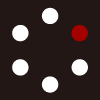
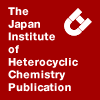
HETEROCYCLES
An International Journal for Reviews and Communications in Heterocyclic ChemistryWeb Edition ISSN: 1881-0942
Published online by The Japan Institute of Heterocyclic Chemistry
e-Journal
Full Text HTML
Received, 11th November, 2011, Accepted, 15th December, 2011, Published online, 22nd December, 2011.
DOI: 10.3987/COM-11-12389
■ Synthesis and Optical Properties of Zincke Salts Having Chiral Anions
Isao Yamaguchi,* Mikihiko Minamitani, and Sachiko Jonai
Department of Material Science, Faculty of Science and Engineering, Shimane University, 1060 Nishikawatsu, Matsue 690-8504, Japan
Abstract
Zincke salts having chiral anions were obtained by the anion exchange reaction of Zincke salts having a chloride anion with R-(-)- or S-(+)-binaphthylphospholic acid sodium salts (R-BINAP-PO4Na and S-BINAP-PO4Na). The anion exchange behavior was investigated by photoluminescence measurements. The circular dichroism (CD) spectra of the Zincke salts having R-BINAP-PO4- or S-BINAP-PO4- showed relatively strong negative and positive Cotton effects, respectively.INTRODUCTION
Zincke salts, a highly electrophilic species formed by reaction between a pyridine derivative and 1-chloro-2,4-dinitrobenzene, are versatile compounds that can afford various N-substituted pyridinium salts as a result of reaction with amines. Pyridinium salts are an important class of compounds that are used as initiators of cationic polymerization,1 cationic surfactants,2 non-linear optical materials,3 and phase transfer catalysts.4 Pyridinium salts containing a chiral N-alkyl group5a have been synthesized through the Zincke reaction and are widely used as starting materials in asymmetric synthesis. However, the synthetic method previously reported for pyridinium salts having a chiral N-alkyl group requires expensive chiral amines. Furthermore, the number of chiral amines is limited. In contrast, many achiral amines exist, and most of them are less expensive than chiral amines. Thus, the reaction of Zincke salts having chiral anions with achiral amines can afford various chiral pyridinium salts at lower cost. We recently reported the synthesis of π-conjugated oligomers and polymers consisting of 5-piperazinium-penta-2,4-dienylideneammonium chloride units as a result of the ring-opening reaction of the pyridinium ring of the Zincke salts with piperazines.6 Additionally, the anion exchange reaction between chloride anion in the polymers and R-(-)- or S-(+)-binaphthylphospholic acid anions yielded helical π-conjugated polymers.7 These results suggest that Zincke salts having chiral anions will be useful starting materials for the synthesis of helical π-conjugated polymers. To the best of our knowledge, however, there has been no report on Zincke salts having chiral anions. An investigation of the structures and optical properties of Zincke salts containing chiral anions will afford fundamental information for the development of new functional chiral materials.
Herein, we report the synthesis and optical properties of Zincke salts produced via anion exchange reactions between N-(2,4-dinitrophenyl)-4-arylpyridinium (aryl = H: 1, phenyl: 2, and 4-pyridyl: 3) or N-(2,4-dinitrophenyl)isoquinolinium (4) chlorides and R-(-)-, S-(+)-, or (±)-binaphthylphospholic acid sodium salts (R-BINAP-PO4Na, S-BINAP-PO4Na, and BINAP-PO4Na).
RESULTS AND DISCUSSION
The reaction of 1, 2, 3, or 4 with R-BINAP-PO4Na or S-BINAP-PO4Na in EtOH caused anion exchange between Cl- and R- or S-BINAP-PO4- to yield Zincke salts containing chiral anions 1R, 1S, 2R, 2S, 3R, 3S, 4R, and 4S in 71%, 90%, 99%, 96%, 82%, 99%, 85%, and 80% yields, respectively (Scheme 1). The reaction of 1, 2, and 3 with BINAP-PO4Na yielded 1rac, 2rac, and 3rac containing a racemic binaphthylphospholic acid anion in 87%, 75%, and 74% yields, respectively (Scheme 1). The results of these reactions are summarized in Table 1. The anion-exchanged Zincke salts were soluble in acetone, EtOH, dimethyl sulfoxide (DMSO), and water, although the original Zincke salts (1-4) were insoluble in acetone and chloroform. The solubility in acetone of the anion exchanged products facilitated their purification.
The NMR and elemental analysis data supported the anion-exchanged structures of 1R-4S. The 1H NMR peak integral between the protons of the pyridinium or isoquinolinium rings and the binaphthyl group suggested that the anion exchange reaction proceeded to completion. The 1H NMR chemical shifts of protons corresponding to R- and S-BINAP-PO4- in 1R-4S were almost the same as those of R- and S-BINAP-PO4Na. However, the 31P NMR spectra of 1R and 1S showed peaks corresponding to phosphate anions at different chemical shifts (δ = 5.02 for 1R and 5.39 for 1S) from that of R- and S-BINAP-PO4Na (δ = 5.87 for R-BINAP-PO4Na and S-BINAP-PO4Na). The reason for the difference between the chemical shifts corresponding to the P atoms in 1R and 1S is unclear. However, the content of water molecules in 1R and 1S may affect the 31P NMR chemical shifts. Elemental analysis and following IR data supported the hydrated structures of the Zincke salts.
The IR spectra of the obtained Zincke salts exhibited strong absorption corresponding to the stretching vibrations of P=O and P-O bonds around 1260 cm-1 and 1100 cm-1, respectively, and the asymmetrical and symmetrical stretching vibrations of the NO2 group at 1543 cm-1 and 1342 cm-1, respectively. The obtained Zincke salts were hygroscopic. Absorption corresponding to hydrated water molecules was observed around 3420 cm-1.
The optical properties of the obtained Zincke salts are summarized in Table 1. Their UV-vis spectra showed absorption corresponding to the R- or S-BINAP-PO4- groups at 216 or 225 nm, respectively. These wavelengths are almost the same as those of R- or S-BINAP-PO4Na. In addition to these peaks, the salts containing Ar = H, Ph, or Py substituents showed absorption peaks corresponding to the pyridinium component at 260, 311, and 274 nm, respectively. Figure 1 shows the UV-vis spectra of 1R, 2R, and 3R in MeOH. The appearance of absorption features corresponding to the pyridinium components of 2R, 2S, 2rac, 3R, 3S, and 3rac at longer wavelengths than those of 1R, 1S, and 1rac is due to the presence of phenyl and pyridyl substituents in 2R, 2S, 2rac, 3R, 3S, and 3rac. The fact that the wavelengths at which absorption corresponding to the pyridinium component of 2R, 2S, and 2rac was observed were longer than those of 3R, 3S, and 3rac is apparently due to intramolecular charge transfer between the phenyl and pyridinium rings in 2R, 2S, and 2rac.
R- and S-BINAP-PO4Na were photoluminescent in solution, whereas the anion-exchanged Zincke salts showed no photoluminescence (PL) in solution. To compare the quenching effect of 1 on BINAP-PO4Na and BINAP-PO4H, PL measurements of the methanol solutions of BINAP-PO4Na or BINAP-PO4H were conducted at a series of 1/methanol concentrations. As shown in Figure 1, the PL intensities of BINAP-PO4Na/methanol solutions decreased with increasing 1 content. In contrast, the PL intensities of the BINAP-PO4H/methanol solutions were almost unchanged regardless of the amount of 1 used. These results suggest that the quenching effect of 1 on BINAP-PO4Na is pronounced when BINAP-PO4- was replaced with Cl- from 1. A quantitative measurement of the PL quenching can be achieved by determining the Stern-Volmer constant, KSV:
I0/I = 1 + KSV[quencher],
where I0 is the intensity of the PL in the absence of the quencher and I is the intensity of the PL in the presence of the quencher. The equation reveals that I0/I increases in direct proportion to the concentration of the quenching moiety, and the constant KSV defines the efficiency of quenching. Stern-Volmer plots for PL quenching by 1 for BINAP-PO4Na are insetted in Figure 2. The KSV value of BINAP-PO4Na was 8.8 × 104 M-1. The result that the KSV value for the 1/BINAP-PO4Na quenching system is considerably greater than that for pyridinium chloride/anthracene quenching system (KSV = 42 M-1)8 corresponds to the fact that the static interaction between N-(2,4-dinitrophenyl)-4-pyridinium cation and BINAP-PO4- facilitates the formation of the quencher/fluorophore adduct. The KSV values for the 2/BINAP-PO4Na and 3/BINAP-PO4Na quenching system was 6.2 × 104 M-1 and 3.5 × 104 M-1, respectively.
The CD spectra of R-BINAP-PO4Na and S-BINAP-PO4Na showed complicated signals in the range of 170-200 nm in methanol. In contrast, the CD spectra of methanol solutions of 1R, 2R, 3R, and 4R as well as 1S, 2S, 3S, and 4S showed relatively strong negative and positive Cotton effects in the range of 205-240 nm, respectively, with a zero-crossing centered at approximately 220 nm, as shown in Figure 3. This wavelength is largely consistent with the λmax positions of R-BINAP-PO4Na and S-BINAP-PO4Na. The difference in the CD signals between R- and S-BINAP-PO4Na and the anion-exchanged Zincke salts is attributed to the difference in the cationic species in these compounds. The peak positions of CD signals of the obtained Zincke salts are summarized in Table 1. In contrast, 1rac, 2rac, and 3rac showed no CD signal.
EXPERIMENTAL
1, 2, 3, and 4 were prepared according to the literatures.9,10 Other reagents were purchased and used without further purification. Solvents were dried, distilled, and stored under N2. Reactions were carried out with standard Schlenk technique under nitrogen.
IR and NMR spectra were recorded on a JASCO FT/IR-660 PLUS spectrophotometer and JEOL AL-400 and ECX-500 spectrometers, respectively. 13C NMR measurements were carried out with gated decoupling technique. Elemental analysis was carried out on a Yanagimoto MT-5 CHN corder. UV-vis and CD spectra were obtained with a JASCO V-560 spectrometer and a JASCO J-720WS, respectively.
Synthesis of 2R. 2 (0.53 g, 1.0 mmol) and R-BINAP-PO4Na (0.37 g, 1.5 mmol) were dissolved in 50 mL of EtOH. After the solution was stirred for 24 h at room temperature, NaCl precipitated from the reaction solution was removed by filtration. The solvent was evaporated under vacuum and the resulting solid was extracted with acetone (150 mL). The solvent was removed under vacuum and resulting solid was dissolved in MeOH (4 mL). The solution was poured in Et2O (400 mL) to give a precipitate, which was collected by filtration and dried under vacuum to obtain 2R as a yellow powder (0.67 g, 99%).
Other Zincke salts were synthesized in an analogous manner.
1R: 1H NMR (400 MHz, DMSO-d6) δ 9.38 (d, J = 5.6 Hz, 2H), 9.12 (d, J = 2.4 Hz, 1H), 8.92-8.98 (m, 2H), 8.40-8.44 (m, 3H), 8.03 (t, J = 8.4 Hz, 4H), 7.44 (d, J = 6.8 Hz, 2H), 7.40 (d, J = 9.2 Hz, 2H), 7.30 (t, J = 8.0 Hz, 2H), 7.21 (d, J = 8.4 Hz, 2H). 13C NMR (100 MHz, DMSO-d6) δ 150.0, 149.2, 149.1, 148.8, 146.1, 143.0, 138.7, 131.9, 131.93, 131.85, 130.3, 130.2, 129.7, 128.4, 128.0, 126.1, 126.0, 124.4, 122.57, 122.55, 121.67, 121.66, 121.1 31P NMR (203 MHz, DMSO-d6) δ 5.02. IR (KBr, cm-1) 3420, 3116, 3072, 1614, 1543, 1343, 1242, 1100, 960, 836, 754. Anal. Calcd for C31H20N3O8P•0.25H2O: C, 62.26; H, 3.46; N, 7.03. Found: C, 62.29; H, 3.89; N, 6.63.
2R: 1H NMR (400 MHz, DMSO-d6) δ 9.40 (d, J = 6.8 Hz, 2H), 9.14 (d, J = 2.4 Hz, 1H), 8.97 (dd, J = 8.8, 2.0 Hz, 1H), 8.82 (d, J = 6.4 Hz, 2H), 8.43 (d, J = 8.4 Hz, 2H), 8.25 (d, J = 7.2 Hz, 2H), 8.02 (t, J = 8.4 Hz, 2H), 7.70-7.76 (m, 3H), 7.38-7.44 (m, 4H), 7.29 (t, J = 8.0 Hz, 2H), 7.20 (d, J = 8.4 Hz, 2H). 13C NMR (100 MHz, DMSO-d6) δ 157.3, 150.0, 149.9, 149.0, 145.9, 143.2, 138.5, 133.1, 133.0, 132.0, 131.9, 130.3, 130.2, 130.0, 129.7, 128.7, 128.4, 126.1, 126.0, 124.4, 124.1, 122.6, 121.7, 121.5. 31P NMR (203 MHz, DMSO-d6) δ 5.76. IR (KBr, cm-1) 3442, 3116, 3058, 1636, 1610, 1541, 1342, 1260, 1243, 1099, 960, 837. Anal. Calcd for C37H24N3O8P•0.3H2O: C, 65.84; H, 3.67; N, 6.23. Found: C, 65.80; H, 3.84; N, 6.47.
3R: 1H NMR (400 MHz, DMSO-d6) δ 9.55 (d, J = 6.4 Hz, 2H), 9.15 (d, J = 2.0 Hz, 1H), 9.00 (dd, J = 8.4, 2.8 Hz, 1H), 8.91-8.94 (m, 4H), 8.44 (d, J = 8.4 Hz, 2H), 8.18 (d, J = 5.2 Hz, 2H), 8.06 (t, J = 8.4 Hz, 2H), 7.45 (t, J = 7.6 Hz, 2H), 7.43 (d, J = 8.8 Hz, 2H), 7.31 (t, J = 8.0 Hz, 2H), 7.20 (d, J = 8.4 Hz, 2H). 13C NMR (100 MHz, DMSO-d6) δ 155.1, 151.2, 149.9, 149.8, 149.2, 146.6, 143.1, 140.4, 138.4, 131.99, 131.92, 130.4, 130.2, 129.8, 128.4, 126.1, 126.0, 125.2, 124.5, 122.53, 122.51, 122.2, 121.66, 121.64, 121.5. 31P NMR (203 MHz, DMSO-d6) δ 5.44. IR (KBr, cm-1) 3447, 3114, 3066, 1637, 1610, 1543, 1342, 1260, 1242, 1100, 961, 837, 817. Anal. Calcd for C36H23N4O8P•0.6H2O: C, 63.46; H, 3.58; N, 8.22. Found: C, 63.50; H, 3.54; N, 7.66.
4R: 1H NMR (400 MHz, DMSO-d6) δ 10.47 (s, 1H), 9.15 (d, J = 2.4 Hz, 1H), 9.05 (d, J = 6.8 Hz, 1H), 8.99 (dd, J = 8.6, 1.8 Hz, 1H), 8.79 (d, J = 6.8 Hz, 1H), 8.59 (d, J = 8.4 Hz, 1H), 8.48 (d, J = 8.8 Hz, 2H), 8.42 (t, J = 8.0 Hz, 1H), 8.16 (t, J = 8.0 Hz, 1H), 8.03 (d, J = 8.8 Hz, 4H), 7.44 (d, J = 7.2 Hz, 2H), 7.41 (d, J = 8.4 Hz, 2H), 7.30 (t, J = 8.0 Hz, 2H), 7.21 (d, J = 8.4 Hz, 2H). 31P NMR (203 MHz, DMSO-d6) δ 5.73. Anal. IR (KBr, cm-1) 3445, 3114, 3066, 1637, 1612, 1591, 1580, 1543, 1345, 1260, 1242, 1100, 961, 840, 817. Calcd for C35H22N4O8P•0.2H2O: C, 64.96; H, 3.49; N, 6.49. Found: C, 64.90; H, 3.53; N, 6.80.
1S: 1H NMR (400 MHz, DMSO-d6) δ 9.38 (d, J = 5.6 Hz, 2H), 9.12 (d, J = 2.4 Hz, 1H), 8.92-8.98 (m, 2H), 8.40-8.44 (m, 3H), 8.03 (t, J = 8.8 Hz, 4H), 7.45 (d, J = 6.8 Hz, 2H), 7.41 (d, J = 8.4 Hz, 2H), 7.30 (t, J = 7.6 Hz, 2H), 7.21 (d, J = 8.0 Hz, 2H). 31P NMR (203 MHz, DMSO-d6) δ 5.39. Anal. Calcd for C31H20N3O8P•0.4H2O: C, 61.98; H, 3.49; N, 7.00. Found: C, 61.98; H, 3.61; N, 6.62.
2S: 1H NMR (400 MHz, DMSO-d6) δ 9.40 (d, J = 7.2 Hz, 2H), 9.14 (d, J = 2.4 Hz, 1H), 8.98 (dd, J = 8.8, 2.4 Hz, 1H), 8.82 (d, J = 7.2 Hz, 2H), 8.43 (d, J = 8.4 Hz, 2H), 8.25 (d, J = 6.8 Hz, 2H), 8.01 (t, J = 8.8 Hz, 2H), 7.70-7.77 (m, 3H), 7.42 (t, J = 7.2 Hz, 2H), 7.37 (d, J = 8.8 Hz, 2H), 7.28 (t, J = 7.2 Hz, 2H), 7.20 (d, J = 8.4 Hz, 2H). 31P NMR (203 MHz, DMSO-d6) δ 5.81. Anal. Calcd for C37H24N3O8P•0.3H2O: C, 65.84; H, 3.67; N, 6.23. Found: C, 65.88; H, 3.81; N, 6.59.
3S: 1H NMR (400 MHz, DMSO-d6) δ 9.55 (d, J = 6.4 Hz, 2H), 9.15 (d, J = 2.4 Hz, 1H), 8.98 (dd, J = 8.8, 2.8 Hz, 1H), 8.92-8.94 (m, 4H), 8.43 (d, J = 8.4 Hz, 2H), 8.18 (d, J = 6.4 Hz, 2H), 8.01 (t, J = 8.4 Hz, 2H), 7.42 (t, J = 7.2 Hz, 2H), 7.38 (d, J = 8.8 Hz, 2H), 7.29 (t, J = 8.0 Hz, 2H), 7.20 (d, J = 8.4 Hz, 2H). 31P NMR (203 MHz, DMSO-d6) δ 5.65. Anal. Calcd for C36H23N4O8P•0.6H2O: C, 63.46; H, 3.58; N, 8.22. Found: C, 63.42; H, 3.58; N, 8.20.
4S: 1H NMR (400 MHz, DMSO-d6) δ 10.47 (s, 1H), 9.15 (d, J = 2.4 Hz, 1H), 9.05 (d, J = 6.8 Hz, 1H), 8.99 (dd, J = 8.6, 1.8 Hz, 1H), 8.79 (d, J = 6.8 Hz, 1H), 8.59 (d, J = 8.4 Hz, 1H), 8.48 (d, J = 8.8 Hz, 2H), 8.42 (t, J = 8.0 Hz, 1H), 8.16 (t, J = 8.0 Hz, 1H), 8.03 (d, J = 8.8 Hz, 4H), 7.44 (d, J = 7.2 Hz, 2H), 7.41 (d, J = 8.4 Hz, 2H), 7.30 (t, J = 8.0 Hz, 2H), 7.21 (d, J = 8.4 Hz, 2H). Anal. Calcd for C35H22N4O8P•0.2H2O: C, 64.96; H, 3.49; N, 6.49. Found: C, 64.88; H, 3.59; N, 6.67.
1rac: 1H NMR (400 MHz, DMSO-d6) δ 9.38 (d, J = 6.4 Hz, 2H), 9.12 (d, J = 2.8 Hz, 1H), 8.91-8.97 (m, 2H), 8.40-8.44 (m, 3H), 8.02 (t, J = 8.4 Hz, 4H), 7.44 (d, J = 7.6 Hz, 2H), 7.39 (d, J = 8.8 Hz, 2H), 7.29 (t, J = 8.0 Hz, 2H), 7.21 (d, J = 8.4 Hz, 2H). 13C NMR (100 MHz, DMSO-d6) δ 150.0, 149.9, 149.1, 148.8, 146.1, 143.0, 138.7, 131.9, 131.8, 130.3, 130.2, 129.7, 128.4, 128.0, 126.1, 126.0, 124.4, 122.5, 121.7, 121.4. 31P NMR (203 MHz, DMSO-d6) δ 5.49. Anal. Calcd for C31H20N3O8P•0.2H2O: C, 62.36; H, 3.44; N, 7.04. Found: C, 62.35; H, 3.92; N, 6.75.
2rac: 1H NMR (400 MHz, DMSO-d6) δ 9.41 (d, J = 6.8 Hz, 2H), 9.14 (d, J = 2.4 Hz, 1H), 8.99 (dd, J = 8.8, 2.4 Hz, 1H), 8.83 (d, J = 5.6 Hz, 2H), 8.42 (d, J = 8.4 Hz, 2H), 8.25 (d, J = 7.2 Hz, 2H), 8.00 (t, J = 8.8 Hz, 2H), 7.70-7.76 (m, 3H), 7.42 (t, J = 8.0 Hz, 2H), 7.38 (d, J = 8.8 Hz, 2H), 7.28 (t, J = 8.0 Hz, 2H), 7.20 (d, J = 8.4 Hz, 2H). 31P NMR (203 MHz, DMSO-d6) δ 5.87. Anal. Calcd for C37H24N3O8P•0.2H2O: C, 62.36; H, 3.44; N, 7.04. Found: C, 65.96; H, 3.61; N, 6.32.
3rac: 1H NMR (400 MHz, DMSO-d6) δ 9.54 (d, J = 6.4 Hz, 2H), 9.15 (d, J = 2.0 Hz, 1H), 8.99 (dd, J = 8.8, 2.4 Hz, 1H), 8.92-8.95 (m, 4H), 8.43 (d, J = 8.8 Hz, 2H), 8.17 (d, J = 6.4 Hz, 2H), 8.03 (t, J = 9.2 Hz, 2H), 7.45 (d, J = 6.8 Hz, 2H), 7.41 (d, J = 8.8 Hz, 2H), 7.30 (t, J = 8.0 Hz, 2H), 7.20 (d, J = 8.4 Hz, 2H). Anal. Calcd for C36H23N4O8P•0.6H2O: C, 63.46; H, 3.58; N, 8.22. Found: C, 63.55; H, 3.62; N, 7.59.
ACKNOWLEDGEMENT
This work was supported by Grant-in-Aid for Scientific Research (C) from Ministry of Education, Science, Culture, and Sports Japan (No. 21550173).
References
1. (a) E. Takahashi, F. Sanda, and T. Endo, J. Polym. Sci., Part A: Polym. Chem., 2002, 40, 1037; CrossRef (b) M. Onciu, A. Onen, and Y. Yagci, Polym. Int., 2001, 50, 144; CrossRef (c) W. Schnabel, Macromol. Rapid Commun., 2000, 628. CrossRef
2. (a) N. O. Mchedlov-Petrossyan, N. A. Vodolazkaya, A. A. Kornienko, E. L. Karyakina, and C. Reichardt, Langmuir, 2005, 21, 7090; CrossRef (b) J. Pernak, J. Kalewska, H. Ksycińska, and J. Cybulski, Eur. J. Med. Chem., 2001, 36, 899. CrossRef
3. (a) H. Umezawa, S. Okada, H. Oikawa, H. Matsuda, and H. Nakanishi, Bull. Chem. Soc. Jpn., 2005, 78, 344; CrossRef (b) B. J. Coe, J. A. Harris, I. Asselberghs, K. Wostyn, K. Clays, A. Persoons, B. S. Brunschwig, S. J. Coles, T. Gelbrich, M. E. Light, M. B. Hursthouse, and K. Nakatani, Adv. Funct. Mater., 2002, 12, 110. CrossRef
4. (a) D. Wang, M. Wang, X. Wang, R. Zhang, J. Ma, and L. Sun, J. Mol. Cat. A: Chem., 2007, 270, 278; CrossRef (b) R. T. Dere, R. R. Pal, P. S. Patil, and M. M. Salunkhe, Tetrahedron Lett., 2003, 44, 5351. CrossRef
5. (a) G. H. R. Viana, I. C. Santos, R. B. Alves, L. Gil, C. Marazano, and R. P. F. Gil, Tetrahedron Lett., 2005, 46, 7773; CrossRef (b) M. Eda, M. J. Kurth, and M. H. Nantz, J. Org. Chem., 2000, 65, 5131; CrossRef (c) D. Urban, E. Duval, and Y. Langlois, Tetrahedron Lett., 2000, 41, 9251; CrossRef (d) D. Gnecco, J. Juárez, A. Galindo, C. Marazano, and R. G. Enriíquez, Synth. Commun., 1999, 29, 281; CrossRef (e) J. Levillain, D. Paquer, A. Sene, and M. Vazeux, Synthesis, 1998, 99; CrossRef (f) S. Kunugi, T. Okubo, and N. Ise, J. Am. Chem. Soc., 1976, 98, 2282; CrossRef (g) E. N. Marvell, G. Caple, and I. Shahidi, J. Am. Chem. Soc., 1970, 92, 5641; CrossRef (h) E. N. Marvell and I. Shahidi, J. Am. Chem. Soc., 1970, 92, 5646. CrossRef
6. (a) I. Yamaguchi, Y. Matsuda, and M. Sato, Polym. Int., 2011, 60, 78; CrossRef (b) I. Yamaguchi, A. Kado, T. Fukuda, H. Fukumoto, T. Yamamoto, and M. Sato, Eur. Polym. J., 2010, 46, 1119; CrossRef (c) I. Yamaguchi, S. Shingai, and M. Sato, Macromolecules, 2008, 41, 6292. CrossRef
7. I. Yamaguchi, S. Jonai, and Y. Matsuda, Macromolecules, 2011, 44, 1273. CrossRef
8. H. J. Pownall and L. C. Smith, Biochemistry, 1974, 13, 2594. CrossRef
9. (a) T. H. Zincke, G. Heuser, and W. I. U. Möller, Justus Liebigs Ann Chem., 1904, 333, 296; CrossRef (b) I. Yamaguchi, H. Higashi, S. Shigesue, S. Shingai, and M. Sato, Tetrahedron Lett., 2007, 48, 7778. CrossRef
10. D. Barbier, C. Marazano, B. C. Das, and P. Potier, J. Org. Chem., 1996, 61, 9596 CrossRef