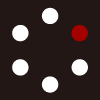
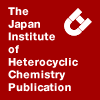
HETEROCYCLES
An International Journal for Reviews and Communications in Heterocyclic ChemistryWeb Edition ISSN: 1881-0942
Published online by The Japan Institute of Heterocyclic Chemistry
e-Journal
Full Text HTML
Received, 22nd November, 2011, Accepted, 27th December, 2011, Published online, 10th January, 2012.
DOI: 10.3987/COM-11-12397
■ Asymmetric Synthesis of (-)-Muricatacin’s Analogue (4S,5R)-5-Hydroxy-4-octadecanolide Exhibiting the Cytotoxicity against Esphageal Cancer Cells
Yow-Fu Tsai,* Chien-Cheng Huang, Shiau-Han Lin, Pei-Mei Su, Ying-Ju Chen, and Tzong-Yuan Wu
Department of Chemistry, Chung Yuan Christian University, 200 Chung Pei Rd., Chung Li 23023, Taiwan, R.O.C.
Abstract
An efficient and facile synthesis, in six steps and 50% overall yield from commercial D-(-)-lyxose as starting material via twice Wittig olefination and one-pot deisopropylidenation and intramolecular lactonization, of the enantiopure (4S,5R)-(+)-5-hydroxy-4-octadecanolide (2), analogue of (-)-muricatacin, and the cytotoxic activity against CE48T cell line (human esophageal carcinoma) are described and the biological activity is the first shown in the literature.Optically pure functionalized hydroxy-γ-lactones possess important biological activities and are important building blocks to synthesize a variety of natural products and biologically active compounds.1 Their synthesis has attracted much interst from organic synthetic chemists. Although many useful synthetic strategies have been reported,2 further investigations for facile and efficient entry to this type of compounds are still required.
(-)-Muricatacin (1, Figure 1), a functionalized 5-hydroxy-γ-lactone, was isolated from the seeds and bark of the tropical plant Annona muricata.3 The synthesis of (-)-muricatacin (1) and its congeners has attracted considerable attention of some organic synthetic laboratories4 over the past years due to possessing diverse biological activities.3,5 Furthermore, the stereochemistry at C4 and C5 position of (-)-muricatacin (1) and its congeners and the length of alkyl side chain which is more than thirteen carbons were reported to show no significant effect on biological activity based on the structure-activity relationship (SAR) studies.6 For the aforementioned reasons, (4S,5R)-(+)-5-hydroxy-4-octadecanolide (2, Figure 1), an unknown analogue of (-)-muricatacin, is chosen to be our synthetic target to develop an asymmetric synthetic methodology, adopted chiral pool strategy from the commercially avaible D-(-)-lyxose as the starting material, for efficiently preparing a variety of the enantiomerically pure hydroxy-γ-lactones.
The total synthesis of (4S,5R)-(+)-5-hydroxy-4-octadec-anolide (2) is outlined in Scheme 1. The synthesis of (4S,5R)-(+)-5-hydroxy-4-octadecanolide (2) started from the chemoselective isopropylidenation of D-lyxose with anhydrous acetone in the presence of catalytic amount of concentrated H2SO4 as catalyst at room temperature to furnish the 2,3-O-isopropylidene-α-D-lyxofuranose (3) as a single isolable anomer in 86% yield.7 Determination of the stereochemistry of isopropylidene 3 at anomeric carbon was compared to the literature results.7b The 1H and 13C NMR spectral data of compound 3 are well identical with the literature data as well as melting point and specific rotation [3: mp 81-83 oC; [α]28D +28.0 (c 1.2, H2O); lit.7b mp 80-82 oC; [α]21D +23.0 (CHCl3)]. Wittig olefination of 3 with dodecyl(triphenyl)phosphor nium bromide/KHMDS led to the corresponding enediol 4 as a sole isolable Z-stereoisomer in 81% yield. As for the stereochemistry of double bond in the 4, it was worked by the homodecouple technique of 1H NMR to determine the coupling constant (J = 11.1 Hz) of both vinyl protons and confirmed the assigned stereochemistry of double bond of the 4. In order to install the desired ester group and carbon chain by Wittig reaction, oxidative cleavage of the enediol 4 was achieved by treatment with NaIO4 in EtOH/H2O (2:1 (v/v)) to afford the aldehyde 5 as a syrup compound in 93% yield.8 Wittig olefination of the aldehyde 5 with the appropriate C2-ylide (Ph3P=CHCO2Et) gave the syrup dienester 6 as a ca. 3.2:1.0 mixture of E- and Z-stereoisomers (monitored by 1H NMR, 6-2E: δ 6.78 (dd, J = 15.6, 5.6 Hz, 1H); 6-2Z: δ 6.19 (dd, J = 12.0, 8.0 Hz, 1H)) in 82% yield. The diastereomer mixture without separation was subsequently subjected to hydrogenation under H2/Pd/C condition to obtain the saturated ester 7 as a white solid in 99% yield. The desired targeted compound (4S,5R)-(+)-5-hydroxy-4-octadecanolide (2) [[α]28D +10.0 (c 0.4, CHCl3)] was provided by means of one-pot deprotection and intramolecular lactonization of ester 7 with hydrochloric acid in THF/H2O (10:3 (v/v)) in 88% yield.9,10
After completing the asymmetric synthesis of hydroxyl lactone 2, the antitumor effect was examined. Furthermore, in order to understanding the effect of the hydroxyl group and lactone scaffold on biological activity, we also prepared the (4S,5R)-(+)-5-acetoxy-4-octadecanolide (2-AC) and optically pure (4S,5R)-octadecane-1,4,5-triol (2-Tol) (Figure 1). Herein, the cytotoxicities of lactone 2, 2-AC and 2-Tol against the CE48T cell line (human esophageal cancer cell) in vitro were evaluated by measuring the inhibition of cell proliferation. As shown in Figure 2, incubation of esophageal cancer cells with 2 and 2-AC for 24 h at a concentration range of 0.01-0.05 mg/mL caused a dose-dependent inhibition of cell proliferation. This result demonstrated both 2 and 2-AC exhibited cytotoxicity against esophageal cancer cells. The IC50 values were 20 and 25 µg/mL for 2 and 2-Ac, respectively. Besides, significant changes in the cell morphology were also observed after treatment with 2 and 2-AC for 24 h at 30 µg/mL. However, the triol 2-Tol did not show cytotoxicity against esophageal cancer cells. These results were consistent with previous studies6 on the cytotoxicity of the lacton derivatives toward cancer cells and demonstrated the core structure of lactone is maybe essential for inhibiting the growth of esophageal cancer cells.
In conclusion, the synthesis of (4S,5R)-(+)-5-hydroxy-4-octadecanolide (2) has been achieved in six steps from the chiral pool D-(-)-lyxose as the starting material in 50% overall yield and the cytotoxicity against esophageal cancer cells is the first shown in the literature for hydroxy-γ-lactone. Exploration of a facile and flexible methodology to prepare the other three stereoisomers of hydroxy-γ-lactone from the chiral pool D-(-)-lyxose and examination of their biological activities are in progress.
ACKNOWLEDGEMENTS
We gratefully acknowledge the financial support in part from the National Science Council, Taiwan (NSC 100-2119-M-033 -001), and (NSC 100-2120-M-033 -001).
References
1. (a) P. Kumar, S. V. Naidu, and P. Gupta, J. Org. Chem., 2005, 70, 2843; CrossRef (b) Y. Hu and R. C. D. Brown, Chem. Commun., 2005, 69, 5636; CrossRef (c) T. Yoshimitsu, T. Makino, and H. Nagaoka, J. Org. Chem., 2005, 69, 1993; CrossRef (d) H. Avedissain, S. C. Sinha, A. Yazhak, P. Neogi, S. C. Sinha, and E. Keinan, J. Org. Chem., 2000, 65, 6035; CrossRef (e) J. D. Ha and J. K. Cha, J. Am. Chem. Soc., 1999, 121, 10012; CrossRef (f) T. B. Towne and F. E. McDonald, J. Am. Chem. Soc., 1997, 119, 6022; CrossRef (g) A. D. Rodriguez, I. C. Pina, and C. L. Barness, J. Org. Chem., 1995, 60, 8096; CrossRef (h) H. C. Brown, S. K. Kulkarni, and U. S. Racherla, J. Org. Chem., 1994, 59, 365; CrossRef (i) M. M. Murata, M. B. M. de Azevedo, and A. E. Green, J. Org. Chem., 1993, 58, 7537 and references cited therein; CrossRef (j) K. Mori, In The Total Synthesis of Natural Products, ed. By J. Apsimon, John Wiley & Sons, 1992, vol. 9 and references cited therein; (k) M. Gasey, A. C. Manoge and P. J. Murphy, Tetrahedron Lett., 1992, 33, 965; CrossRef (l) T. Yokomatsu, Y. Yuasa, S. Kane, and S. Shibuya, Heterocycles, 1991, 32, 2315; CrossRef (m) R. S. Ward, Tetrahedron, 1990, 46, 5029; CrossRef (n) J. Arija, J. Font, and R. M. Ortuno, Tetrahedron, 1990, 46, 1931; CrossRef (o) S. Tsuboi, J. Sakamoto, T. Sakai, and M. Utaka, Chem. Lett., 1989, 1427; CrossRef (p) K. Tomioka, Y. S. Cho, F. Sato, and K. Koga, J. Org. Chem., 1988, 53, 4094; CrossRef (q) R. M. Ortuno, J. Bigorra, and J. Font, Tetrahedron, 1988, 44, 5139; CrossRef (r) S. Hanessian, Total Synthesis of Natural Products: The Chiron Approach, Pergamon Press, 1986. and references cited therein; (s) S. Hannessian, P. J. Murray, and S. P. Sahoo, Tetrahedron Lett., 1985, 26, 5627. CrossRef
2. (a) M. He, A. Lei, and X. Zhang, Tetrahedron Lett., 2005, 46, 1823; CrossRef (b) F. Schleth and A. Studer, Angew. Chem. Int. Ed., 2004, 43, 313; CrossRef (c) T. Benincori, S. Rizzo, T. Pilati, A. Ponti, M. Sada, E. Pagliarini, S. Ratti, C. Giuseppe, L. de Ferra, and F. Sannicoló, Tetrahedron: Asymmetry, 2004, 15, 2289; CrossRef (d) F. Schleth, T. Vogler, K. Harms, and A. Studer, Chem. Eur. J., 2004, 10, 4171; CrossRef (e) M. Amador, X. Ariza, J. Garcia, and J. Ortiz, J. Org. Chem., 2004, 69, 8172; CrossRef (f) A. Samarat, H. Amri, and Y. Landais, Tetrahedron, 2004, 60, 8949; CrossRef (g) X. Lu and Q. Zhang, Pure Appl. Chem., 2001, 73, 247; CrossRef (h) B. El Ali and H. Alper, Synlett, 2000, 161; CrossRef (i) S. Marcaccini, R. Pepino, C. F. Marcos, C. Polo, and T. J. Torroba, Heterocycl. Chem., 2000, 37, 1501; CrossRef (j) S. V. Ley, L. R. Cox, and G. Meek, Chem. Rev., 1996, 96, 423; CrossRef (k) M. P. Doyle, Aldrichimica Acta, 1996, 29, 3; (l) R. Bossio, S. Marcaccini, and R. Pepino, Liebigs Ann. Chem., 1994, 5, 527; CrossRef (m) R. Bossio, S. Marcaccini, R. Pepino, and T. Torroba, Synthesis, 1993, 783; CrossRef (n) Y. S. Rao, Chem. Rev., 1976, 76, 625. CrossRef
3. M. J. Rieser, J. F. Kozlowski, K. V. Wood, and J. L. McLaughlin, Tetrahedron Lett., 1991, 32, 1137. CrossRef
4. P. Ghosal, V. Kumar, and A. K. Shaw, Carbohydr. Res., 2010, 345, 41 and references cited therein. CrossRef
5. (a) B. Figadère, J.-C. Harmange, A. Laurens, and A. Cavé, Tetrahedron Lett., 1991, 32, 7539; CrossRef (b) M. J. Rieser, J. F. Kozlowski, K. V. Wood, and J. L. McLaughlin, Tetrahedron Lett., 1991, 32, 1137. CrossRef
6. A. Cave, C. Chaboche, B. Figadere, J. C. Harmange, A. Laurens, J. F. Peyrat, M. Pichon, M. Szlosek, J. Cotte-Laffite, and A. M. Quero, Eur. J. Med. Chem., 1997, 32, 617. CrossRef
7. (a) M. S. M. Pearson, N. Floquet, C. Bello, P. Vogel, R. Plantier-Royon, J. Szymoniak, P. Bertus, and J.-B. Behr, Bioorg. Med. Chem., 2009, 17, 8020; CrossRef (b) J. Barbat, J. Gelas, and D. Horton, Carbohydr. Res., 1991, 219, 115. CrossRef
8. (a) W. H. Kim, J.-A. Kang, H.-R. Lee, A.-Y. Park, P. Chun, B. Lee, J. Kim, J.-A. Kim, L. S. Jeong, and H. R. Moon, Carbohydr. Res. 2009, 344, 2317; CrossRef (b) S.-H. Yoon, H.-S. Moon, S.-K. Hwang, S. R. Choia, and S.-K. Kang, Bioorg. Med. Chem., 1998, 6, 1043. CrossRef
9. A. Gypser, M. Peterek, and H.-D. Scharf, J. Chem. Soc., Perkin Trans. 1, 1997, 1013. CrossRef
10. (S)-5-((1R)-1-hydroxytetradecyl)-dihydrofuran-2(3H)-one (2): mp 48-50 °C; [α]28D +10.0 (c 0.4, CHCl3); FT-IR (neat) cm-1: 3539, 3413, 2954, 2917, 2848, 2360, 2341, 1757, 1460, 1189 cm-1; 1H NMR (300 MHz, CDCl3): δ 4.44 (td, J = 7.2 Hz, 1H), 4.00-3.90 (m, 1H), 2.70-2.40 (m, 2H), 2.40-2.00. (m, 2H), 1.86 (s, 1H), 1.70-1.40 (m, 2H), 1.26 (bs, 22H), 0.88 (t, J = 6.0 Hz, 3H); 13C NMR (100 MHz, CDCl3): δ 177.6 (C), 82.9 (CH), 71.3 (CH), 31.9 (CH2), 31.8 (CH2), 29.7 (CH2), 29.6 (CH2), 29.6 (CH2), 29.5 (CH2), 29.5 (CH2), 29.5 (CH2) 29.32 (CH2), 28.7 (CH2), 25.6 (CH2), 22.7 (CH2), 21.0 (CH2), 14.1 (CH3); HRMS-ESI [M + Na]+ Calcd for C18H34O3Na 321.2400, Found 321.2400.