0 ºC and stirring was continued for an extra hour at room temperature, evaporated the solvent, extracted with CHCl3 and dried over Na2SO4. The organic layer was evaporated by rotary evaporator to give yellow oil (2a). The obtained oil was heated at 170–180 ºC in an oil bath under vacuum for 10 min. The resulting oil was solidified, then washed with ether resulting in (3a) as white needles; mp 136–138 ºC; 1H-NMR: (500 MHz, CDCl3): δ = 13.11 (s, 1H, OH), 9.22-9.15 (m, 1H), 8.10 (d, J = 8.9 Hz, 1H), 7.89 (dd, J = 3.1, 6.0 Hz, 1H), 7.76-7.68 (m, 3H), 4.61 (q, J = 7.0, 6.9 Hz, 2H), 4.22 (q, J = 7.0, 6.9 Hz, 2H), 4.13 (s, 2H), 1.60 (t, J = 7.0 Hz, 3H), 1.29 (t, J = 7.0 Hz, 3H); 13C NMR (175 MHz, CDCl3): δ = 170.99, 170.46, 168.29, 158.14, 147.62, 135.89, 130.69, 129.47, 128.19, 127.15, 126.27, 125.97, 119.97, 115.10, 104.00, 63.29, 61.91, 34.65, 14.66, 14.62; HR-MS (TOFEI) calcd for C20H19NO5S (385.0984); found (385.0991).
Ethyl 2-[(ethoxymethyl)thio]-4-hydroxybenzo[h]quinoline-3-carboxylate (3b). This compound was prepared according to the same procedure as that applied for 3a using chloromethyl ethyl ether; yellow crystals; mp 120–122 ºC; 1H-NMR: (500 MHz, CDCl3): δ = 13.11 (s, 1H), 9.26–9.19 (m, 1H), 8.13 (d, J = 9.0 Hz, 1H), 7.91 (d, J = 3.3 Hz, 1H), 7.75 (d, J = 9.0 Hz, 1H), 7.72 (dd, J = 3.2, 6.1 Hz, 2H), 5.76 (s, 2H), 4.60 (q, J = 7.1 Hz, 2H), 3.76 (q, J = 7.0 Hz, 2H), 1.59 (t, J = 7.0 Hz, 3H), 1.25 (t, J = 7.0 Hz, 3H); 13C NMR (175 MHz, CDCl3): δ = 171.17, 168.31, 158.23, 147.41, 135.88, 129.49, 128.24, 127.33, 126.21, 125.73, 120.02, 115.14, 104.38, 71.49, 65.78, 63.14, 31.29, 15.47, 14.61; HR-MS (TOFEI): calcd for C19H19NO4S (357.1035); found (357.1031).
Ethyl 4-hydroxy-2-(methylthio)benzo[h]quinoline-3-carboxylate (3c). Prepared according to the method applied for 3b, using 1equivalent MeI; yellow powder; mp 158–160 ºC; 1H-NMR: (500 MHz, CDCl3): δ = 13.09 (s, 1H, OH), 9.22–9.16 (m, 1H), 8.10 (d, J = 8.9 Hz, 1H), 7.87 (dd, J = 2.8, 6.0 Hz, 1H), 7.71 (d, J = 8.8 Hz, 1H), 7.69–7.65 (m, 2H), 4.57 (q, J = 7.2 Hz, 2H), 2.81 (s, 3H), 1.57 (t, J = 7.25 Hz, 3H); 13C NMR (175 MHz, CDCl3): δ = 170.97, 167.71, 160.16, 147.03, 135.45, 130.46, 128.99, 127.80, 126.74, 125.42, 125.31, 119.68, 114.21, 103.77, 62.63, 15.14, 14.27; HR-MS (TOFEI) calcd for C17H15NO3S (313.0773); found (313.0779).
Ethyl 4-hydroxy-2-[(4-methoxybenzyl)thio]benzo[h]quinoline-3-carboxylate (3d). Prepared according to the method applied for 3b, using 1 equivalent P-MeOBnCl; white crystals; mp 135–136 ºC; 1H-NMR: (500 MHz, CDCl3): δ = 13.14 (s, 1H, OH), 9.16 (dd, J = 8.2, 5.9 Hz, 1H), 8.10 (d, J = 9.0 Hz, 1H), 7.88 (dd, J = 6.7, 2.4 Hz, 1H), 7.75-7.69 (m, 1H), 7.66 (dt, J = 7.0, 3.9 Hz, 2H), 7.43 (t, J = 5.8 Hz, 2H), 7.25 (s, 1H), 6.85 (dd, J = 9.2, 2.5 Hz, 2H), 4.70 (s, 2H), 4.53 (q, J = 7.1 Hz, 2H), 3.83 (s, 3H), 1.50 (t, J = 7.2 Hz, 3H); 13C NMR (175 MHz, CDCl3): δ = 170.89, 167.83, 159.74, 158.77, 147.09, 135.52, 130.42, 130.39, 129.79, 129.07, 127.88, 126.88, 125.58, 125.35, 123.12, 121.25, 119.73, 114.54, 114.02, 103.53, 62.70, 55.29, 35.42, 14.31; HR-MS (TOFEI) calcd for C24H21NO4S (419.1191); found (419.1191).
Ethyl 4-hydroxy-2-{[(2Z)-3-phenylprop-2-en-1-yl]thio}benzo[h]quinoline-3-carboxylate (3e). Prepared according to the method applied for 3b, using 1 equivalent ClCH2C6H5CH=CH; white powder; mp 157–159 ºC; 1H-NMR: (500 MHz, CDCl3): δ = 13.13 (s, 1H, OH), 9.21 (m, 1H), 8.12 (d, J = 8.8 Hz, 1H), 7.91 (m, 1H), 7.74 (d, J = 9.1 Hz, 1H), 7.71 (2H, m), 7.35 (d, J = 7.2 Hz, 2H), 7.26 (m, 3H), 7.20 (d, J = 7.2 Hz, 1H) 6.76 (d, J = 7.5 Hz, 1H), 6.60 (m, 1H), 4.58 (q, J = 7.1, 7.0 Hz, 2H), 4.28 (d, J = 5.6 Hz 2H), 1.56 (t, J = 5.8 Hz, 3H); 13C NMR (175 MHz, CDCl3): δ = 165.61, 162.64, 153.83, 141.88, 131.83, 130.28, 127.61, 125.23, 123.83, 123.29, 122.65, 122.21, 121.69, 121.12, 120.53, 120.35, 120.04, 119.19, 114.47, 109.24, 98.46,57.50, 28.77, 15.07, 9.07; HR-MS (TOFEI) calcd for C25H21NO3S (415.1242); found (415.1246).
Ethyl 2-(benzylthio)-4-hydroxybenzo[h]quinoline-3-carboxylate (3f). Prepared according to the method applied for 3b, using 1 equivalent BnCl; white crystalline product; mp 130–132 ºC; 1H-NMR: (500 MHz, CDCl3): δ = 13.07 (s,1H, OH), 9.10 (dd, J = 8.3, 6.6 Hz, 1H), 8.09–8.04 (m, 1H), 7.88–7.82 (m, 1H), 7.68 (d, J = 8.9 Hz, 2H), 7.66–7.59 (m, 1H), 7.52 (d, J = 7.1 Hz, 2H), 7.36–7.29 (m, 2H) 7.28–7.22 (m, 1H), 4.73 (s, 2H), 4.52 (q, J = 7.1 Hz, 2H), 1.49 (t, J = 7.0 Hz, 3H); 13C NMR (175 MHz, CDCl3): δ = 170.85, 167.82, 159.48, 147.04, 138.01, 135.50, 130.39, 129.29, 129.05, 128.59, 127.85, 127.09, 126.84, 125.60, 125.35, 123.23, 121.12, 119.70, 114.54, 103.50, 62.72, 35.95, 14.32; HR-MS (TOFEI) calcd for C23H19NO3S (389.1086); found (389.1093).
Ethyl 4-(3-bromopropoxy)-2-((2-ethoxy-2-oxoethyl)thio)benzo[h]quinoline-3-carboxylate (3g). To a mixture of the quinoline derivative 3a (0.385 g, 1 mmol) and K2CO3 (0.386 g, 2.8 mmol) in dry DMF (25 mL) under nitrogen atmosphere, 1,3-dibromopropane (0.565 g, 2.8 mmol) and KI (catalytic amount) were added. The reaction mixture was heated at 70 ºC for 24 h, then poured into ice-H2O, the resulting product was collected by filtration and recrystalized from hexane:CHCl3 (1:3) resulting in a white powder; mp 162–164 ○C; 1H-NMR: (500 MHz, CDCl3): δ = 9.21–9.14 (m, 1H), 7.95 (d, J = 8.9 Hz, 1H), 7.91–7.85 (m, 1H), 7.76 (d, J = 8.9 Hz, 1H), 7.70 (m, 2H), 4.53 (q, J = 7.1 Hz, 2H), 4.35 (t, J = 5.8 Hz, 2H), 4.22 (q, J = 7.1 Hz, 2H), 4.15 ( s, 2H), 3.70 (t, J = 6.4 Hz, 2H), 2.42 (q, J = 6.1 Hz, 2H), 1.49 (t, J = 7.1 Hz, 3H), 1.27 (t, J = 7.1 Hz, 3H); 13C NMR (175 MHz, CDCl3): δ = 162.11, 160.69, 160.25, 153.77, 148.08, 134.29, 130.22, 129.08, 127.61, 127.07, 125.94, 125.47, 119.97, 115.82, 113.80, 70.98, 70.01, 61.21, 33.81, 29.86, 25.01, 14.43, 14.31; APCI-MS: 506.40 (M++1, 100).
Ethyl 4-ethoxy-2-[(ethoxymethyl)thio]benzo[h]quinoline-3-carboxylate (3h). Utilizing the same alkylation procedure as applied for preparation of 3g using diethylsulfate starting from 3b; white crystals; mp 80–82 ºC; 1H-NMR: (500 MHz, CDCl3): δ = 9.17 (m, 1H), 8.07 (m, 1H), 7.98 (dd, J = 9.0, 22.2 Hz, 2H), 7.81 (m, 2H), 5.76 (s, 2H), 4.46 (q, J = 7.0 Hz, 2H), 4.29 (q, J = 6.9 Hz, 2H), 3.61 (q, J = 7.0, 2H), 1.45 (t, J = 6.9 Hz, 3H), 1.39 (t, J = 7.1 Hz, 3H), 1.11 (t, J = 7.0 Hz, 3H); 13C NMR (175MHz, CDCl3): δ = 166.25, 163.20, 160.74, 154.97, 147.39, 134.92, 132.90, 131.95, 129.10, 128.97, 127.01, 125.21, 120.13, 119.55, 118.87, 72.10, 65.30, 62.94, 16.36, 15.65, 14.83; HR-MS (TOFEI) calcd for C21H23NSO4 (385.1426); found (385.1441).
Ethyl 4-(benzyloxy)-2-[(2-ethoxy-2-oxoethyl)thio]benzo[h]quinoline-3-carboxylate (3i). A 250 mL round bottom flask was charged with triphenylphosphine (2.7 g, 10.3 mmol) to which was added 70 mL THF. The resulting clear solution was cooled to -78 ºC and DEAD (1.63 mL, 10.3 mmol) was added over 5 min. The yellow reaction mixture was stirred for extra 5 min after which benzyl alcohol (1.169 mL, 11.3 mmol) was added and stirred for 5 min. Neopentyl alcohol (0.50 g, 5.7 mmol) and 3a (3.96 g, 10.3 mmol) were added sequentially to the reaction mixture as solids. The resulting suspension was allowed to remain at -78 ºC for 5 min during which most of the starting material dissolved. The cooling bath was then removed and the reaction was stirred overnight at room temperature, TLC indicated complete consumption of the reactant. The clear solution was concentrated to approximately ¼ of the original volume under vaccum then applied to a silica gel column and eluted with 5:1 hexane/EtOAc resulting in a white crystalline product; mp 105–107 ºC; 1H-NMR: (500 MHz, CDCl3): δ = 9.08 (m, 1H), 8.05 (m, 1H), 7.94 (m, 2H), 7.81 (m, 2H), 7.79 (m, 2H), 7.41 (m, 3H), 5.26 (s, 2H), 4.44 (q, J = 5.3 Hz, 2H), 4.25 (s, 2H), 4.12 (q, J = 5.1 Hz, 2H), 1.35 (t, J = 6.8 Hz, 3H), 1.21 (t, J = 7.5 Hz, 3H); 13C NMR (175 MHz, CDCl3): δ = 166.28, 160.67, 154.90, 147.41, 134.38, 130.60, 128.74, 127.80, 127.10, 126.65, 124.99, 119.47, 118.59 (8 carbons), 71.90, 71.25, 65.13, 62.16, 15.73, 14.97, 14.18; HR-MS (TOFEI) calcd for C27H25NO5S (475.1453); found (475.1458).
Ethyl 2-[(3-chloro-2-oxopropyl)thio]-4-hydroxybenzo[h]quinoline-3-carboxylate (3j). Utilizing the same alkylation procedure as applied for preparation of 3b using 1,3-dichloroacetone; yellowish brown powder; mp 98–100 ºC; 1H-NMR: (500 MHz, CDCl3): δ = 13.09 (s, OH), 8.93 (d, J = 8.5 Hz, 1H), 8.10 (d, J = 8.9 Hz, 1H), 7.89 (d, J = 7.3 Hz, 1H), 7.79-7.69 ( m, 3H), 4.61 (q, J = 7.16, 7.17 Hz, 2H), 4.40 (s, 2H), 4.32 (s, 2H), 1.58 (t, J = 7.1 Hz, 3H); 13C NMR (175 MHz, CDCl3): δ = 169.36, 168.04, 164.36, 157.46, 146.21, 134.68, 129.38, 129.24, 127.89, 126.92, 125.81, 124.64, 119.10, 114.30, 105.48, 35.69, 33.38, 14.02, 13.92; HR-MS (TOFEI) calcd for C19H16ClNO4S (389.0489); found (389.0495).
Ethyl 2-[(cyanomethyl)thio]-4-hydroxybenzo[h]quinoline-3-carboxylate (3k). To a stirring solution of 4 (0.8 g, 2.67 mmol) in THF (10 mL) and H2O (40 mL) was added NaHCO3 (1 g, 7.23 mmol), and stirred for 15 min, then bromoacetonitrile (0.5 g, 4.16 mmol) was added to the resulting solution and stirred for 4 h at room temperature. After completion of the reaction, the solution was acidified by acetic acid, extracted with chloroform, dried over Na2SO4, filtered to give 3k as a white powder; mp 208–210 ºC; 1H-NMR: (500 MHz, DMSO-d6): δ = 12.42 (s, 1H, OH), 9.26–9.21 (m, 1H), 8.12 (d, J = 8.8 Hz, 1H), 8.10–8.07 (m, 1H), 7.97 (d , J = 9.1 Hz, 1H), 7.82 (m, 2H), 4.53 (q, J = 7.0 Hz, 2H), 4.43 (s, 2H), 1.45 (t, J = 7.1 Hz, 3H); 13C NMR (175 MHz, DMSO-d6): δ = 169.97, 168.07, 155.09, 147.23, 135.59, 130.17, 129.48, 127.97, 127.41, 126.65, 125.31, 119.37, 117.49, 115.02, 103.45, 63.17, 17.27, 14.28; HR-MS (TOFEI) calcd. for C18H14N2O3S (338.0725); found (338.0722).
Ethyl 2-(aminothio)-4-hydroxybenzo[h]quinoline-3-carboxylate (5). To a stirring solution of 4 (0.8 g, 2.67 mmol) in THF (10 mL) and H2O (40 mL) was added NaHCO3 (1 g, 7.23 mmol), and stirred for 15 min, then hydroxylamine-O-sulfonic acid (0.50 g, 4.42 mmol) was added to the resulting solution and stirred for 4 h at room temperature. After completion of the reaction, the solution was acidified by acetic acid, extracted with CHCl3, dried over Na2SO4, filtered to give 5 as a white fluffy powder; mp 175–177 ºC; 1H-NMR: (500 MHz, DMSO-d6): δ = 8.54 (s, 1H, OH), 8.12 (t, J = 7.7 Hz, 2H), 7.86 (d, J = 8.7 Hz, 1H), 7.84–7.82 (m, 2H), 4.31 (q, J = 7.0 Hz, 2H), 1.33 (t, J = 7.0 Hz, 3H); 13C NMR (175 MHz, DMSO-d6): δ = 166.97, 162.57, 134.55, 128.96, 128.79, 127.95, 127.82, 127.52, 124.57, 121.53, 121.31, 105.48, 64.22, 61.66, 60.35, 14.31; HR-MS (TOFEI) calcd for C16H14N2O3S: (314.0725); found (314.0736).
Ethyl 7-hydroxy-8-oxo-8,9-dihydrobenzo[h]thieno[2,3-b]quinoline-9-carboxylate (6a). Sodium ethoxide (0.29 g, 4.3 mmol) was added to the solution of 3a (1.49g, 3.89 mmol) in EtOH (50 mL) and refluxed for 4 h. After completion of the reaction, EtOH was removed, added H2O (50 mL), and acidified by AcOH, separated solid was collected and dried as a yellowish powder; mp >250 ºC; 1H-NMR: (500 MHz, DMSO-d6): δ = 13.37 (s, 1H, OH), 8.74–8.69 (m, 1H), 8.16 (d, J = 8.8 Hz, 1H), 8.11 (dd, J = 6.8, 2.3 Hz, 1H), 7.87–7.83 (m, 2H), 7.84–7.79 (m, 1H), 4.29 (q, J = 7.0 Hz, 2H), 3.34 (s, 1H), 1.07 (t, J = 7.0 Hz, 3H). 13C NMR (175 MHz, DMSO-d6): δ = 175.31, 161.89, 157.05, 156.99, 150.56, 138.42, 134.91, 129.33, 128.84, 127.28, 123.86, 122.89, 120.52, 118.40, 114.34, 95.39, 60.29, 14.40; HR-MS (TOFEI) calcd. for C18H13NO4S (339.0565); found (339.0560).
7-Hydroxybenzo[h][1,2]thiazolo[5,4-b]quinolin-8(9H)-one (7). Sodium ethoxide (0.20 g, 3 mmol) was added to the solution of 5 (0.84 g, 2.7 mmol) in EtOH (50 mL) and refluxed for 4 h. After completion of the reaction, EtOH was removed, added H2O (50 mL), and acidified by AcOH, separated solid was collected and dried as a light brown powder; mp >250 ºC; 1H-NMR: (500 MHz, DMSO-d6): δ = 13.13 (s, 1H, OH), 8.61 (d, J = 7.8 Hz, 1H), 8.21 (d, J = 8.73 Hz, 1H), 8.14–8.11 (m, 1H), 7.85–7.79 (m, 3H); 13C NMR (175 MHz, DMSO-d6): δ = 172.90, 165.02, 162.24, 137.78, 134.91, 129.05, 128.61, 127.12, 125.23, 123.55, 123.06, 121.47, 120.27, 107.41; HR-MS (TOFEI) calcd. for C14H8N2O2S (268.0306); found (268.0310).
7-Hydroxy-8-oxo-8,9-dihydrobenzo[h]thieno[2,3-b]quinoline-9-carboxylic acid (6b). A mixture of ester (6a) (0.339 g, 1 mmol) and NaOH (0.088 g, 2.2 mmol) in H2O (20 mL) was stirred at 100 ○C for 4 h. After being cooled, the reaction mixture was neutralized with 1 M HCl, extracted with CH2Cl2 and dried. Evaporation followed by crystallization from EtOH afforded the acid as an orange red powder; mp >250 ○C; 1H-NMR: (500 MHz, DMSO-d6): δ = 13.10 (s,1H, OH), 12.95 (s, 1H, OH), 12.09 ( s, 1H, OH), 8.91 (d, J = 8.4Hz, 1H), 8.05 (d, J = 7.9 Hz, 1H), 7.96 (d, J = 8.8 Hz, 1H), 7.80 (t, J = 7.4 Hz, 1H), 7.76–7.68 (m, 2H); 13C NMR (175 MHz, DMSO-d6): δ = 166.24, 159.89, 158.27, 156.39, 149.46, 140.42, 133.91, 128.24, 127.35, 127.28, 123.56, 122.36, 120.49, 119.21, 115.34, 98.29; TOF-MS EI: calcd. for C16H9NO4S (311.16); found (311.16).
Ethyl 7-methoxy-8-oxo-8,9-dihydrobenzo[h]thieno[2,3-b]quinoline-9-carboxylate (6c). To a mixture of 6a (0.339 g,1 mmol) and K2CO3 (0.386 g, 2.8 mmol) in dry DMF (25 mL) under nitrogen atmosphere was added dimethyl sulfate (0.126 g, 1 mmol) along with KI (catalytic amount). The reaction mixture was heated at 70 ºC for 24 h, and then poured into ice-H2O. The resulting alkylated derivative was collected by filtration and recrystalized from hexane:CHCl3 (1:3) to afford a yellowish powder; mp charring ≥280 ºC; 1H-NMR: (500 MHz, DMSO-d6): δ = 9.15–9.07 (m, 1H), 8.11 (d, J = 9.0 Hz, 1H), 7.98 (dd, J = 2.7, 6.2 Hz, 1H), 7.75 (d, J = 9.1 Hz, 1H), 7.74–7.69 (m, 2H), 4.35 (s, 3H), 4.10 (q, J = 7.0 Hz, 2H), 1.25 (t, J = 7.0 Hz, 3H); 13C NMR (175 MHz, DMSO-d6): δ = 176.31, 160.89, 158.05, 155.91, 150.56, 139.42, 131.91, 128.33, 128.84, 125.28, 123.86, 122.89, 120.52, 118.40, 117.34, 95.39, 60.29, 47.21, 14.32; HR-MS (TOFEI) calcd for C19H15NO4S (353.0722); found (353.0731).
Ethyl 7-ethoxy-8-oxo-8,9-dihydrobenzo[h]thieno[2,3-b]quinoline-9-carboxylate (6d). Utilizing the same alkylation procedure as applied for preparation of 6c using diethyl sulfate (0.154 g, 1mmol); yellow powder; mp 170–172 ºC; 1H-NMR: (500 MHz, CDCl3): δ = 10.94 (s, 1H, OH), 9.35–9.28 (m, 1H), 8.16 (d, J = 9.1 Hz, 1H), 7.88 (dt, J = 3.2 Hz, 1H), 7.77–7.69 (m, 3H), 4.50 (q, J = 7.0 Hz, 2H), 4.46 (q, J = 7.1 Hz, 2H), 1.58 (t, J = 7.0 Hz, 3H), 1.45 (t, J = 7.1 Hz, 3H); 13C NMR (175 MHz, CDCl3): δ = 168.17, 161.14, 160.56, 157.93, 150.46, 134.80, 130.96, 129.66, 128.12, 127.55,126.90, 125.85, 120.24, 119.14, 116.44, 100.46, 74.24, 62.15, 16.18, 14.76; HR-MS (TOFEI): calcd for C20H17NO4S (367.0871); found (367.0872).
Ethyl 7,8-diethoxybenzo[h]thieno[2,3-b]quinoline-9-carboxylate (6e). Utilizing the same alkylation procedure as applied for preparation of 6d using diethyl sulfate (0.431 g, 2.8 mmol) orange powder; mp 120–122 ºC; 1H-NMR: (500 MHz, CDCl3): δ = 9.41–9.29 (m, 1H), 8.13 (d, J = 9.1 Hz, 1H), 7.94–7.87 (m, 1H), 7.80–7.69 (m, 3H), 4.44 (q, J = 7.0 Hz, 4H), 4.39 (q, J = 7.0 Hz, 2H), 1.56 (t, J = 6.6 Hz, 6H), 1.45 (t, J = 7.1 Hz, 3H); 13C NMR (175 MHz, CDCl3): δ = 162.27, 160.22, 159.97, 153.38, 149.86, 134.58, 130.54, 129.00, 128.11, 128.00, 127.58, 125.27, 119.49, 119.25, 118.89, 116.62, 73.66, 72.23, 61.34, 15.59, 15.33, 14.23; HR-MS (TOFEI) calcd for C22H21NO4S (395.1191); found (395.1195).
Ethyl 8,9-dihydro-7,10-dioxa-12-thia-13-azaazuleno[8,1-ab]phenanthrene-11-carboxylate (6f). This compound was prepared using the same procedure as that used for the synthesis of 6e using 1,2-dibromoethane to afford a yellow crystalline product; mp 250–252 ºC; 1H-NMR: (500 MHz, CDCl3): δ = 9.35–9.28 (m, 1H), 8.14 (d, J = 9.1 Hz, 1H), 7.93–7.87 (m, 1H), 7.74 (dt, J = 5.9, 9.7 Hz, 3H), 4.96–4.92 (m, 2H), 4.84–4.79 (m, 2H), 4.42 (q, J = 7.1 Hz, 2H), 1.43 (t, J = 7.1 Hz, 3H); 13C NMR (175 MHz, CDCl3): δ = 162.14, 159.55, 158.21, 152.80, 148.32, 134.34, 130.16, 129.10, 127.63, 127.08, 125.82, 125.48, 119.48, 113.87, 111.47, 104.81, 73.66, 72.21, 61.15, 14.42; HR-MS (TOFEI) calcd for C20H15NO4S (365.0722); found (365.0727).
9-Cyano-7-hydroxy-8-oxo-8,9-dihydrobenzo[h]thieno[2,3-b]quinoline (8). Sodium ethoxide (0.20 g, 3 mmol) was added to the solution of 3k (0.91 g, 2.7 mmol) in EtOH (50 mL) and refluxed for 4 h. After completion of the reaction, EtOH was removed, added H2O (50 mL), and acidified by AcOH; the separated solid was collected and dried as a brown powder; mp >250 ºC; 1H-NMR: (500 MHz, DMSO-d6): δ = 13.39 (s, OH), 8.66 (s, 1H), 8.16 (d, J = 8.7 Hz, 1H), 8.10 (d, J = 9.1Hz, 1H), 7.83 (dd, J = 6.8, 14.0 Hz, 4H); 13C NMR (175 MHz, DMSO-d6): δ = 173.40, 165.25, 167.01, 162.58, 140.01, 138.78, 135.26, 130.05, 129.41, 127.45, 125.13, 124.55, 122.06, 121.34, 120.12, 105.32; HR-MS (TOFEI) calcd. for C16H8N2O2S (292.3019); found (292.3017).
9-Acetyl-9H-benzo[h]pyrazolo[3',4':4,5]thieno[2,3-b]quinoline-7,10-diyl diacetate (9). Compound 6a (0.50 g, 1.5 mmol) was allowed to react with hydrazine hydrate (10.1 g, 201 mmol) by refluxing in EtOH for 3 h, then cooling. After evaporation of the solvent, the remaining mixture was refluxed with Ac2O (5 mL) for 4 h. The reaction mixture was then cooled and was diluted with water (30 mL) and stirred for 30 min. The precipitate was collected, dried and recrystalized from THF to yield compound 9 as yellow crystals; mp ≥250 ºC; 1H-NMR: (500 MHz, CDCl3): δ = 9.32 (dd, J = 9.7, 16.2 Hz, 1H), 7.94 (dd, J = 4.2, 8.8 Hz, 2H), 7.86 (d, J = 9.0 Hz, 1H), 7.81–7.70 (m, 2H), 2.77 (s, 3H), 2.64 (s, 3H), 2.64 (s, 3H); 13C NMR: (175 MHz, CDCl3): δ = 169.63, 167.42, 166.98, 166.82, 150.52, 149.42, 149.13, 139.75, 134.18, 130.42, 129.33, 127.98, 127.92, 127.60, 125.35, 118.25, 117.91, 113.19, 108.85, 23.45, 20.81, 20.61; HR-MS (TOFEI): calcd for C20H13N3O4S (391.0627); found (391.0638) (-COCH3).
Diethyl [4-(2-ethoxy-2-oxoethyl)-3-(naphthalen-1-yl)-1,3-thiazol-2(3H)-ylidene]-propanedioate (10). To a stirred solution of compound 1a (0.367 g, 1 mmol) in THF (50 mL) was added chloroethylacetoacetate (0.164 g, 1 mmol) dropwise at 0 ºC and stirring was continued for an extra 12 h at room temperature. The solvent was evaporated, and the resulting oil was extracted with CHCl3 and dried over Na2SO4. The organic layer was evaporated by rotary evaporator to give yellow oil. The obtained oil was heated at 170–180 ºC in an oil bath under vacuo for 10 min. The resulting oil was solidified then washed with ether affording compound 10 as yellow crystals; mp 140–142 ºC; 1H-NMR: (500 MHz, CDCl3): δ = 7.95 (dt, J = 1.14, 1.14, 8.02 Hz, 1H), 7.92–7.89 (m, 1H), 7.55 (m, 2H), 7.51 (d, J = 7.85 Hz, 1H), 7.49 (d, J = 1.54 Hz, 1H), 7.43 (m, 1H), 6.59 (d, J = 0.97 Hz, 1H), 3.81 (m, 2H), 3.71–3.60 (m, 2H), 3.38 (m, 2H), 3.12 (dd, J = 0.91, 17.24 Hz, 1H), 2.9 (d, J = 1.05, 17.28 Hz, 1H), 1.02 (t, J = 7.16 Hz, 3H), 0.89 (t, J = 7.15 Hz, 6H); 13C NMR: (175 MHz, CDCl3): δ = 168.11, 166.28, 163.11, 134.33, 134.18, 132.55, 130.63, 129.10, 128.45, 128.13, 127.07, 125.18, 122.44, 106.74, 90.04, 61.30, 60.65, 60.02, 38.17, 34.02, 30.97, 14.57, 13.88, 13.85; HR-MS (TOFEI) calcd for C24H25NO6S (455.1403); found (455.1398).
1H-3-Thia-11c-azaazuleno[1,8,7,6-cdef]phenanthrene-1,6(5H)-dione (11). The appropriate ester (10) (0.455 g, 1 mmol) was added to PPA (2.27 g, 5 parts by weight) preheated to 150 ºC, and the mixture was stirred for 3 h before being cooled to room temperature, H2O was added, the solution was made basic with conc. NH4OH, and extracted several times with CH2Cl2. The combined extracts were dried (MgSO4), filtered and evaporated in vacuo to yield essentially pure brownish black crystals; mp 200–202 ºC; 1H-NMR: (500 MHz, CDCl3): δ = 8.80 (d, J = 8.7 Hz, 1H), 8.28 (dd, J = 6.9, 5.4 Hz, 2H), 8.09 (d, J = 8.7 Hz, 1H), 7.79–7.72 (m, 1H), 6.93 (s, 1H), 6.79 (s, 1H), 4.09 (d, J = 14.8Hz, 2H). 13C NMR: (175 MHz, CDCl3): δ = 172.68, 157.01, 136.38, 135.96, 135.76, 133.01, 132.18, 130.48, 128.39, 127.47, 126.61, 124.86, 122.53, 115.61, 106.12, 105.63, 44.80; HR-MS (TOFEI) calcd for C17H9NO2S (291.0354); found (291.0349).
ACKNOWLEDGMENT
The authors would like to extend their gratitude to Dr. Louise Dawe from CREAIT labs at Memorial
University for X-ray analyses, and Dr. Jules Dore from Biomedical Sciences Division at the Faculty of Medicine, Memorial University for assisting in bioassays.
References
1. G. Y. Lesher, E. J. Froelich, M. D. Gruett, J. H. Bailey, and R. P. Brundage, J. Med. Pharm. Chem., 1962, 91, 1063. CrossRef
2. C. Mugnaini, S. Pasquini, and F. Corelli, Curr. Med. Chem., 2009, 16, 1746. CrossRef
3. S. K. Srivastava, A. Jha, S. K. Agarwal, R. Mukherjee, and A. C. Burman, Anti-cancer Agents Med. Chem., 2007, 7, 685. CrossRef
4. J. Hosomi, Y. Asahina, and S. Suzue, 1989, WO8912055 (Chem. Abstr., 1990, 113, 6328m).
5. C. Sissi and M. Palumbo, Curr. Med. Chem. Anti-Cancer Agents, 2003, 3, 439. CrossRef
6. a) Zohrea Amoozgar and Mohsen Daneshtalab (2007) Regioselective N1-Alkylation of 4-quinoline-3-carboxylic acid esters using modified Mitsnobu reaction. 21st International Congress For Heterocyclic Chemistry (ICHC-21), July 15-20, 2007, Sydney, Australia, Abstract no. TP-21; b) R. Garlapati and M. Daneshtalab. Design, Synthesis and Biological Evaluation of Thieno[2,3-b]quinolones as Topoisomerase Poisons. 22nd International Congress for Heterocyclic Chemistry (ICHC-21), August 2-7th. Abstract no. PO-43.
7. K. Chipa, K. Yamamoto, K. Miyamoto, J. Nakano, J. Matsumoto, S. Nakamura, and K. Nakada, (Dainippon Pharmaceutical Co., Ltd., Japan). Jpn. Kokai. Tokkyo Koho 1991, 11pp. CODEN: JKXXAF JP 03223289 A.
8. M. J. Robinson, B. A. Martin, and T. D. Gootz, J. Biol. Chem., 1991, 266, 14585.
9. M. J. Robinson, B. A. Martin, T. D. Gootz, P. R. McGuirk, and N. Osheroff, Antimicrob. Agents Chemother., 1992, 36, 751.
10. S. J. Froelich-Ammon, P. R. McGuirk, T. D. Gootz, and M. R. Jefson, Antimicrob. Agents Chemother., 1993, 37, 646.
11. S. H. Elsea, P. R. McGuirk, T. D. Gootz, M. Moynihan, and N. Osheroff, Antimicrob. Agents Chemother., 1993, 37, 2179.
12. W. E. Kohlbrenner, N. Wideburg, D. Weigl, A. Saldivar, and D. T. Chu, Antimicrob. Agents Chemother., 1992, 36, 81.
13. O. Kennard and W. N. Hunter, Angew. Chem, Int. Ed. Engl., 1991, 30, 1254. CrossRef
14. I. Sánchez, R. Reches, D. H. Caignard, P. Renard, and M. D. Pujol, Eur. J. Med. Chem., 2006, 41, 340. CrossRef
15. M. Shimizu, H. Kikumoto, T. Konakahara, Y. Gama, and I. Shibuya, Heterocycles, 1999, 51, 3005. CrossRef
16. M. Shimizu, A. Takeda, H. Fukazawa, Y. Abe, and I. Shibuya, Heterocycles, 2003, 60, 1855. CrossRef
17. T. Chiyoda, K. Iida, K. Takatori, and M. Kajiwara, Synlett, 2000, 1427. CrossRef
18. J. H. Choi, E. B. Choi, and C. S. Pak, Synlett, 2003, 166. CrossRef
19. B. N. Meyer, N. R. Ferrigni, J. E. Putnam, L. B. Jacobsen, D. E. Nichols, and J. L. McLaughlin, Planta Med., 1982, 45, 31. CrossRef
20. T. Mosmann, J. Immunol. Methods, 1983, 65, 1. CrossRef
21. P. Dua and R. Gude, Cancer Chemother. Pharmacol., 2006, 58, 195. CrossRef
22. Z. Bikadi and E. Hazai, J. Chem. Inf., 2009, 1, 15.
23. T. A. Halgren, J. Comput. Chem., 1996, 17, 490. CrossRef
24. G. M. Morris, D. S. Goodsell, R. S. Halliday, R. Huey, W. E. Hart, R. K. Belew, and A. Olson, J. Comput. Chem., 1998, 19, 1639. CrossRef
25. F. J. Solis and R. J. Wets, Math. Oper. Res., 1981, 6, 19. CrossRef