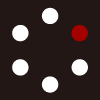
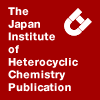
HETEROCYCLES
An International Journal for Reviews and Communications in Heterocyclic ChemistryWeb Edition ISSN: 1881-0942
Published online by The Japan Institute of Heterocyclic Chemistry
e-Journal
Full Text HTML
Received, 17th November, 2011, Accepted, 15th December, 2011, Published online, 22nd December, 2011.
DOI: 10.3987/COM-11-12392
■ Hydrolysable Tannins Isolated from Syzygium aromaticum: Structure of a New C-Glucosidic Ellagitannin and Spectral Features of Tannins with a Tergalloyl Group
Li-Ming Bao, _ Eerdunbayaer, Akiko Nozaki, Eizo Takahashi, Keinosuke Okamoto, Hideyuki Ito, and Tsutomu Hatano*
Graduate School of Medicine, Dentistry and Pharmaceutical Sciences, Okayama University, 1-1-1 Tsushima-naka, Okayama 700-8530, Japan
Abstract
Eighteen hydrolysable tannins, including a new C-glucosidic tannin named aromatinin A (1), were isolated from an aqueous acetone extract of dried flower buds of Syzygium aromaticum Merr. et Perry. We determined that 1 had a gallic acid C-glucoside structure, based on the spectral data and synthesis from casuarinin (18) and gallic acid (20). This is a rare example of hydrolysable tannins with gallic acid C-glucoside structure. We also report the 1H nuclear magnetic resonance (NMR) spectral features of syzyginin A (2), bicornin (3), and platycaryanin A (4), which were also isolated from S. aromaticum, based on their structures with a tergalloyl group or its depsidone form. The remaining known compounds were identified as alunusnin A (5), rugosin C (6), 1,2,3-tri-O-galloyl-β-D-glucose (7), 1,2,3,6-tetra-O-galloyl-β-D-glucose (8), tellimagrandin II (9), casuarictin (10), heterophylliin D (11), rugosin D (12), rugosin F (13), euprostin A (14), 1,2-di-O-galloyl-3-O-digalloyl-4,6-O-(S)-hexa- hydroxydiphenoy-β-D-glucose (15), alienanin B (16), squarrosanin A (17), and 18. The antifungal effects of hydrolysable tannins, 9, 12, and 18 against Candida strains are also described.INTRODUCTION
Cloves are aromatic flower buds of Syzygium aromaticum, a woody plant belonging to the family Myrtaceae. Cloves have been used as a spice and as a constituent material for traditional East Asian medicines. Previously, we reported that eugenol, the major constituent of clove essential oil, and related compounds show antifungal effects against Candida species.1 Isolation of tannins with tergalloyl, syzygyl, and valoneoyl groups from S. aromaticum leaves has also been reported.2,3 Our further investigation of the hydrophilic constituents of cloves led to the isolation of 18 tannins, including a new C-glucosidic ellagitannin. This study considers the isolation and structures of those tannins and the spectral features of tannins with a tergalloyl group. Our preliminary study indicated that several tannins showed antifungal effects against Candida species, which are also described.
RESULTS AND DISCUSSION
An aqueous acetone homogenate of dried S. aromaticum flower buds was concentrated and extracted with n-hexane, Et2O, EtOAc, and n-BuOH, successively. Each extract was separated by chromatography using Diaion HP-20, Toyopearl HW-40, Sephadex LH-20, or MCI-gel CHP-20P, followed by preparative reversed phase high performance liquid chromatography (HPLC) to yield tannins 1–18 (Figures 1-4).
Compound 1 was obtained as an off-white amorphous powder. The high-resolution electrospray-ionization mass spectrum (HRESIMS) with negative-ion mode established the molecular formula as C48H32O30. The 1H NMR spectrum of 1 showed glucose proton signals (Table 1) with chemical shifts and coupling constants similar to those of C-glucosidic tannins, such as casuarinin (18) and stachyurin (19).4 The spectrum also showed a two-proton singlet due to a galloyl group at δ 7.21 and four one-proton singlets at δ 7.18, 6.88, 6.544, and 6.539 in the aromatic proton region. Three one-proton singlets at δ 6.88, 6.544, and 6.539 among them accounted for two hexahydroxydiphenoyl (HHDP) groups, one of which participates in the C-glucosidic linkage arising from a phenol-aldehyde coupling.5 These 1H NMR data indicate the existence of two HHDP groups at O-2/O-3 and O-4/O-6 of the glucose core, and suggest that a galloyl group is at O-5 of the glucose core in 1, as observed for 18 and 19. The remaining aromatic proton signal at δ 7.18 was attributed to an additional galloyl group (ring A in formula 1) forming another C-glucosidic linkage. The 13C NMR spectrum of 1 showed glucose carbon signals at δ 41.3 (C-1), 82.6 (C-2), 75.3 (C-3), 73.0 (C-4), 71.4 (C-5), and 64.2 (C-6), whose chemical shifts were similar to those of the corresponding carbon signals in squarrosanin A (17)6 [δ 41.4 (C-1), 82.0 (C-2), 75.1 (C-3), 72.8 (C-4), 71.1 (C-5), and 64.5 (C-6)], indicating the presence of two C-glucosidic linkages at the anomeric carbon. The heteronuclear multiple bond correlation (HMBC) spectrum of 1 showed correlations between glucose H-1 and A-ring C-3, and between glucose H-2 and A-ring C-2 (arrows in Figure 1), indicating that the galloyl group (i.e., A ring) was linked to glucose C-1. The glucose H-2, H-3, H-4, and H-6 signals showed three-bond correlations with the ester carbonyl carbon signals in the HHDP groups (Figure 1), further indicating that the HHDP groups are at O-2/O-3 and O-4/O-6 of the glucose residue. The configuration at glucose C-1 was assigned to be S based on the characteristic singlet of the H-1 signal, as observed for 19 and other C-glucosidic tannins.7 The circular dichroism (CD) spectrum of 1 showed a positive Cotton effect in the short-wavelength region ([θ]235 +1.4 x 105), indicating the S configuration of both HHDP groups.8
The structure 1 assigned to this compound was further confirmed by acid-catalyzed condensation of 18 with gallic acid (20) to afford 1. This compound was new and was named aromatinin A. Although norbergenin (21)9 (Figure 5) was a known example of a C-glucoside of galloyl ester, compound 1 is a rare example of hydrolysable tannins with gallic acid C-glucoside structure.
Compound 2 was obtained as an off-white amorphous powder. HRESIMS of 2 indicated its molecular formula to be C48H34O31. The 1H NMR spectrum exhibited three two-proton singlets at δ 7.10, 7.00, and 6.93, due to the galloyl groups, and three one-proton singlets at δ 6.80, 6.49, and 6.46, attributable to a triphenoyl group in the aromatic region. The coupling pattern of the glucose proton signals (Table 1) was almost identical to that of tellimagrandin II (9),4,10 indicating the presence of a β-glucopyranose core with 4C1 conformation, and that a diphenoyl moiety of the triphenoyl group was at O-4/O-6 of the glucose core. The 13C NMR spectrum of 2 indicated that the triphenoyl group was assignable to a tergalloyl group (see Experimental). The 1H signal pattern for 2 was similar to that for syzyginin A (22) (Figure 2), which was previously isolated from the leaves of this plant.2 However, the proton signal assignable to H-6” (δ 6.80) in 2 shifted upfield relative to the corresponding proton of 22 [H-6” (δ 6.98)]. Furthermore, a carboxyl carbon signal and C-1” of the tergalloyl group in the 13C NMR spectrum of 2 shifted downfield relative to the corresponding signals of 22 [δ 170.6 → 171.5 (C-7”), δ 114.3 → 116.1 (C-1”)]. We assumed that these differences could be due to salt formation of the carboxyl group in the tergalloyl group, and examined the effect of adding deuterium chloride (DCl) to an acetone-d6 solution of 2 on the 1H chemical shifts. The addition caused a downfield shift of the tergalloyl proton H-6” of 2 from δ 6.80 to δ 6.91, accompanied by the formation of a free carboxyl group. Based on these data, compound 2 was assigned to the salt form of 22.
Compound 3 was obtained as an off-white amorphous powder. HRESIMS in the negative-ion mode established the molecular formula as C48H32O30. The 1H NMR spectrum showed three two-proton singlets due to galloyl groups at δ 7.06, 6.97, and 6.95, and three one-proton singlets attributable to a triphenoyl group at δ 6.93, 6.84, and 6.52. The spectrum also showed sugar proton signals (Table 1) in which the coupling constants were of a β-glucopyranose core with a 4C1 conformation. Their chemical shifts were similar to those of tannins in which three galloyl groups are at O-1–O-3, and the diphenoyl part of the triphenoyl group is at O-4/O-6 of the glucose core.10 Although the chemical shifts of the galloyl and glucose protons were closely similar to those of the corresponding protons of 2, those of three one-proton singlets (δ 6.93, 6.84, and 6.52) in the 1H NMR spectrum of 3 were different from those of the corresponding protons (δ 6.80, 6.49, and 6.46) of 2. Comparisons of the 13C NMR spectrum of 3 to that of 2 showed upfield shifts in the following triphenoyl carbon signals: C-ring C-7” (δ 171.5 → 163.5), B-ring C-4’ (δ 149.6 → 148.2), and B-ring C-6’ (δ 150.0 → 145.1), in contrast to the downfield shifts of the carbon signals of B-ring C-1’ (δ 115.9 → 120.8), B-ring C-3’ (δ 107.9 → 112.4), and B-ring C-5’ (δ 136.7 → 142.8). These changes in 13C chemical shifts, coupled with the [M-H]- ion peak at m/z 1087 in the ESIMS, which was 18 mass units lower than that of 2, indicated that the triphenoyl group in 3 formed a depsidone structure at the carbon ortho to the phenoxy moiety on the diphenoyl group. The carbon signal of B-ring C-6’ (δ 145.1) shifted upfield compared to the carbon signal of B-ring C-4’ (δ 148.2), and the neighboring carbons, B-ring C-1’ (δ 120.8) and B-ring C-5’ (δ 142.8), shifted downfield (Δδ +4.9 and +6.1) compared to the corresponding B-ring C-1’ (δ 115.9) and B-ring C-5’ (δ 136.7) signals in 2, respectively. Therefore, the depsidone linkage was assigned at C-6’ rather than at C-4’. In the HMBC spectrum (in CD3OD), the H-3’ signal of the depsidone-forming tergalloyl group at δ 6.90 showed connectivity with the glucose H-6 signal at δ 5.32 via a common tergalloyl carbonyl carbon C-7’ (δ 168.2). Furthermore, the tergalloyl H-3 signal at δ 6.49 was correlated with the glucose H-4 signal at δ 5.30 via the ester carbonyl signal tergalloyl C-7 (δ 169.1). These data agree with the orientation of the
depsidone-forming tergalloyl group in 3, as shown in the formula. The CD spectrum of 3 showed a positive Cotton effect in the short wavelength region ([θ]224 +4.9 × 104), indicating the S configuration of the tergalloyl group. The structure thus assigned for 3 was further supported by the formation of 2 from 3, based on cleavage of the depside linkage. Previously, structure 3 was given the revised structure for bicornin based on spectral comparison.11 The depsidone structure of bicornin was thus substantiated by a successful chemical correlation in the present experiment.
Compound 4 was obtained as an off-white amorphous powder. The negative ESIMS of 4 showed an [M-H]- ion peak at m/z 1103, which corresponded to the molecular formula C48H32O31. The 1H and 13C NMR spectra of 4 were similar to those of glansrin A (23)12 (Figure 5), whereas those of platycaryanin A (24)13 showed some differences in the tergalloyl group chemical shifts. However, adding DCl to an acetone-d6 solution of 4 indicated that 4 is a salt form of 24 (Figure 2), as was
the case with 2 and 22. The orientation of the tergalloyl group in 4 was confirmed to be the same as that in 24 by HMBC correlation with 4. The structural assignment for 4 was further supported by the formation of 4 from alunusnin A (5)13,14 and by cleavage of the depside linkage in 5 (see Experimental). Based on these findings, compound 4 was assigned to a salt in the carboxyl group in the tergalloyl residue in 24.
As shown in Figure 6, respective comparisons of the 1H NMR spectra of 2 and 4 to those of 22 and 24 showed upfield shifts of the tergalloyl group H-6” signals, accompanied by salt formation of the free carboxyl group. As a result, the chemical shifts of the tergalloyl proton signals in 4 were apparently similar to those for 23, although the assignments of the H-3 and H-3’ signals in 23 were interchanged. This may cause confusion when identifying this type of tannin and strongly suggests the necessity for a desaltation procedure to purify tannins. In contrast, the H-3’ signals of the depsidone-forming tergalloyl group (Figure 7) of 3 and 5 showed remarkable downfield shifts relative to the corresponding signals of the tergalloyl group in 2 and 4. Therefore, depsidone formation was indicative of this downfield shift [δ 6.93 (3) and 6.96 (5)] to show the pattern of two singlets (δ 6.84–6.96) and one singlet (δ 6.52–6.59) (for the depsidone form, 3, 5 in Figure 6), from the pattern of one singlet (δ 6.80–7.01) and two singlets (δ 6.46–6.65) (for the carboxyl form, 2, 4, 22, and 24 in Figure 6). Signals that appeared in the lowest chemical shifts were then compared to distinguish the tergalloyl from the valoneoyl group. Signals of the tergalloy protons (H-6”; δ 6.98 in 22 and δ 7.01 in 24) showed upfield shifts relative to the corresponding signals of the valoneoyl protons (H-6”; δ 7.10 in 6 and δ 7.14 in rugosin A15). Analogous differences were also observed in the comparison of the depsidone-forming tergalloyl groups (H-3’; δ 6.93 in 3 and δ 6.96 in 5) and depsidone-forming valoneoyl groups (H-3’; δ 7.20 in praecoxin C16 and δ 7.12 in prostratin C17). These spectral features will facilitate identification of these types of tannins from other sources.
The remaining known compounds were identified as alunusnin A (5), rugosin C (6),15 1,2,3-tri-O-galloyl-β-D-glucose (7),18 1,2,3,6-tetra-O-galloyl-β-D-glucose (8),19 tellimagrandin II (9),4,10 casuarictin (10),4 heterophylliin D (11),20 rugosin D (12),21 rugosin F (13),21 euprostin A (14),17 1,2-di-O-galloyl-3-O-digalloyl-4,6-O-(S)-hexahydroxydiphenoy-β-D-glucose (15),22 alienanin B (16),23,24 squarrosanin A (17),6 and casuarinin (18).4,14
The preliminary results for the antifungal effects of four tannins, on the examination with those obtained from several sources (see Experimental), against three Candida strains are shown in Table 2. Although the antifungal activity of 9, 12, and 18 was lower than that of amphotericin B, used as a positive control, these compounds showed antifungal activities against all strains examined, but 8 had no activity. Further examination to find tannins with more potent effects on Candida species should be conducted.
EXPERIMENTAL
General procedures. HRESIMS was performed using a Bruker MicroTOF-Q spectrophotometer in negative ion mode. ESIMS was performed using an API 4000 liquid chromatography-mass spectroscopy system in negative ion mode. CD spectra were measured on a JASCO J-720 W spectrophotometer. NMR experiments were performed using a Varian INOVA AS 600 instrument (600 MHz for 1H NMR and 151 MHz for 13C NMR). The solvent was acetone-d6 and D2O (9:1, v/v), unless mentioned otherwise. Chemical shifts are given in δ (ppm) values relative to those of the solvent signal [acetone-d6 (δH 2.04; δC 29.8), CD3OD (δH 3.30; δC 49.0)] on the tetramethylsilane scale. Column chromatography was conducted on Toyopearl HW-40 (coarse grade; Tosoh, Tokyo, Japan), Sephadex LH-20 (Pharmacia Fine Chemicals, Upsala, Sweden), Diaion HP-20 (Mitsubishi Chemicals, Tokyo, Japan), and MCI-gel CHP-20P (Mitsubishi Chemicals, Tokyo, Japan). Normal phase HPLC was conducted on a YMC-Pack SIL A-003 column (4.6 i.d. × 250 mm) developed with n-hexane-MeOH-tetrahydrofuran-formic acid (60:45:15:1) containing oxalic acid (500 mg/1.2 L; flow rate, 1.5 mL/min; 280 nm UV detection) at room temperature. Analytical reversed phase HPLC was performed on a YMC-Pack ODS A-302 column (4.6 i.d. × 150 mm) with 0.01 M H3PO4-0.01 M KH2PO4-CH3CN (42.5:42.5:15; flow rate, 1.0 mL/min; 280 nm UV detection) at 40 ºC. Preparative reversed phase HPLC was performed on a YMC-Pack ODS A-324 column (10 i.d. × 300 mm) developed with 0.01 M H3PO4-0.01 M KH2PO4-CH3CN (2:2:1; flow rate, 2.0 mL/min; 280 nm UV detection) at 40 ºC.
Plant material. Dried S. aromaticum flower buds were purchased from Tochimoto-tenkai-do (Osaka, Japan). The specimen was kept at the Medicinal Botanical Garden of Okayama University (No. 007605001).
Extraction and isolation. Plant material (68 g) was homogenized in H2O-acetone (1:9, v/v, 3 L) and filtered. The filtrate was then concentrated to 300 mL and extracted with n-hexane (300 mL × 3), Et2O (300 mL × 3), EtOAc (300 mL × 3), and n-BuOH (300 mL × 3), successively, to furnish the n-hexane (10.6 g), Et2O (1.37 g), EtOAc (3.54 g), and n-BuOH (3.56 g) extracts, and a water-soluble portion (2.27 g). The EtOAc extract was subjected to chromatography on Toyopearl HW-40 (2.2 i.d. × 40 cm) (TP1) with 70% EtOH → 70% acetone. The eluates were fractionated by monitoring either normal or reversed phase HPLC. Fr. 26–27 (41.6 mg) from TP1 was further separated on MCI-gel CHP-20P with H2O → aq. MeOH → 70% acetone, to yield 1,2,3-tri-O-galloyl-β-D-glucose (7) (6.4 mg) and 1,2,3,6-tetra-O- galloyl-β-D-glucose (8) (6.6 mg). Fr. 87–100 (132.6 mg) from TP1 was applied to a Sep-Pak C18 cartridge with H2O → aq. MeOH (10% → 20% → 30% → 40%, v/v) → 100% MeOH to give tellimagrandin II (9) (49.2 mg). Fr. 121–165 (185.9 mg) from TP1 was subjected to column chromatography on MCI-gel CHP-20P with H2O → aq. MeOH (20% → 40% → 60%, v/v) → 100% MeOH → 70% acetone, to give syzyginin A (2) (9.6 mg) and casuarictin (10) (15.2 mg). Fr. 183–195 (23.2 mg) from TP1 was further subjected to a Sep-Pak C18 cartridge followed by preparative HPLC to yield platycaryanin A (4) (7.9 mg). In a separate experiment, another batch of cloves (500 g) was homogenized in H2O-acetone (1:9, v/v, 15 L), and the filtered homogenate was concentrated in vacuo to 1.5 L and extracted with n-hexane (1.5 L × 3), Et2O (1.5 L × 3), EtOAc (1.5 L × 3), and n-BuOH (1.5 L × 3), successively, to yield the n-hexane (93.2 g), Et2O (15.1 g), EtOAc (25.7 g), and n-BuOH (66.0 g) extracts, and a water-soluble portion (23.9 g). A part (10 g) of the EtOAc extract was subjected to Toyopearl HW-40 (2.2 i.d. × 60 cm) (TP2) with 70% EtOH → 70% acetone. Fr. 165–210 (276.2 mg) from TP2 was further subjected to MCI-gel CHP-20P (MC1) using H2O → aq. MeOH (20% → 40% → 60%, v/v) → 100% MeOH → 70% acetone. The Fr. 20% MeOH eluate from MC1 afforded casuarinin (18) (19.7 mg). The Fr. 40% MeOH eluate from MC1 was further subjected to column chromatography over MCI-gel CHP-20P with H2O → aq. MeOH (20% → 40% → 60%, v/v) →100% MeOH → 70% acetone, followed by preparative HPLC to yield 2 (9.8 mg) and rugosin C (6) (5.8 mg). Fr. 70% acetone (306.6 mg) from TP2 was separated on Toyopearl HW-40 (2.2 i.d. × 40 cm) [with 70% EtOH → 70% EtOH-70% acetone (9:1 → 8:2 → 7:3, v/v) → 70% acetone], MCI-gel CHP-20P [with H2O → aq. MeOH (20% → 40% → 60%, v/v) → 100% MeOH → 70% acetone], Sephadex LH-20 (with 70% EtOH-70% acetone), successively, and further purification of the fractions by preparative HPLC gave bicornin (3) (2.2 mg), alunusnin A (5) (4.4 mg), heterophylliin D (11) (2.7 mg), rugosin D (12) (9.5 mg), and rugosin F (13) (15.8 mg). The n-BuOH extract (66.0 g) was subjected to Diaion HP-20 (5.5 i.d. × 25 cm) chromatography with H2O → aq. MeOH (20% → 40% → 60%, v/v) → 100% MeOH → 70% acetone as eluants. The 40% MeOH eluate was applied to column chromatography over Toyopearl HW-40 (2.2 i.d. × 60 cm) (with 70% EtOH → 70% acetone), MCI-gel CHP-20P (with aq. MeOH → 70% acetone), Sephadex LH-20 (with 70% EtOH-70% acetone), and further purification of the fractions by preparative HPLC gave 6 (8.7 mg), euprostin A (14) (2.6 mg), 1,2-di-O-galloyl-3-O-digalloyl-4,6-O-(S)-hexahydroxydiphenoy-β-D-glucose (15) (2.6 mg), and squarrosanin A (17) (1.6 mg). The 60% MeOH eluate (5.89 g) was purified by column chromatography on Toyopearl HW-40 (2.2 i.d. × 60 cm) with 70% EtOH → 70% acetone and a Sep-Pak C18 cartridge with H2O → aq. MeOH (20% → 40% → 60%, v/v) → 70% acetone, to give 13 (3.8 mg). The water-soluble portion (23.9 g) was applied to Diaion HP-20 (5.5 i.d. × 25 cm) chromatography with H2O → aq. MeOH (20% → 40% → 60%, v/v) → 100% MeOH → 70% acetone as eluants. The 40% MeOH eluate (5.92 g) was further subjected to Toyopearl HW-40 (2.2 i.d. × 60 cm) with 70% EtOH → 70% acetone. Repeated chromatography of the 70% acetone fraction (249.3 mg) on Toyopearl HW-40 with 70% EtOH → 70% EtOH-70% acetone (9:1 → 8:2 → 7:3, v/v) → 70% acetone, followed by preparative HPLC of the fractions gave aromatinin A (1) (4.6 mg) and alienanin B (16) (2.6 mg). An additional 2 kg of cloves was also treated in an analogous way to afford 1 (9.8 mg), 3 (8.6 mg), and 5 (10.4 mg).
Aromatinin A (1): An off-white amorphous powder, [α]D +92.0o (c 1.0, MeOH). ESIMS m/z: 1087 [M-H]-. HRESIMS m/z: 1087.0866 [M-H]- (calcd. for C48H32O30 - H, 1087.0906). CD [θ] (nm, in MeOH): [θ]235 +1.4 × 105, [θ]262 -2.9 × 104, [θ]281 +2.4 × 104. 1H NMR δ: 7.21 (2H, s), 7.18 (1H, s), 6.88 (1H, s), 6.544 (1H, s), 6.539 (1H, s), glucose protons (Table 1). 1H NMR (CD3OD) δ: 7.15 (2H, s), 7.04 (1H, s), 6.81 (1H, s), 6.48 (1H, s), 6.40 (1H, s), 5.70 (1H, d, J = 8 Hz, Glc H-4), 5.26 (1H, dd, J = 3.5, 8 Hz, Glc H-5), 5.22 (1H, br s, Glc H-1), 5.08 (1H, s, Glc H-3), 4.94 (1H, s, Glc H-2), 4.90 (1H, dd, J = 3.5, 13.5 Hz, Glc H-6), 4.09 (1H, d, J = 13.5 Hz, Glc H-6). 13C NMR δ: 41.3 (Glc C-1), 64.2 (Glc C-6), 71.4 (Glc C-5), 73.0 (Glc C-4), 75.3 (Glc C-3), 82.6 (Glc C-2), 105.9, 107.4, 108.8 (HHDP C, E, F-ring C-3), 110.2 (galloyl D-ring C-2, 6), 111.7 (galloyl A-ring C-6), 115.4, 115.5, 116.0, 116.9 (HHDP C-1), 120.5 (galloyl D-ring C-1), 121.8 (galloyl A-ring C-2), 123.0, 123.3, 125.1, 126.6 (HHDP C-2), 127.9 (HHDP B-ring C-3), 135.1, 136.1, 136.4, 137.5 (HHDP C-5), 137.6 (galloyl A-ring C-4), 139.4 (galloyl D-ring C-4), 142.7, 142.8, 143.8, 144.0, 144.2, 144.3, 145.0, 145.2 (HHDP C-4, 6 ), 145.6 (galloyl A-ring C-5), 145.9 (galloyl D-ring C-3, 5), 146.7 (galloyl A-ring C-3), 166.3 (galloyl D-ring C-7), 168.3 (HHDP E-ring C-7), 168.4 (galloyl A-ring C-7), 169.1 (HHDP F-ring C-7), 169.7 (HHDP B-ring C-7), 169.8 (HHDP C-ring C-7). 13C NMR (CD3OD) δ: 42.5 (Glc C-1), 64.9 (Glc C-6), 72.1 (Glc C-5), 74.2 (Glc C-4), 76.6 (Glc C-3), 83.0 (Glc C-2), 105.8, 108.0, 109.3 (HHDP C, E, F-ring C-3), 110.6 (galloyl D-ring C-2, 6), 111.1 (galloyl A-ring C-6), 116.1 (HHDP B-ring C-1), 116.3 (HHDP F-ring C-1), 116.4 (HHDP E-ring C-1), 117.5 (HHDP C-ring C-1), 121.0 (galloyl D-ring C-1), 123.1, 123.8, 125.5, 127.0 (HHDP C-2), 128.2 (HHDP B-ring C-3), 130.8, 136.0, 137.2, 137.5, 137.7 (HHDP C-5), 139.3 (galloyl A-ring C-4), 140.3 (galloyl D-ring C-4), 144.0, 144.3, 144.9 (2C), 144.8, 145.4, 146.0, 146.04 (HHDP C-4, 6), 146.5 (galloyl A-ring C-5), 146.6 (galloyl D-ring C-3, 5), 147.5 (galloyl A-ring C-3), 167.4 (galloyl D-ring C-7), 169.2 (HHDP E-ring C-7), 170.0 (galloyl D-ring C-7), 170.4 (HHDP F-ring C-7), 171.3 (HHDP B-ring C-7, C-ring C-7).
Syzyginin A (2): An off-white amorphous powder, [α]D +10.5o (c 1.0, MeOH). ESIMS m/z: 1105 [M-H]-. HRESIMS m/z: 1105.1027 [M-H]- (calcd. for C48H34O31 - H, 1105.1011). CD [θ] (nm, in MeOH): [θ]239 +5.8 × 104, [θ]261 -1.3 × 104, [θ]286 +1.2 ×104. 1H NMR δ: 7.10, 7.00, 6.93 (each 2H, s), 6.80, 6.49, 6.46 (each 1H, s), glucose protons (Table 1). 1H NMR (acetone-d6-D2O + DCl) δ: 7.08, 6.99, 6.97 (each 2H, s), 6.91, 6.61, 6.46 (each 1H, s). 13C NMR δ: 63.3 (Glc C-6), 70.5 (Glc C-4), 71.8 (Glc C-2), 72.7 (Glc C-5), 73.1 (Glc C-3), 93.5 (Glc C-1), 107.9 (tergalloyl C-3’), 108.0 (tergalloyl C-3), 108.3 (tergalloyl C-6”), 109.9 (galloyl C-2’, 6’), 110.0 (galloyl C-2”, 6”), 110.1 (galloyl C-2, 6), 115.9 (tergalloyl C-1’), 116.1 (tergalloyl C-1”), 116.6 (tergalloyl C-1), 119.2 (galloyl C-1”), 119.8 (galloyl C-1’), 119.9 (galloyl C-1), 124.7 (tergalloyl C-2), 131.4 (tergalloyl C-2’), 136.7 (tergalloyl C-5’), 137.1 (tergalloyl C-5), 139.3 (tergalloyl C-4”), 139.5 (galloyl C-4”), 139.8 (tergalloyl C-2”, galloyl C-4’), 139.9 (tergalloyl C-3”, galloyl C-4), 142.2 (tergalloyl C-5”), 144.5 (tergalloyl C-6), 145.1 (tergalloyl C-4), 145.7 (galloyl C-3”, 5”), 145.9 (galloyl C-3’, 5’), 146.1 (galloyl C-3, 5), 149.6 (tergalloyl C-4’), 150.0 (tergalloyl C-6’), 165.2 (galloyl C-7), 166.1 (galloyl C-7’), 166.6 (galloyl C-7”), 168.0 (tergalloyl C-7), 168.1 (tergalloyl C-7’), 171.5 (tergalloyl C-7”).
Bicornin (3): An off-white amorphous powder, [α]D -11.2o (c 0.7, MeOH). ESIMS m/z: 1087 [M-H]-. HRESIMS m/z: 1087.0906 [M-H]- (calcd. for C48H32O30 - H, 1087.0906). CD [θ] (nm, in MeOH): [θ]224 +4.9 × 104, [θ]258 -1.0 × 104, [θ]289 +1.9 × 104. 1H NMR δ: 7.06 (2H, s), 6.97 (2H, s), 6.95 (2H, s), 6.93 (1H, s), 6.84 (1H, s), 6.52 (1H, s), glucose protons (Table 1). 1H NMR (CD3OD) δ: 7.04 (2H, s), 6.95 (2H, s), 6.93 (2H, s), 6.90 (1H, s), 6.87 (1H, s), 6.49 (1H, s), 6.12 (1H, d, J = 8 Hz, Glc H-1), 5.77 (1H, t, J = 9.5 Hz, Glc H-3), 5.54 (1H, dd, J = 8, 9.5 Hz, Glc H-2), 5.32 (1H, dd, J = 6, 13 Hz, Glc H-6), 5.30 (1H, t, J = 9.5 Hz, Glc H-4), 4.45 (1H, dd, J = 6, 9.5Hz, Glc H-5), 3.98 (1H, d, J = 13 Hz, Glc H-6). 13C NMR δ: 63.9 (Glc C-6), 70.8 (Glc C-4), 71.8 (Glc C-2), 72.6 (Glc C-5), 73.2 (Glc C-3), 93.5 (Glc C-1), 106.9 (tergalloyl C-3), 109.7 (tergalloyl C-6”), 110.0 (galloyl C-2’, 6’), 110.10 (galloyl C-2”, 6”), 110.13 (galloyl C-2, 6), 112.3 (tergalloyl C-1”), 112.4 (tergalloyl C-3’), 113.9 (tergalloyl C-1), 119.2 (galloyl C-1), 119.8 (galloyl C-1’), 119.9 (galloyl C-1”), 120.8 (tergalloyl C-1’), 124.7 (tergalloyl C-2), 130.4 (tergalloyl C-2’), 132.1 (tergalloyl C-3”), 136.1 (tergalloyl C-5), 136.4 (galloyl C-4”), 139.3 (galloyl C-4’), 139.5 (galloyl C-4), 139.9 (tergalloyl C-4”), 142.8 (tergalloyl C-5’), 143.4 (tergalloyl C-2”), 143.9 (tergalloyl C-5”), 145.1 (tergalloyl C-6’), 145.2 (tergalloyl C-6), 145.8 (galloyl C-3”, 5”), 145.90 (galloyl C-3’, 5’), 145.92 (galloyl C-3, 5), 146.1 (tergalloyl C-4), 148.2 (tergalloyl C-4’), 163.5 (tergalloyl C-7”), 165.2 (galloyl C-7), 166.1 (galloyl C-7’), 166.7 (galloyl C-7”), 167.2 (tergalloyl C-7’), 167.8 (tergalloyl C-7). 13C NMR (CD3OD) δ: 64.4 (Glc C-6), 71.2 (Glc C-4), 72.5 (Glc C-2), 73.3 (Glc C-5), 73.9 (Glc C-3), 94.1 (Glc C-1), 107.2 (tergalloyl C-3), 110.1 (tergalloyl C-6”), 110.4 (galloyl C-2’, 6’), 110.5 (galloyl C-2”, 6”), 110.6 (galloyl C-2, 6), 112.3 (tergalloyl C-1”), 113.0 (tergalloyl C-3’), 114.5 (tergalloyl C-1), 119.7 (galloyl C-1 ), 120.3 (galloyl C-1’), 120.4 (galloyl C-1”), 121.3 (tergalloyl C-1’), 124.9 (tergalloyl C-2), 132.5 (tergalloyl C-2’), 137.0 (tergalloyl C-3”), 137.4 (tergalloyl C-5), 140.1 (galloyl C-4”), 140.3 (galloyl C-4’), 140.7 (galloyl C-4), 141.6 (tergalloyl C-4”), 143.4 (tergalloyl C-5’), 144.2 (tergalloyl C-2”), 144.5 (tergalloyl C-5”), 145.5 (tergalloyl C-6’), 145.6 (tergalloyl C-6), 146.3 (galloyl C-3”, 5”), 146.4 (galloyl C-3’, 5’), 146.5 (galloyl C-3, 5), 146.6 (tergalloyl C-4), 148.9 (tergalloyl C-4’), 165.5 (tergalloyl C-7”), 166.1 (galloyl C-7), 166.9 (galloyl C-7’), 167.5 (galloyl C-7”), 168.2 (tergalloyl C-7’), 169.1 (tergalloyl C-7).
Platycaryanin A (4): An off-white amorphous powder, [α]D -10.6o (c 0.6, MeOH). ESIMS m/z: 1103 [M-H]-. CD [θ] (nm, in MeOH): [θ]238 +2.1 × 105, [θ]262 -6.0 × 104, [θ]286 +4.1 × 104. 1H NMR δ: 7.15 (2H, s), 6.88 (1H, s), 6.59 (1H, s), 6.55 (1H, s), 6.44 (1H, s), 6.36 (1H, s), glucose protons (Table 1). 1H NMR (acetone-d6-D2O + DCl) δ: 7.16 (2H, s), 6.95 (1H, s), 6.62 (1H, s), 6.56 (1H, s), 6.45 (1H, s), 6.39 (1H, s). 1H NMR (CD3OD) δ: 7.11 (2H, s), 6.80 (1H, s), 6.57 (1H, s), 6.54 (1H, s), 6.43 (1H, s), 6.41 (1H, s), 6.14 (1H, d, J = 8.5 Hz, Glc H-1), 5.46 (1H, t, J = 9.5 Hz, Glc H-3), 5.33 (1H, dd, J = 6.5, 13.2 Hz, Glc H-6), 5.22 (1H, t, J = 9.5 Hz, Glc H-4), 5.20 (1H, dd, J = 8.5, 9.5 Hz, Glc H-2), 4.37 (1H, dd, J = 6.5, 9.5 Hz, Glc H-5), 3.92 (1H, d, J = 13.2 Hz, Glc H-6). 13C NMR δ: 63.3 (Glc C-6), 69.0 (Glc C-4), 73.2 (Glc C-5), 75.9 (Glc C-2), 77.0 (Glc C-3), 92.1 (Glc C-1), 107.1 (HHDP C-3’), 107.2 (HHDP C-3), 107.7 (tergalloyl C-3, 3’), 108.3 (tergalloyl C-6”), 110.1 (galloyl C-2, 6), 114.3 (HHDP C-1’), 115.0 (HHDP C-1), 116.6 (tergalloyl C-1’), 116.9 (tergalloyl C-1), 119.4 (galloyl C-1), 124.7 (HHDP C-2), 125.7 (HHDP C-2’), 126.0 (tergalloyl C-2), 131.5 (tergalloyl C-2’), 136.1 (HHDP C-5’), 136.5 (HHDP C-5), 136.8 (tergalloyl C-5’), 137.2 (tergalloyl C-5), 139.1 (tergalloyl C-4”), 139.6 (tergalloyl C-2”), 140.0 (tergalloyl C-3”, galloyl C-4), 142.2 (tergalloyl C-5”), 144.36 (tergalloyl C-6), 144.4 (HHDP C-6’), 144.7 (HHDP C-6), 145.07 (tergalloyl C-4), 145.09 (HHDP C-4, 4’), 146.2 (galloyl C-3, 5), 149.7 (tergalloyl C-4’, 6’), 165.2 (galloyl C-7), 168.1 (tergalloyl C-7), 168.2 (tergalloyl C-7’), 168.8 (HHDP C-7), 169.4 (HHDP C-7’), 171.7 (tergalloyl C-7”). 13C NMR (CD3OD) δ: 64.0 (Glc C-6), 69.6 (Glc C-4), 74.0 (Glc C-5), 76.4 (Glc C-2), 77.7 (Glc C-3), 92.8 (Glc C-1), 107.7 (HHDP C-3, 3’), 108.1 (tergalloyl C-3), 108.4 (tergalloyl C-6”), 108.9 (tergalloyl C-3’), 110.5 (galloyl C-2, 6), 114.9 (HHDP C-1’), 115.6 (HHDP C-1), 116.3 (tergalloyl C-1’), 117.2 (tergalloyl C-1), 119.8 (galloyl C-1), 125.1 (HHDP C-2), 126.0 (HHDP C-2’), 126.3 (tergalloyl C-2), 131.6 (tergalloyl C-2’), 137.3 (HHDP C-5’), 137.5 (HHDP C-5), 137.8 (tergalloyl C-5’), 138.0 (tergalloyl C-5), 139.6 (tergalloyl C-4”), 140.2 (tergalloyl C-2”), 140.8 (tergalloyl C-3”, galloyl C-4), 142.8 (tergalloyl C-5”), 144.8 (tergalloyl C-6), 144.9 (HHDP C-6), 145.0 (HHDP C-6’), 145.8 (tergalloyl C-4), 145.9 (HHDP C-4’), 146.0 (HHDP C-4), 146.7 (galloyl C-3, 5), 150.1 (tergalloyl C-6’), 150.4 (tergalloyl C-4’), 166.1 (galloyl C-7), 169.0 (tergalloyl C-7), 169.2 (tergalloyl C-7’), 169.9 (HHDP C-7), 170.7 (HHDP C-7’), 173.5 (tergalloyl C-7”).
Alunusnin A (5): An off-white amorphous powder, [α]D -13.6o (c 1.0, MeOH). ESIMS: m/z 1085 [M-H]-. HRESIMS m/z: 1085.0741 [M-H]- (calcd. for C48H30O30 - H, 1085.0749). CD (MeOH): [θ]237 +7.2 × 104, [θ]263 -2.7 × 104, [θ]288 +1.1 × 104, [θ]324 -3.2 × 103. 1H NMR δ: 7.15 (2H, s), 6.96 (1H, s), 6.91 (1H, s), 6.59 (1H, s), 6.45 (1H, s), 6.40 (1H, s), glucose protons (Table 1). 13C NMR (CD3OD) δ: 63.8 (Glc C-6), 69.1 (Glc C-4), 73.0 (Glc C-5), 75.9 (Glc C-2), 76.9 (Glc C-3), 92.1 (Glc C-1), 106.6 (tergalloyl C-3), 107.1 (HHDP C-3), 107.2 (HHDP C-3’), 109.7 (tergalloyl C-6”), 110.1 (galloyl C-2, 6), 112.3 (tergalloyl C-1”), 112.5 (tergalloyl C-3’), 113.9 (tergalloyl C-1), 114.4 (HHDP C-1’), 114.9 (HHDP C-1), 119.3 (galloyl C-1), 120.8 (tergalloyl C-1’), 124.6 (tergalloyl C-2), 125.7 (HHDP C-2), 125.9 (HHDP C-2’), 132.0 (tergalloyl C-2’), 136.18 (tergalloyl C-5), 136.2 (HHDP C-5’), 136.5 (HHDP C-5), 140.0 (galloyl C-4), 140.5 (tergalloyl C-4”), 142.8 (tergalloyl C-5’), 143.5, 144.0 (tergalloyl C-5”), 144.39 (HHDP C-6’), 144.41 (HHDP C-6), 145.07 (HHDP C-4’), 145.09 (HHDP C-4), 145.2 (tergalloyl C-6'), 145.9 (tergalloyl C-4), 146.1 (galloyl C-3, 5), 148.2 (tergalloyl-4’), 163.7 (tergalloyl C-7”), 165.2 (galloyl C-7), 167.2 (tergalloyl C-3’), 168.0 (tergalloyl C-3), 168.7 (HHDP C-7), 169.5 (HHDP C-7’).
Cleavage of the depside linkage in 3 and 5:16 A solution of 3 (1.3 mg) in 0.03 M KH2PO4-Na2HPO4 buffer (pH 5.8, 1 mL) was maintained at 37 ºC for 10 h. The solution was acidified with 10% HCl (0.2 mL) and then extracted with EtOAc. Removing the organic layer solvent gave 2 (1 mg). Compound 5 (1 mg) was similarly treated to yield 4. The identities of 2 and 4 were also confirmed by 1H NMR and HPLC.
Transformation of 18 and 20 into 1:25 A mixture of 18 (1.5 mg), 20 (3 mg), and p-toluenesulfonic acid (1.5 mg) in dioxane (300 µL) was heated in a boiling-water bath for 5 h. After evaporation of the solvent, the residue was purified by preparative HPLC to yield 1 (0.8 mg). The identity of 1 was also confirmed by 1H NMR and HPLC.
Antifungal effects on Candida species:1 The method used was described in ref. 1. Briefly, a fungal suspension in nutrient broth was inoculated onto the surface of a Sabouraud agar plate. Each sterile paper disk (8 mm in diameter) was impregnated with 10 µl of sample solution (15 mg/mL) and was placed onto the agar plates. The diameter (in mm) of the growth inhibition zones was measured after incubation at 37 ºC for 24 h. Tannins used in this study were those obtained from Camptotheca acuminata (8, 9),26 Rosa rugosa (12),21 and Casuarina stricta (18). 4
ACKNOWLEDGEMENTS
The Varian INOVA 600AS NMR instrument used in this study is the property of the SC-NMR laboratory of Okayama University. This study was supported, in part, by Intractable Infectious Diseases Research Project Okayama (IIDTPO).
References
1. A. Nozaki, E. Takahashi, K. Okamoto, H. Ito, and T. Hatano, Yakugaku Zasshi, 2010, 130, 895. CrossRef
2. T. Tanaka, Y. Orii, G. Nonaka, I. Nishioka, and I. Kouno, Phytochemistry, 1996, 43, 1345. CrossRef
3. T. Tanaka, Y. Orii, G. Nonaka, and I. Nishioka, Chem. Pharm. Bull., 1993, 41, 1232. CrossRef
4. T. Okuda, T. Yoshida, M. Ashida, and K. Yazaki, J. Chem. Soc., Perkin Trans. 1, 1983, 1765. CrossRef
5. T. Okuda, T. Yoshida, and T. Hatano, Progress in the Chemistry of Organic Natural Products, 1995, 66, 1.
6. M. Yoshimura, H. Ito, K. Miyashita, T. Hatano, S. Taniguchi, Y. Amakura, and T. Yoshida, Phytochemistry, 2008, 69, 3062. CrossRef
7. G. Nonaka, T. Sakai, T. Tanaka, K. Mihashi, and I. Nishioka, Chem. Pharm. Bull., 1990, 38, 2151. CrossRef
8. T. Okuda, T. Yoshida, T. Hatano, T. Koga, N. Toh, and K. Kuriyama, Tetrahedron Lett., 1982, 23, 3937. CrossRef
9. S. B. Kalidhar, M. R. Parthasarathy, and P. Sharma, Indian J. Chem. B, 1981, 720.
10. C. K. Wilkins and B. A. Bohm, Phytochemistry, 1976, 15, 211. CrossRef
11. T. Yoshida, T. Hatano, T. Kuwajima, and T. Okuda, Heterocycles, 1992, 33, 463. CrossRef
12. T. Fukuda, H. Ito, and T. Yoshida, Phytochemistry, 2003, 63, 795. CrossRef
13. T. Tanaka, S. Kirihara, G. Nonaka, and I. Nishioka, Chem. Pharm. Bull., 1993, 41, 1708. CrossRef
14. M. Ishimatsu, T. Tanaka, G. Nonaka, and I. Nishioka, Phytochemistry, 1989, 28, 3179. CrossRef
15. T. Hatano, N. Ogawa, T. Yasuhara, and T. Okuda, Chem. Pharm. Bull., 1990, 38, 3308. CrossRef
16. T. Hatano, K. Yazaki, A. Okonogi, and T. Okuda, Chem. Pharm. Bull., 1991, 39, 1689. CrossRef
17. T Yoshida, O. Namba, L. Chen, Y. Liu, and T. Okuda, Chem. Pharm. Bull., 1990, 38, 3296. CrossRef
18. T. Hatano, N. Ogawa, R. Kira, T. Yasuhara, and T. Okuda, Chem. Pharm. Bull., 1989, 37, 2083. CrossRef
19. E. A. Haddock, R. K. Gupta, S. M. K. A. Shafi, E. Haslam, and D. Magnolato, J. Chem. Soc., Perkin Trans.1, 1982, 2515. CrossRef
20. T. Yoshida, Z. X. Jin, and T. Okuda, Chem. Pharm. Bull., 1991, 39, 49. CrossRef
21. T. Hatano, N. Ogawa, T. Shingu, and T. Okuda, Chem. Pharm. Bull., 1990, 38, 3341. CrossRef
22. T. Yoshida, T. Maruyama, A. Nitta, and T. Okuda, Phytochemistry, 1996, 42, 1171. CrossRef
23. G. Nonaka, T. Sakai, K. Mihashi, and I. Nishioka, Chem. Pham. Bull., 1991, 39, 884. CrossRef
24. T. Yoshida, F. Nakata, K. Hosotani, A. Nitta, and T. Okuda, Chem. Pham. Bull., 1992, 40, 1727. CrossRef
25. T. Hatano, S. Shida, L. Han, and T. Okuda, Chem. Pharm. Bull., 1991, 39, 876. CrossRef
26. T. Hatano, Y. Ikegami, T. Shingu, and T. Okuda, Chem. Pharm. Bull., 1988, 36, 2017. CrossRef