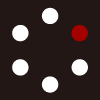
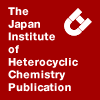
HETEROCYCLES
An International Journal for Reviews and Communications in Heterocyclic ChemistryWeb Edition ISSN: 1881-0942
Published online by The Japan Institute of Heterocyclic Chemistry
e-Journal
Full Text HTML
Received, 22nd November, 2011, Accepted, 19th December, 2011, Published online, 21st December, 2011.
DOI: 10.3987/REV-11-723
■ Role of Ionic Liquids as Supports of Catalysts
Hisahiro Hagiwara*
Graduate School of Science and Technology, Niigata University, 8050, 2 no-cho, Ikarashi, Niigata 950-2181, Japan
Abstract
Chemically stable and independent properties of an ionic liquid has been utilized as a liquid support for immobilization of homogeneous or heterogeneous catalysts by simply dissolving or suspending in an ionic liquid. After triturating products with molecular solvent, the catalyst, which was kept in the ionic liquid, is separated, recovered and used repeatedly. Covalent bonding of catalyst residue on an ionic liquid is an alternative method of immobilization. Confine of homogeneous catalyst with the aid of an ionic liquid in pores of amorphous solid (SILPC protocol) is simple and efficient way of immobilization for higher activity, recycle use, easy handling and low cost. Recent representative developments in these areas are reviewed.I OVERVIEW OF AN IONIC LIQUID AS A LIQUID SUPPORT
An ionic liquid is a chemically stable liquid having non-volatility, non-lipophilicity, hydrophobicity and thermal stability. These characteristic features derive from non-symmetrical structures of organic cations and hydrophobic fluorous counter anions in contrast to a combination of symmetrical organic cations and hard anions such as surfactants, which enable an ionic liquid as a recyclable reaction medium in synthetic organic chemistry due to facile phase separation not only from common organic solvents but also from water. When mixed with water and organic solvent, these liquids clearly separate into three phases due to immiscibility in each other, in which an ionic liquid comes at the bottom due to higher density. After a reaction in an ionic liquid, organic solvent and water could extract product and inorganic salt, respectively. If the catalyst is maintained in the ionic liquid after extractions, recycle use of the catalytic system is possible, in which an ionic liquid works as a support of catalyst.
When the phases do not separate clearly, addition of alcohol or salt might be effective for separation. Tuning combination of cation and anion of an ionic liquid is an alternative way for better separation. Very small volatility of an ionic liquid enables sublimation or distillation of products directly in vacuo from an ionic liquid layer. If the catalyst is thermally stable, the ionic liquid and the catalyst could be used repeatedly. Supercritical carbon dioxide is compatible for product extraction due to negative solubility of anhydrous ionic liquid, especially in the case when an ionic liquid becomes homogeneous with common organic solvent.
An ionic liquid as a designer solvent is an additional attractive feature, in which numerous combinations of anions and cations are expected for a variety of new ionic liquids having novel properties. Among a variety of ionic liquids, most commonly and conventionally used ionic liquids are imidazolium ionic liquids (Figure 1). Alternative cation is pyridinium, ammonium or phosphonium cation. As anions, bis(trifluoromethanesulfonyl)imides (TFSI), nitrate or trifluoromethanesulfonate anion is used often.
In addition to the role of an ionic liquid as non-covalent support of catalysts, catalytic residue could be immobilized by covalent bonding on an ionic liquid. The ionic liquid, which tagged catalyst or ligand, is easy to recover due to higher affinity with an ionic liquid and clear phase separation with common organic solvent.
These characteristic features are distinguished from so-called environmentally benign green media such as water, polyethylene glycol, fluorous solvent or supercritical carbon dioxide. Water is safe and cheap, though solubility of substrates or catalysts is a major issue. Most organometallic catalysts are hydrolyzed in aqueous media. Special tuning of catalysts is required for recycle use in water. Polyethylene glycol has the same features as water. Fluorus solvent dissolves and maintains organofluorous compounds and catalysts, though substitution of reagents with fluorine atom limits widespread use. Thermal stability is moderate compared to an ionic liquid. Supercritical carbon dioxide is obtained under mild conditions and chemically and physically stable, which lacks ability as a support of catalysts. Use of high pressure apparatus restricts conventional use.
Higher solubility of substrate and catalyst along with low solubility of product are ideal in a catalytic reaction in an ionic liquid, because the product could be isolated by partition with conventional organic solvent, in which the catalyst must be maintained in an ionic liquid for recycle use. Proper selection and tuning of an ionic liquid are desireable to demonstrate higher reactivity, selectivity and recyclability of the reaction in an ionic liquid.
Catalytic reactions in an ionic liquid have been focused particularly on the recycle use of both the catalyst and the ionic liquid from the standpoint of green chemistry, as mentioned above, because an ionic liquid does not leach out in environment due to its characteristic properties, if treated properly. Ionic organometallic ctalyst is maintained in an ionic liquid, which does not require special design of ligand. Most central metals and reaction intermediates are electrophilic, but an ionic liquid is neutral to these species due to the low nucleophilicity of delocalized anions of an ionic liquid. A cationic intermediate of a reaction may be stabilized by interaction with the anion of an ionic liquid. While imidazolium cation is unreactive to catalysts, N-heterocyclic carbene (NHC) is easily formed by base thereby ligating, stabilizing and activating central metal, because the pKa value of the proton at C-2 is about 21, which is comparable to isopropyl alcohol. Otherwise, NHC complex is formed by oxidative addition of zero valent metal into C-H bond at C-2 (Figure 2). In the case when the metal is neutral, organometallic catalyst is extracted on work-up along with product from the ionic liquid layer. Tuning of ligand having high affinity with an ionic liquid is required.
In the same manner, neutral organomolecular catalyst needs special tuning to recycle in an ionic liquid. Low solubility of substrates and catalysts in ionic liquids are improved by addition of co-solvent, which is also useful to carry out reaction at low temperature.
Although an ionic liquid have many advantages as a reaction medium, one issue is its high cost for large-scale use in spite of commercial availability. Realization of an idea to use minimum amount or recycle of an ionic liquid is desirable for cost-cut.
Because there is a large volume of accumulations on catalytic reactions in ionic liquids,1 comprehensive review is beyond the scope of this article, in which some representative examples are mentioned to advocate utilities of ionic liquids as supports of catalysts.
II REACTION CATALYZED BY ORGANOMETALLIC CATALYSTS IN AN IONIC LIQUID
It is not an overstatement that almost all known organometallic reactions in molecular solvents have been carried out in ionic liquids so far. Substrates and catalysts are surrounded and solvated only by ions, in which reactions proceed under totally different environment.
One of the most investigated is the reaction catalyzed by Pd.2 Pd-NHC complex as an active catalytic species generated from imidazolium cation (Figure 2) or Pd nano-particles (vide infra) is proposed in the most cases.3
Mizoroki-Heck reaction in [bmim]PF6 was reported employing Pd(OAc)2. After triturating the product with ether, Pd and the ionic liquid was used repeatedly.4 Addition of a small amount of PPh3 enhanced the reaction.5 While, the reaction catalyzed by more stable Pd/C was reported in [bmim]PF6 without adding ligand, in which suspension of the Pd/C in the ionic liquid was recycled.6
Suzuki-Miyaura reaction is an another representative of Pd catalyzed reaction. Because substrates are stable and the reaction is carried out under ambient reaction condition, the reaction have been applied for process scale production in pharmaceutical as well as electroorganic industries. Reaction rate and TON increased in an ionic liquid in several applications. In [bmim]BF4, pre-heating of Pd(PPh3)4 and arylhalide enabled coupling at room temperature in a shorter period of time,7 which suggests formation of Pd-NHC complex (Figure 2). The product was triturated with ether, while boronic acid salt was washed away with water, and the ionic liquid layer keeping Pd was re-used again.8
Sonogashira reaction was carried out in [bmim]BF4 without addition of ligand. Formation of Pd nano-particles was observed by in situ TEM measurement during the reaction by super sonic irradiation.9
In [bedim]BF4, Negishi reaction proceeded with imidazolium cation having diphenylphosphino group at C-2 as a ligand, though recycle experiment was not efficient.10
Stille reaction with PdCl2(PhCN)2, PPh3As and CuI in [bmim]BF4 was slow than the reaction in N-methylpyrrolidinone, accompanying homo-coupling byproduct.11
Pd catalyzed Tsuji-Trost reaction was also carried out in an ionic liquid.12
A variety of gas dissolves well in an ionic liquid. Hydroformylation of terminal olefins were well investigated in ionic liquids with Pt, Ru or Rh catalyst.13 In the hydroformylation, [Rh2(OAc)4] and P(OPh)3 were designed in a flow reactor system which combined [bmim]PF6 and supercritical carbon dioxide.14 Bidentate ligand having xanthene framework (Figure 3) improved the yield of linear aliphatic aldehyde and recyclability of the system.15
The yield of carbonylation of aryl halides with carbon monoxide increased in [bmim]BF4 than the reaction in alcohol. The decreased catalytic activity of Pd in an ionic liquid as a result of recycle use was retrieved by addition of PPh3.16
An ionic liquid is stable under oxidative condition and was used for oxidation reaction17 such as Wacker oxidation18 or Ni catalyzed oxidation of benzaldehyde.19 Oxidation of primary alcohol in an ionic liquid by TEMPO-CuCl provided only aldehyde even under aqueous condition.20 Asymmetric epoxidation of allylether by chiral Mn(III)(salen) complex21 and epoxidation of olefin by methyltrioxorhenium in ionic liquids were reported.22 In the asymmetric epoxidation by Jacobson catalyst, dichloromethane was added as a co-solvent in order to carry out the reaction at low temperature.23
Catalytic hydrogenation in an ionic liquid is also well investigated. In catalytic hydrogenation by Wilkinson catalyst,24 product was triturated with supercritical carbon dioxide in order to maintain the catalyst in an ionic liquid layer for recycle use because of its neutral and thus lipophilic nature.25 Rh or Ru catalyst having chiral ligand (Figure 4) has been used for catalytic asymmetric hydrogenation of α-acetamidocinnamic acid, 2-phenylacrylic acid and tiglic acid.26 Reduction of diene with Pd(acac)2 in [bmim] BF4 afforded mono-ene selectively.27
Development of the Grubbs or Schrock catalysts for olefin metathesis reaction has changed the concepts of contemporary synthetic organic chemistry. Widespread use of the catalysts stimulated recycle use of the catalysts due to their high cost, large molecular weight, low TON and instability. Although there are several precedents of metathesis reactions in an ionic liquid,28 recycle use of catalysts are not efficient, because catalysts are easily partitioned into even in n-hexane due to neutral and lipophilic nature.
Weakly coordinated catalysts are used often for co-polymerization of alkenes. Highly polar and less coordinative ionic liquids are expected to be interesting solvents for co-polymerization. Some representative results are ethylene oligomerization with Ni catalysts (Figure 5) in [bmim]PF629 and alternating co-polymerization of styrene and carbon monoxide with (bipy)Pd(OAc)2,30 though catalyst recycling was not carried out.
Not only organometallic catalysts but also Lewis acids were immobilized in an ionic liquid. (S)-BINOL In(III) complex was repeatedly used in [hmim]PF6 for enantioselective Diels-Alder cycloaddition.31
III PREPARATION OF METAL NANO-PARTICLES AND CATALYTIC REACTIONS
Metal nano-particles are expected high catalytic activities due to its fine size and wide surface area.32 In order to disturb aggregation of nano-particles and keep dispersive property in a solution, addition of quaternary ammonium salt is useful. Actually, metal nano-particles do not aggregate and disperse homogeneously in an ionic liquid due to the interaction between cationic surface of the metal and the anion. Ni nano-particles were obtained by the reduction of bis(η4-1,5-cyclooctadiene)nickel(0) or Ir[ClCOD]2 under 5 MPa of hydrogen in [bmim]TFSI or [bmim]PF6 at 70 °C, which were effective for recyclable catalytic hydrogenations.33 Nano-particles of Pd, Ir, Ge and nano-wire of Ti have been prepared by electro-organic methods.34 Reduction in ionic liquids provided fine nano-particles of Au, Ir, Pd, Pt, Rh and Ru of the same size.34 Irradiation of laser is an alternative way to prepare nano-particles of Pd and Rh.35 Pd cluster of 3 nm was applied to Mizoroki-Heck reaction in the presence of ammonium salt such as n-Bu4N+Cl- or n-Oct4N+(HCO2)-. Formation of Pd nano-particles was observed by TEM after Mizoroki-Heck reaction in [bmim]PF6 or [bmim]BF4.36 These Pd nano-particles are considered to be a reservoir of Pd active species,36 in which Pd-NHC complex was identified by NMR.36b
The Pd-nano-particles stabilized by phenanthroline exhibited higher catalytic activity and recyclability in the catalytic hydrogenation of olefins.37 Stable Rh nano-catalyst was employed for hydrogenation of aromatic compounds.38
IV CATALYTIC REACTIONS BY ORGANOMOLECULAR CATALYSTS IN AN IONIC LIQUID
Organomolecular catalyst attracts currently much attention as an environmentally benign catalyst due to advantages such as rational design, easy production, easy disposal, and absence of heavy metals. Numerous efforts have been devoted for the developments of new catalysts. In spite of these advantages, recycle use of the catalyst is anticipated with the aid of an ionic liquid, because of homogeneity of the catalysts in molecular solvent, moderate stability and TON.39
Proline and its derivatives have been employed for a variety of nucleophilic reactions, and thus there are several applications in an ionic liquid.40 To improve enantioselectivity, the reaction has to be carried out at lower temperature, in which co-solvent such as DMF was added to increase the solubility of substrates and catalyst and to lower melting point of the ionic liquid. Recycle of expensive unnatural D-phenylalanine was reported in [bmim]PF6 with dimethylimidazolidinone as a co-solvent (Eq. 1) in intramolecular aldol condensation leading to Wieland-Miescher ketone, which is a very versatile compound for total syntheses of terpenoids.41 Recycle use of MacMillan imidazolidinone (Figure 6) was investigated in an ionic liquid for enantioselective Diels-Alder reaction.42 Chiral pyrollidine derived from proline catalyzed direct 1,4-conjugate addition of intact aldehyde to vinylketone in [bmim]PF6 in moderate chemical and enatiomeric yields (Figure 7).43
V TASK SPECIFIC IONIC LIQUIDS AND CATALYTIC REACTIONS
Task specific ionic liquid is an ionic liquid, which was tagged with functional residues such as catalyst, ligand, electroconductor and so on.44 Characteristic chemical features of an ionic liquid are installed to the catalyst, which gives higher affinity with an ionic liquid and non-affinity with conventional liquids.
n order to improve highly lipophilic nature of the Grubbs catalysts, an ionic liquid residue was incorporated into the ligand of Grubbs catalyst, which was successfully recovered in an ionic liquid and recycled in metathesis reactions (Figures 8 and 9).45
Characteristic features of task specific ionic liquids are well exploited for immobilization of organomolecular catalyst and recycle use. An ionic liquid was tagged with sulfonic acid residue (Figure 10), which was effective for acid catalyzed transformation such as esterification or pinacol reactions.46 Similarly, the catalyst was employed for Friedel-Crafts reaction, nitration of aromatic compounds and Beckmann rearrangement.47 Sulfonic acidic ionic liquid having TfO- anion was used for condensation of neat indole and 1,3-dicarbonyl compound leading to 3-alkenylated indole (Figure 11)48 and for Ritter reaction of amide and tertiary alcohol leading to amide.49 Not only Brönsted acidic task specific ionic liquid but also basic task specific ionic liquid was reported, in which Hunig base was immobilized on quaternary ammonium salt (Figure 12) for Knoevenagel condensation, though the activity decreased on recycle use.50
Enantioselective aldol reaction by proline with an ionic liquid tag (Figure 13) proceeded in high enantioselectivity.51 Conjugate addition of cyclohexanone to nitroolefin was catalyzed by tagged pyrrolidine (Figure 14) in highly diastereo- and enantio-selective manner.52 Since proline does not dissolve easily even in polar solvent such as DMSO, higher solubility in an ionic liquid improved reactivity and recyclability of proline and its derivatives.
The ionic liquid tagged with iodoarene was useful for the preparation of α-tosyloxyketone, oxazole or benzenesultam (Figure 15).53 Oxidation of alcohol was carried out with an ionic liquid having N-hydroxyimide tag (Figure 16).54 Similarly, ionic liquid was tagged with TEMPO by ether or ester linkage for oxidation of alcohol (Figures 17 and 18).55 The ionic liquid tagged with porphycene sensitizer (Figure 19) was recycled in oxidation by singlet oxygen, which enabled de-chlorination of trichlorophenol.56 Some reactions were carried out in conventional solvents and task specific ionic liquids were recovered and re-used several times after precipitation by poor solvent.
n order to make separation of the task specific ionic liquid more easily on recycle use, the acidic task specific ionic liquid residue was grafted on the surface of silica (Figure 20), which was re-used easily for esterification and nitration of aromatic compounds after filtration. The esterification between long chain carboxylic acid and long chain alcohol was successful without solvent, while the nitration proceeded without concentrated sulfuric acid. 57 The catalyst was also effective to 1,2-58 and 1,4-addition59 of indoles in conventional solvents and could be recycled after filtration (Eq. 2).
An acidic ionic liquid grafted on polystyrene beads was applied to esterification, which was recovered by filtration.60 PEG was an alternative organic support of acidic ionic liquid, which was recovered by precipitation after cooling to room temperature from homogeneous toluene solution (Figure 21).61
VI IMMOBILIZATION OF HOMOGENEOUS CATALYST WITH THE AID OF IONIC LIQUIDS ON SOLID SUPPORT AS SILPC
Although an ionic liquid is a very useful liquid support for a variety of catalysts, there still remain some issues especially for large-scale use. The cost of an ionic liquid is still high. High viscosity of an ionic liquid prevents efficient diffusion of substrates thereby decreasing reaction rate and makes extraction of the product laborious during work-up. SILPC protocol (Supported Ionic Liquid Phase Catalyst), which immobilizes an ionic liquid solution of a catalyst into pores of amorphous inorganic oxide, is a promising method to cope with these issues (Figure 22).62 Preparation of SILPC is quite simple. After amorphous inorganic solids were suspended in a THF solution of homogeneous catalyst and an ionic liquid, evacuation of the solvent provided SILPC. The catalyst was immobilized in a thin layer of the ionic liquid, which spread over a wide surface area of pores of inorganic solids. The ionic liquid layer was kept mainly by hydrogen bonding63 between protons at C-2 of the imidazolium cation of an ionic liquid and lone pairs of normal or reversed phase inorganic oxide. Electrostatic interactions between ionic liquid and solid might be secondary interaction. Catalytic reaction proceeds by partition of the substrates into the thin ionic liquid layer inside of pores from outer solution phase (Figure 22). Higher viscosity of an ionic liquid does not interfere reactions anymore.
This protocol has a number of advantages: 1) A variety of catalysts soluble in an ionic liquid could be immobilized. 2) Immobilization could be easily carried out non-covalently without chemical transformations. 3) Catalyst might be activated in an ionic liquid. 4) Sophisticated and unstable catalyst might be stabilized by dissolution in an ionic liquid due to the absence of direct contact with the surface of solid support different from conventional sintering method. 5) Delocalization of catalyst in pores of solid support increases concentration of the catalyst. 6) Reactions could be carried out in conventional solvents. 7) Technical know-how accumulated on the homogeneous reaction could be applicable. 8) Product and SILPC could be separable by filtration. 9) SILPC could be recyclable after filtration without pre-treatment. 10) Small amount of an ionic liquid is enough for SILPC immobilization, which is cost effective. Pd(OAc)2, CuBr, Grubbs Ru catalyst were immobilized as Pd-SILPC, Pd-nano-SILPC, Pd-SH-SILPC, Cu-SILPC, Ru-SILPC, respectively in the pores of normal or reversed phase amorphous silica, alumina or nano-silica gel dendrimer as solid supports with the aid of ionic liquids. MacMillan imidazolidinone was also immobilized as Mac-SILPC. These heterogenized homogeneous catalysts are employed successfully for Mizoroki-Heck reaction,64 Suzuki-Miyaura reaction,65 catalytic hydrogenation,66 Huisgen[3+2]cycloaddition,67 olefin metathesis reaction,68 enantioselective Diels-Alder reaction,69 and exhibited higher catalytic activities and recyclability than their homogeneous counterparts (Figure 23). Mizoroki-Heck reaction proceeded in water, when the SILPC was prepared from N,N-diethylaminopropylated silica.64b Notably, Pd-SILPC immobilized on reversed-phase alumina recorded TON of 2x107 and TOF 3x104 h-1 in the Suzuki-Miyaura reaction in 50% aqueous ethanol.65a In this system, formation of very fine Pd-nano-particles was observed by TEM. Pd-nano-SILPC immobilized on nano-silica gel dendrimer was effective to afford ortho-substituted biphenyl at room temperature.65b TEM observation of the Pd-nano-SILPC showed worm-hole structure involving nano-Pd clusters, which is critical for high catalytic activity. Catalytic hydrogenation by the SILPC immobilized on mercaptopropylated silica gel (Pd-SH-SILPC) was recyclable (up to 10 times), mild and selective enough that formyl, bromoaryl, benzylether, and Cbz groups remained intact, while tetra-substituted α,β-enones were reduced at room temperature at ambient pressure. Catalytic reactions by SILPC except metathesis reaction proceeded at room temperature without a ligand in a shorter period of time. SILPCs were recovered by filtration and re-used several times. Coating SILPC surface with PET enhanced durability of Pd-SILPC up to 10 times in Suzuki-Miyaura reaction.70
Covalent bonding of an ionic liquid residue on solid surface and immobilization of homogeneous catalyst is an alternative way of SILPC immobilization. Imidazolium cation was grafted on the surface of silica gel (Figure 24) for immobilizations of Pd or Cu salt, which were employed for recycle of Suzuki-Miyaura or Kharasch reaction, respectively.71 Pd-EDTA immobilized in an ionic liquid brush (Figure 25) was applied to Suzuki-Miyaura reaction.72 Similarly, peroxotungstates were immobilized for selective oxidation of sulfides by hydrogen peroxide.73 The surface of carbon nano-tube film was grafted with imidazolium cation by amide bond to immobilize Rh, which was effective for recyclable catalytic hydrogenation.74 After grafting the surface of MCM-48 with ionic liquid residue, Mn(III) salen complex was immobilized in the presence of an external ionic liquid for asymmetric epoxidation and exhibited high enantiomeric excess for cyclic olefins (Figure 26).75 The same protocol was applied to Suzuki-Miyaura reaction by the SILPC immobilized on inner surface of mesoporous silica SBA-15.76 Active carbon cloth was employed for SILPC immobilization of Pt for catalytic hydrogenation of citral.77
Because an ionic liquid is thermally stable, SILPC protocol is suitable at high temperature for vapor phase reaction in a flow reactor. Starting from hydroformylation78 and hydrogenation79 by Mehnert, hydroformylation in a fixed-bed flow reactor by Wasserscheid followed.80 Since then, a group of Wasserscheid and Riisager actively developed gas phase reaction such as water-gas-shift reaction81 and methanol carbonylation.82 Friedel-Crafts isopropylation in a batch reactor employing aluminum ionic liquid was a new application.83 Vanadium-SILPC on silica gel employing VO(acac)2 and [BuPy]CF3CO2– was employed in an attempt to remove VOC such as styrene or benzylalcohol by air-oxidation.84 Although vapor phase reaction is limited to volatile and less functionalized substrates of low molecular weight, leaching of metal catalyst is suppressed minimum, which might be suitable for industrial scale reaction in a flow system.
VII CONCLUSIONS
Characteristic features of an ionic liquid make non-covalent immobilization of catalysts very facile due to the absence of chemical transformations, which could be applicable not only to homogeneous catalysts but also to heterogeneous catalysts. Some catalysts are stabilized and activated in an ionic liquid to exhibit higher reactivity than in conventional molecular solvent. Take-up of products from an ionic liquid could be easily carried out and the catalysts could be recycled. Moreover, SILPC protocol, which confines an ionic liquid solution of a catalyst in pores of amorphous solid, is highly promising due to easy handling, high catalytic performance and low cost. Covalent bonding of catalyst residues on ionic liquids is an additional protocol, which utilizes better affinity with ionic liquids for recycle use. These protocols are very attractive and present higher potential for immobilization of almost all known catalysts not only for process scale but also for industrial scale reactions.
References
1. Review: (a) ‘Ionic Liquids in Synthesis,’ ed. by P. Wasserscheid and T. Welton, Wiley-VCH Verlags, GmbH&Co, KGaA, Weinheim, 2nd ed., 2008; (b) V. I. Parvulescu and C. Hardacre, Chem. Rev., 2007, 107, 2615; CrossRef (c) S. Chowdhury, R. S. Mohan, and J. L. Scott, Tetrahedron, 2007, 63, 2363; CrossRef (d) T. Welton, Coordination Chem. Rev., 2004, 248, 2459; CrossRef (e) H. Olivier-Bourbigou and L. Magna, J. Mol. Cat. A: Chemical, 2002, 182-183, 419; CrossRef (f) J. Dupont, R. F. de Souza, and P. A. Z. Suarez, Chem. Rev., 2002, 102, 3667; CrossRef (g) D. Zhao, M. Wu, Y. Kou, and E. Min, Catalysis Today, 2002, 74, 157; CrossRef (h) C. M. Gordon, Applied Catalysis A: General, 2001, 222, 101; CrossRef (i) R. Sheldon, Chem. Commun., 2001, 2399. CrossRef
2. Review: (a) R. Singh, M. Sharma, R. Mamgain, and D. S. Rawat, J. Braz. Chem. Soc., 2008, 19, 357; CrossRef (b) A. Molnar, Chem. Rev., 2011, 111, 2251. CrossRef
3. L. S. Ott, M. L. Cline, M. Deetlefs, K. R. Seddon, and R. G. Finke, J. Amer. Chem. Soc., 2005, 127, 5758. CrossRef
4. A. J. Carmichael, M. J. Earle, J. D. Holbrey, P. B. McCormac, and K. R. Seddon, Org. Lett., 1999, 1, 997. CrossRef
5. L. Xu, W. Chen, and J. Xiao, Organometallics, 2000, 19, 1123. CrossRef
6. H. Hagiwara, Y. Shimizu, T. Hoshi, Suzuki, M. Ando, K. Ohkubo, and C. Yokoyama, Tetrahedron Lett., 2001, 42, 4349. CrossRef
7. F. McLachlan, C. J. Mathews, P. J. Smith, and T. Welton, Organometallics, 2003, 22, 5350. CrossRef
8. C. J. Mathews, P. J. Smith, and T. Welton, Chem. Commun., 2000, 1249. CrossRef
9. A. R. Gholap, K. Venkatesan, R. Pasricha, T. Daniel, R. J. Lahoti, and R. V. Srinivasan, J. Org. Chem., 2005, 70, 4869. CrossRef
10. J. Sirieix, M. Ossberger, B. Betzemeier, and P. Knochel, Synlett, 2000, 1613. CrossRef
11. S. T. Handy and X. Zhang, Org. Lett., 2001, 3, 233. CrossRef
12. C. de Bellefon, E. Pollet, and P. Grenouillet, J. Mol. Cat. A, 1999, 145, 121. CrossRef
13. Review: M. Haumann and A. Riisager, Chem. Rev., 2008, 108, 1474. CrossRef
14. M. F. Sellin, P. B. Webb, and D. J. Cole-Hamilton, Chem. Commun., 2001, 781. CrossRef
15. P. Wasserscheid, H, Waffenschmidt, P. Machnitzki, K. W. Kottsieper, and O. Stelzer, Chem. Commun., 2001, 451. CrossRef
16. E. Mizushima, T. Hayashi, and M. Tanaka, Green Chem., 2001, 3, 76. CrossRef
17. J. Muzart, Adv. Synth. Catal., 2006, 348, 275. CrossRef
18. V. V. Namboodiri, R. S. Varma, E. Sahle-Demessie, and U. R. Pillai, Green Chem., 2002, 4, 170. CrossRef
19. J. Howarth, Tetrahedron Lett., 2000, 41, 6627. CrossRef
20. I. A. Ansari and R. Gree, Org. Lett., 2002, 4, 1507. CrossRef
21. C. E. Song and E. J. Roh, Chem. Commun., 2000, 837. CrossRef
22. G. S. Owens and M. M. Abu-Omar, Chem. Commun., 2000, 1165. CrossRef
23. C. E. Song and E. J. Roh, Chem. Commun., 2000, 837. CrossRef
24. F. Liu, M. B. Abrams, T. Baker, and W. Tumas, Chem. Commun., 2001, 433. CrossRef
25. Y. Chauvin, L. Mussmann, and H. Olivier, Angew. Chem., Int. Ed., 1995, 34, 2698. CrossRef
26. R. A. Brown, P. Pollet, E. McKoon, C. A. Eckert, C. L. Liotta, and P. G. Jessop, J. Amer. Chem. Soc., 2001, 123, 1254. CrossRef
27. J. Dupont, P. A. Z. Suarez, A. P. Umpierre, and R. F. de Souza, J. Braz. Chem. Soc., 2000, 11, 293. CrossRef
28. (a) D. Semeril, H. Olivier-Bourbigou, C. Bruneau, and P. H. Dixneuf, Chem. Commun., 2002, 146; CrossRef (b) R. C. Buijsman, E. van Vuuren, and J. G. Sterrenburg, Org. Lett., 2001, 3, 3785. CrossRef
29. P. Wasserscheid, C. M. Gordon, C. Thlgers, M. J. Mulddon, and J. R. Dunkin, Chem. Commun., 2001, 1186. CrossRef
30. M. A. Klingshirn, G. A. Broker, J. D. Holbrey, K. H. Shaughnessy, and R. D. Rogers, Chem. Commun., 2002, 1394. CrossRef
31. F. Fu, Y.-C. Teo, and T.-P. Loh, Org. Lett., 2006, 8, 5999. CrossRef
32. D. Astruc, F. Lu, and J. R. Aranzaes, Angew. Chem. Int. Ed., 2005, 44, 7852. CrossRef
33. (a) P. Migowski, S. R. Teixeira, G. Machado, M. C. M. Alves, J. Geshev, and J. Dupont, J. Electron Spectroscopy and Related Phenomena, 2007, 156–158, 195; CrossRef (b) J. Dupont, G. S. Fonseca, A. P. Umpierre, P. F. P. Fichtner, and S. R. Teixeira, J. Amer. Chem. Soc., 2002, 124, 4228. CrossRef
34. Review: (a) P. Migowski and J. Dupont, Chem. Eur. J., 2007, 13, 32; CrossRef (b) M. Antonietti, D. Kuang, B. Smarsly, and Y. Zhou, Angew. Chem., Int. Ed., 2004, 43, 4988. CrossRef
35. M. A. Gelesky, A. P. Umpierre, G. Machado, R. R. B. Correia, W. C. Magno, J. Morais, G. Ebeling, and J. Dupont, J. Amer. Chem. Soc., 2005, 127, 4588. CrossRef
36. (a) C. C. Cassol, A. P. Umpierre, G. Machado, S. I. Wolke, and J. Dupont, J. Amer. Chem. Soc., 2005, 127, 3298; CrossRef (b) M. T. Reetz and E. Westermann, Angew. Chem., Int. Ed., 2000, 39, 165. CrossRef
37. J. Huang, T. Jiang, B. Han, H. Gao, Y. Chang, G. Zhao, and W. Wu, Chem. Commun., 2003, 1654. CrossRef
38. G. S. Fonseca, A. P. Umpierre, P. F. P. Fichtner, S. R. Teixeira, and J. Dupont, Chem. Eur. J., 2003, 9, 3263. CrossRef
39. (a) Review: W. Miao and T. H. Chan, Acc. Chem. Res., 2006, 39, 897; CrossRef (b) W. Miao and T. H. Chan, Adv. Synth. Catal., 2006, 348, 1711. CrossRef
40. (a) H.-M. Guo, L.-F. Cun, L.-Z. Gong, A.-Q. Mi, and Y.-Z. Jiang, Chem. Commun., 2005, 1450; CrossRef (b) P. Kortrusz, I. Kmentova, B. Gotov, S. Toma, and E. Solcaniova, Chem. Commun., 2002, 2510. CrossRef
41. M. Nozawa, T. Akita, T. Hoshi, T. Suzuki, and H. Hagiwara, Synlett, 2007, 661. CrossRef
42. J. K. Park, P. Sreekanth, and B. M. Kim, Adv. Synth. Catal., 2004, 346, 49. CrossRef
43. H. Hagiwara, T. Okabe, T. Hoshi, and T. Suzuki, J. Mol. Cat. A: Chemical, 2004, 214, 167. CrossRef
44. Review: (a) M. Lombardo, A. Quintavalla, M. Chiarucci, and C. Trombini, Synlett, 2010, 1746; CrossRef (b) R. Giernoth, Angew. Chem. Int. Ed., 2010, 49, 2834; CrossRef (c) S.-G. Lee, Chem. Commun., 2006, 1049; CrossRef (d) J. H. Davis, Jr., Chem. Lett., 2004, 33, 1072. CrossRef
45. (a) H. Wakamatsu, Y. Saito, M. Masubuchi, and R. Fujita, Synlett, 2008, 1805; CrossRef (b) H. Clavier, N. Audic, M. Mauduit, and J. C. Guillemin, Chem. Commun., 2004, 2282; CrossRef (c) N. Audic, H. Clavier, M. Mauduit, and J. C. Guillemin, J. Amer. Chem. Soc., 2003, 125, 9248; CrossRef (d) Q. Yao and Y. Zhang, Angew. Chem. Int. Ed., 2003, 42, 3395. CrossRef
46. A. C. Cole, J. L. Jensen, I. Ntai, K. L. T. Tran, K. J. Weaver, D. C. Forbes, and J. H. Davis, Jr., J. Amer. Chem. Soc., 2002, 124, 5962. CrossRef
47. (a) K. Qiao and C. Yokoyama, Chem. Lett., 2004, 33, 472; CrossRef (b) K. Qiao and C. Yokoyama, Chem. Lett., 2004, 33, 808. CrossRef
48. S. Santra, A. Majee, and A. Hajra, Tetrahedron Lett., 2011, 52, 3825. CrossRef
49. R. G. Kalkhambkar, S. N. Waters, and K. K. Laali, Tetrahedron Lett., 2011, 52, 867. CrossRef
50. C. Paun, J. Barklie, P. Goodrich, H. Q. N. Gunaratne, A. McKeown, V. I. Parvulescu, and C. Hardacre, J. Mol. Cat. A: Chemical, 2007, 269, 64. CrossRef
51. W. Miao and T. H. Chan, Adv. Synth. Catal., 2006, 348, 1711. CrossRef
52. S. Luo, X. Mi, L. Zhang, S. Liu, H. Xu, and J.-P. Cheng, Angew. Chem. Int. Ed., 2006, 45, 3093. CrossRef
53. Y. Ishiwata and H. Togo, Tetrahedron, 2009, 65, 10720. CrossRef
54. S. Koguchi and T. Kitazume, Tetrahedron Lett., 2006, 47, 2797. CrossRef
55. (a) A. Fall, M. Sene, M. Gaye, G. Gomez, and Y. Fall, Tetrahedron Lett., 2010, 51, 4501; CrossRef (b) W. Qian, E. Jin, W. Bao, and Y. Zhang, Tetrahedron, 2006, 62, 556; CrossRef (c) X.-E. Wu, L. Ma, M.-X. Ding, and L.-X. Gao, Synlett, 2005, 607. CrossRef
56. (a) M. Jabbar, H. Shimakoshi, and Y. Hisaeda, Chem. Commun., 2007, 1653; CrossRef (b) H. Shimakoshi, T. Baba, Y. Iseki, I. Aritome, C. Adachi, and Y. Hisaeda, Chem. Commun., 2008, 2882. CrossRef
57. K. Qiao, H. Hagiwara, and C. Yokoyama, J. Mol. Cat. A: Chemical, 2005, 246, 65. CrossRef
58. H. Hagiwara, M. Sekifuji, T. Hoshi, K. Qiao, and C. Yokoyama, Synlett, 2007, 1320. CrossRef
59. H. Hagiwara, M. Sekifuji, T. Hoshi, T. Suzuki, B. Quanxi, K. Qiao, and C. Yokoyama, Synlett, 2008, 608. CrossRef
60. Z. Xua, H. Wana, J. Miao, M. Han, C. Yang, and G. Guana, J. Mol. Cat. A: Chemical, 2010, 332, 152. CrossRef
61. H. Zhi, C. Lu, Q. Zhang, and J. Luo, Chem. Commun., 2009, 2878. CrossRef
62. Review: (a) Y. Gu and G. Li, Adv. Synth. Catal., 2009, 351, 817; CrossRef (b) H. Hagiwara, Kagaku Kogyo, 2008, 59, 199.
63. (a) R. Lungwitz and S. Spange, J. Phys. Chem. C, 2008, 112, 19443; CrossRef (b) R. Lungwitz, T. Linder, J. Sundermeyer, I. Tkatchenko, and S. Spange, Chem. Commun., 2010, 46, 5903. CrossRef
64. (a) H. Hagiwara, Y. Sugawara, K. Isobe, T. Hoshi, and T. Suzuki, Org. Lett., 2004, 6, 2325; CrossRef (b) H. Hagiwara, Y. Sugawara, T. Hoshi, and T. Suzuki, Chem. Commun., 2005, 2942. CrossRef
65. (a) H. Hagiwara, K. H. Ko, T. Hoshi, and T. Suzuki, Chem. Commun., 2007, 2838; CrossRef (b) H. Hagiwara, H. Sasaki, N. Tsubokawa, T. Hoshi, T. Suzuki, T. Tsuda, and S. Kuwabata, Synlett, 2010, 1990. CrossRef
66. H. Hagiwara, T. Nakamura, T. Hoshi, and T. Suzuki, Green Chem., 2011, 13, 1133. CrossRef
67. H. Hagiwara, H. Sasaki, T. Hoshi, and T. Suzuki, Synlett, 2009, 643. CrossRef
68. (a) H. Hagiwara, N. Okunaka, T. Hoshi, and T. Suzuki, Synlett, 2008, 1813; CrossRef (b) H. Hagiwara, T. Nakamura, N. Okunaka, T. Hoshi, and T. Suzuki, Helv. Chim. Acta, 2010, 93, 175. CrossRef
69. H. Hagiwara, T. Kuroda, T. Hoshi, and T. Suzuki, Adv. Synth. Catal., 2010, 352, 909. CrossRef
70. H. Hagiwara, K. Sato, T. Hoshi, and T. Suzuki, Synlett, 2012, accepted for publication.
71. (a) T. Sasaki, M. Tada, C. Zhong, T. Kume, and Y. Iwasawa, J. Mol. Cat. A; Chemical, 2008, 279, 200. CrossRef
72. J.–F. Wei, J. Jiao, J.-J. Feng, J. Lv, X.-R. Zhang, X.-Y. Shi, and Z.-G. Chen, J. Org. Chem., 2009, 74, 6283. CrossRef
73. X. Shi, X. Han. W. Ma, J. Wei, J. Li, Q. Zhang, and Z. Chen, J. Mol. Cat. A: Chemical, 2011, 341, 57. CrossRef
74. L. Rodriguez-Perez, E. Teuma, A. Falqui, M. Gomez, and P. Serp, Chem. Commun., 2008, 4201. CrossRef
75. L.-L. Lou, K. Yu, F. Ding, W. Zhou, X. Peng, and S. Liu, Tetrahedron Lett., 2006, 47, 6513. CrossRef
76. (a) J.-P. Mikkola, P. Virtanen, H. Karhu, T. Salmi, and D. Yu. Murzin, Green Chem., 2006, 8, 197; CrossRef (b) J.-P. Mikkola, J. Aumo, D. Yu. Murzin, and T. Salmi, Catalysis Today, 2005, 105, 325. CrossRef
77. P. Hana, H. Zhang, X. Qiu, X. Ji, and L. Gao, J. Mol. Cat. A: Chemical, 2008, 295, 57. CrossRef
78. C. P. Mehnert, R. A. Cook, N. C. Dispenziere, and M. Afeworki, J. Amer. Chem. Soc., 2002, 124, 12932. CrossRef
79. C. P. Mehnert, E. J. Mozeleski, and R. A. Cook, Chem. Commun., 2002, 3010. CrossRef
80. (a) M. Jakuttis, A. Schonweiz, S. Werner, R. Franke, K.-D. Wiese, M. Haumann, and P. Wasserscheid, Angew. Chem. Int. Ed., 2011, 50, 4492; CrossRef (b) M. Haumann, K. Dentler, J. Joni, A. Riisager, and P. Wasserscheid, Adv. Synth. Catal., 2007, 349, 425; CrossRef (c) A. Riisager, R. Fehrmann, S. Flicker, R. van Hal, M. Haumann, and P. Wasserscheid, Angew. Chem. Int. Ed., 2005, 44, 815; CrossRef (d) A. Riisager, K. M. Eriksen, P. Wasserscheid, and R. Fehrmann, Catal. Lett., 2003, 90, 149; CrossRef (e) A. Riisager, P. Wasserscheid, R. van Hal, and R. Fehrmann, J. Catalysis, 2003, 219, 452. CrossRef
81. S. Werner, N. Szesni, A. Bittermann, M. J. Schneider, P. Harter, M. Haumann, and P. Wasserscheid, Applied Catalysis A: General, 2010, 377, 70. CrossRef
82. A. Riisager, B. Jørgensen, P. Wasserscheid, and R. Fehrmann, Chem. Commun., 2006, 994. CrossRef
83. J. Joni, M. Haumann, and P. Wasserscheid, Adv. Synth. Catal., 2009, 351, 423. CrossRef
84. Y. Taniguchi, Y. Makioka, and Y. Takaishi, Kagaku Kogyo, 2009, 60, 517.