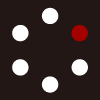
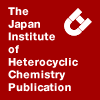
HETEROCYCLES
An International Journal for Reviews and Communications in Heterocyclic ChemistryWeb Edition ISSN: 1881-0942
Published online by The Japan Institute of Heterocyclic Chemistry
e-Journal
Full Text HTML
Received, 24th November, 2011, Accepted, 15th December, 2011, Published online, 22nd December, 2011.
DOI: 10.3987/COM-11-12398
■ Novel Rearrangements of a 8aH-Pyrido[1,2-d]thieno[2’,3’-b][1,4]thiazepine Derivative
Akikazu Kakehi,* Kennosuke Itoh, Hiroyuki Suga, Yuuki Ohkubo, Ayaka Kobayashi, and Takashi Nishi
Department of Chemistry and Material Engineering, Faculty of Engineering, Shinshu University, 4-17-1 Wakasato, Nagano 380-8553, Japan
Abstract
The reaction of 5-ethoxycarbonyl-3-[1-(4-methylpyridinio)]-4-phenythiopene-2-thiolate with dimethyl acetylenedicarboxylate in refluxing chloroform or tetrahydrofuran afforded the corresponding 10H-pyrrolo[1,2-d]thieno[2’,3’-b][1,4]thiazocines or the 6,8-dithia-2-azatetracyclo[7.3.2.02,10.03,7]-tetradeca-Δ3,7,4,11,13-tetraene derivative. Also, the thermal conversion from the former product to dimethyl (Z)- and (E)-2-[thieno[2’,3’:2,3][1,4]thiazino[4,5-a]pyrrol-8-ylidene]succinates was confirmed.In our recent paper2 we reported the formation of an interesting byproduct, dimethyl 2-[thieno-[2’,3’:2,3][1,4]thiazino[4,5-a]pyrrol-8-ylidene]succinate such as C (see Figure 1), in the one-pot synthesis of thieno[3,2-d]thiazole derivatives by the reactions of some 3-(1-pyridinio)thiophene- 2-thiolates with dimethyl acetylenedicarboxylate (DMAD) in refluxing xylene. We were particularly interested in the presence of the pyrrole ring in this byproduct because a ring contraction rearrangement from the pyridine ring to the pyrrole one is involved in this reaction and we already observed the formation of similar ring transformation products, e.g., 5-thia-2,3-diazatricyclo-[4.3.2.02,7]undeca-3,8,10-triene derivatives such as F in the reactions of pyridinium 1-(arylthio-carbonyl)aminides with DMAD.3 From these observations we first assumed the following rough mechanistic sequence: 8aH-pyrido[1,2-d]thieno[2’,3’-b][1,4]thiazepine (A) → 6,8-dithia-2-azatetracyclo[7.3.2.02,10.03,7]tetradeca-Δ3,7,4,11,13-tetraene (B)→8H-thieno[2’,3’:2,3][1,4]-thiazino[4,5-a]pyrrole (C) and examined the isolation or the detection of the intermediates such as B or its thiophene-free analogue in the reactions of various pyridinium 1-(2-thioxo)ethylides and 3-(1-pyridinio)thiophene-2-thiolates with DMAD (2) under milder conditions. Almost all reactions which we reinvestigated under this assumption afforded only already established products such as thiazepines (A) or their intramolecular Diels-Alder type adducts (D). However, the reaction of one substrate, 5-ethoxycarbonyl-3-[1-(4-methylpyridinio)]-4-phenylthiophene-2-thiolate, with 2 gave unexpected 10H-pyrrolo[1,2-d]thieno[2’,3’-b][1,4]thiazocines (E) together with the expected 6,8-dithia-2-azatetra-cyclo[7.3.2.02,10.03,7]tetradeca-Δ3,7,4,11,13-tetraene (B). In this communication we report novel rearrangement reactions from the 8aH-pyrido[1,2-d]thieno[2’,3’-b][1,4]thiazepine derivative to the corresponding rearranged products B and E and the thermal transformation from E to the corresponding dimethyl 8H-pyrrolo[2,1-c]thieno[2’,3’-b][1,4]thiazin-8-ylidenesuccinate (C).
As described above, the reactions of almost all pyridinium 1-(2-thioxo)ethylides and 3-(1-pyridinio)-thiophen-2-thiolates with 2 under various reaction conditions afforded only the corresponding type A and/or D adducts, but not the type B product. For example, the reactions of 3-(1-pyridinio)thiophene-2-thiolates (1a–c), which formed the corresponding type C products in refluxing xylene,2 with 2 in refluxing chloroform or tetrahydrofuran (THF) afforded only intramolecular Diels-Alder type adducts 3a–c4 and any other significant products could not be obtained (Scheme 1). So, we focused our attention on some other substrates whose yields for adducts such as 3 in our previous reactions which were carried out in refluxing chloroform were very low.5 After some elaboration we found that one substrate among them, 5-ethoxycarbonyl-3-[1-(4-methylpyridinio)]-4-phenylthiophene-2-thiolate (1d), smoothly reacted with 2 in refluxing chloroform for 10 h to produce the unexpected 2-ethyl 9,10-dimethyl 7-methyl-3-phenyl-10H-pyrrolo[1,2-d]thieno[2’,3’-b][1,4]thiazocine-2,9,10-tricarboxylates (4d+5d)6 as a diastereomeric mixture7 in a 85% yield, while similar reactions of 1d
in refluxing THF gave the expected adduct 6d8 in a 38% yield. On the other hand, the reactions of 1d with 2 in refluxing methylene chloride or benzene afforded the mixtures of 4d+5d (60%) and 6d (30%) or 3d (13%), 4d+5d (56%) and 6d (11%) respectively. Our attempts to separate the mixture of 4d+5d by column chromatography were unsuccessful. This and the fact that the mixtures of 4d+5d obtained under different conditions had almost the same isomeric ratio (ca. 85:15) strongly suggests the presence of an equilibrium between them. The structures of products 3a–d could be determined by comparison with some data of known compounds and an authentic sample (3d)5 and the structure of 6d was also decided by the similarity of the chemical shifts and the coupling constants for the skeletal protons in the 1H-NMR spectrum with those of dimethyl 4-aryl-5-thia-2,3-diazatricyclo[4.3.2.02,7]undeca-3,8,10-triene-6,11-dicarboxylate derivatives.3 On the other hand, we could recognize that mixture 4d+5d is a 1:1 adduct between pyridinium betaine (1d) and 2 from
its elementary analysis and has a trisubstituted aromatic pyrrole structure and each one uncoupled vinyl and methine proton from its 1H-NMR spectral analysis, but further information for these structures was not obtained. Eventually, the X-ray analysis of the crystals obtained from the recrystallization of 4d+5d made the structure for the major product 4d clear.9 The ORTEP drawing for 4d is shown in Figure 2.10
We next examined the formation of a dimethyl 8H-pyrrolo[2,1-c]thieno[2’,3’-b][1,4]thiazin-8-ylidenesuccinate derivative such as C. Contrary to our first assumption, compound 6d did not give the corresponding 1,4-thiazine derivative 7 in refluxing xylene for 2 d and also was not converted to 1,4-thiazocines 4d+5d in refluxing chloroform for 10 h. On the other hand, mixture 4d+5d in boiling xylene for 2 d provided the expected products 711 in 12 and 20% yield respectively as the (Z)- and (E)-isomers in relation to the 8-exo methylene group, together with pyrrolium betaine 8 (34%).12 (Scheme 2) Interestingly, product 8 gradually changed to (Z)-7 and (E)-7 during the separation and purification processes. Furthermore, (Z)-7 was also unstable and isomerized to (E)-7 even while being kept at room temperature.
The structures of (Z)-7 and (E)-7 were determined by comparison of their 1H-NMR spectra with known compounds,2 and the distinction between the Z- and E-isomers was assumed by considering their stability and the presence of the geminal coupling constant of the methylene group attached at the 8(1)-position of (Z)-7. This structural assumption for isomers was also confirmed by their X-ray analyses and the ORTEP drawings10 for compound (Z)-7 and (E)-7 are shown in Figures 3 and 4.13 Similarly, the structure of 8 was assigned by the presence of a trisubstituted pyrrole ring and the 2 vinyl protons in its 1H-NMR spectral analysis and by the intermediacy as a precursor to final products (Z)-7 and (E)-7.
Possible mechanisms for these reactions are shown in Scheme 3. In particular, the transformation from [1,4]thiazocines 4d+5d to final products (Z)-7 and (E)-7 can be readily interpreted by adding
another intermediate, 8-[1,2-bis(methoxycarbonyl)vinyl-8H-pyrrolo[2,1-c]thieno[2’,3’-b][1,4]thiazine (11d), which is formed from the cyclization in another resonance structure 8d’ of pyrrolium betaine 8d. However, the reaction mechanism from thiazepine 9d14 to 4d+5d is unclear15 because the promising route from 6d, which has a 1,4-dihydropyrrole ring in that skeleton, to 4d+5d was refused. The reason why different products 3d, 4d+5d, and 6d are formed from the same substrate 1d and reagent 2 dependent upon the conditions employed is unclear, but the temperature dependency for the formation of 3d in benzene5 or type F compounds in chloroform3 strongly suggests larger contribution of the reaction temperature than the solvent effect. The scope and limitation for these rearrangements are now in progress.
References
1. Preparation of new nitrogen-bridged heterocycles. 75. For 74 of this series, see A. Kakehi, K. Itoh, H. Suga, N. Yamaguchi, T. Abe, Y. Yuda, and H. Katsumata, Heterocycles, 2012, 84, 753. CrossRef
2. A. Kakehi, S. Ito, H. Suga, Y. Okumura, and T. Nishi, Heterocycles, 2010, 81, 175. CrossRef
3. A. Kakehi, S. Ito, F. Ishida, and Y. Tominaga, J. Org. Chem., 1997, 62, 7788. CrossRef
4. 3a, colorless needles, mp195−196 °C. IR (KBr): 1628, 1713, 1730 cm-1. 1H-NMR δ (CDCl3): 2.40 (3H, s, 3-Me), 2.45 (3H, s, Ac), 2.77 (1H, ddd, J = 7.1, 5.9, 2.2 Hz, 11-H), 3.56 (1H, dd, J = 7.1, 1.4 (W-shaped coupling with the 10-H) Hz, 11-H), 3.72 and 3.74 (each 3H, s, CO3Me), 4.16 (1H, dd, J = 6.6, 1.4 (W-shaped coupling with the 14-H), 1.2 Hz, 7-H), 6.09 (1H, ddd, J = 8.3, 6.6, 2.2 Hz, 13-H), 6.29 (1H, ddd, J = 8.3, 5.9, 1.2 Hz, 12-H). Anal. Calcd for C18H17NO5S2: Calcd C, 55.23; H, 4.38; N, 3.58. Found C, 55.18; H, 4.41; N, 3.50. 3b, colorless needles, mp152−154 °C. IR(KBr): 1630, 1721, 1742 cm-1. Anal. Calcd for C23H19NO5S2: Calcd C, 60.91; H, 4.22; N, 3.09. Found C, 60.81; H, 4.36; N, 3.05. 3c, colorless needles, mp178−181 °C. IR(KBr): 1636, 1725, 1742 cm-1. Anal. Calcd for C23H19NO5S2: Calcd C, 60.91; H, 4.22; N, 3.09. Found C, 60.96; H, 4.26; N, 3.00.
5. A. Kakehi, S. Ito, M. Mitani, and M. Kanaoka, Bull. Chem. Soc. Jpn., 1994, 67, 1646. CrossRef
6. 4d+5d, pale yellow prisms, mp 129−132 °C. IR (KBr): 1653, 1699, 1734 cm-1. Anal. Calcd for C25H23NO6S2: Calcd C, 60.35; H, 4.66; N, 2.82. Found C, 60.29; H, 4.75; N, 2.78. 1H-NMR δ (CDCl3) 4d: 1.16 (3H, t, J = 7.1 Hz, OCH2CH3), 2.07 (3H, s, 7-Me), 3.75 and 3.80 (each 3H, s, CO2Me), 4.10−4.20 (2H, m, OCH2CH3), 5.35 (1H, s, 10-H), 5.78 (1H, d, J = 2.8 Hz, 6-H), 6.14 (1H, d, J = 2.8 Hz, 5-H), 6.88−6.94 (2H, m, Ph-H), 7.15−7.22 (2H, m, Ph-H), 7.23−7.28 (1H, m, Ph-H), 7.92 (1H, s, 8-H). 5d: 1.16 (3H, t, J = 7.1 Hz), 1.98 (3H, s, 7-Me), 3.49 and 3.86 (each 3H, s, CO2Me), 4.10−4.20 (2H, m, OCH2CH3), 5.21 (1H, s, 10-H), 5.61 (1H, d, J = 2.8 Hz, 6-H), 6.07 (1H, d, J = 2.8 Hz, 5-H), 6.88−6.94 (2H, m, Ph-H), 7.15−7.22 (2H, m, Ph-H), 7.23−7.28 (1H, m, Ph-H), 7.87 (1H, s, 8-H).
7. This molecule has only one asymmetric carbon (C(10)) and usually exists as a pair of enantiomers. However, this rigid tricyclic structure, which is further promoted by the 3-phenyl group, strongly prevents the rotation of the C(3a)-N(4) bond and leads to an additional asymmetry.
8. 6d, colorless needles, mp 156−158 °C. IR (KBr): 1437, 1719 cm-1. 1H-NMR δ (CDCl3): 1.14 (3H, t, J = 7.1 Hz, OCH2CH3), 1.68 (3H, s, 12-Me), 3.21 (1H, d, J = 4.6 Hz, 1-H), 3.69 and 3.79 (each 3H, s, CO2Me), 4.12 and 4.14 (each 1H, q, J = 7.1 Hz, OCH2CH3), 4.23 (1H, d, J = 2.0 Hz, 10-H), 5.64 (1H, br s, 11-H), 7.33−7.42 (5H, m, Ph-H), 7.44 (1H, d, J = 4.6 Hz, 13-H). 13C-NMR δ (CDCl3): 14.10, 15.05, 47.01, 52.00, 53.27, 60.75, 64.82, 66.64, 122.24, 125.53, 126.88, 127.76, 127.91, 128.24, 129.41, 134.14, 139.24, 142.49, 145.36, 151.44, 161.23, 164.16, 168.61. Anal. Calcd for C25H23NO6S2: Calcd C, 60.35; H, 4.66; N, 2.82. Found C, 60.28; H, 4.81; N, 2.73.
9. Crystal data of 4d: C25H23NO6S2; M = 497.58; monoclinic, space group P21/n (#14), Z = 4 with a = 12.02 (2) Å, b = 12.38 (3) Å, c = 16.42 (2) Å, β = 95.99o (13); V = 2430.1 (72) Å3, and Dcalc. = 1.360 g/cm3. All calculations were performed using CrystalStructure.16 The structure was solved by a direct method (SIR92).17 The non-hydrogen atoms were refined anisotropically, and the hydrogen atoms were attached at the idealized position and not refined. The final R- and Rw-factors after full-matrix least-squares refinements were 0.063 and 0.045 for 3175 (I>2.00s(I)) observed reflections, respectively.
10. C. K. Johnson, "ORTEO II, Report ORNL-5138," Oak Ridge National Laboratory, Oak Ridge, Tennessee, 1976.
11. (Z)-7 (this compound could not be obtained in a pure state because of the ready transformation to (E)-7 isomer), pale yellow prisms. 1H-NMR δ (CDCl3): 1.15 (3H, t, J = 7.1 Hz, OCH2CH3), 1.96 (3H, s, 7-Me), 3.51 (1H, br d, J = 18.8 Hz), 3.69 (1H, br d, J = 18.8 Hz), 3.69 and 3.87 (each 3H, s, CO2Me), 4.14 and 4.16 (each 1H, q, J = 7.1 Hz, OCH2CH3), 5.94 (1H, d, J = 2.9 Hz, 6-H), 6.11 (1H, d, J = 2.9 Hz, 5-H), 7.41−7.50 (5H, m, Ph-H). (E)-7, pale yellow prisms, mp 181−182 °C. IR (KBr): 1414, 1688, 1746 cm-1. 1H-NMR δ (CDCl3): 1.14 (3H, t, J = 7.1 Hz, OCH2CH3), 1.92 (3H, s, 7-Me), 3.71 and 3.76 (each 3H, s, CO2Me), 3.76 (2H, s, CH2), 4.15 (2H, q, J = 7.1 Hz, OCH2CH3), 5.89 (1H, d, J = 2.9 Hz, 6-H), 6.07 (1H, d, J = 2.9 Hz, 5-H), 7.31−7.49 (5H, m, Ph-H).
12. 8 (this compound could not be obtained in a pure state because of the transformation to (Z)-7 and (E)-7 isomers), colorless powder. 1H-NMR δ (CDCl3): 1.12 (3H, t, J = 7.1 Hz, OCH2CH3), 1.95 (3H, s, 3-Me), 3.60 and 3.73 (each 3H, s, CO2Me), 4.12 and 4.13 (each 1H, q, J = 7.1 Hz, OCH2CH3), 5.74 (1H, d, J = 2.9 Hz, 4-H), 5.97 (1H, d, J = 2.9 Hz, 5-H), 6.45 and 6.50 (each 1H, s, vinyl-H), 7.35−7.53 (5H, br s, Ph-H).
13. Crystal data of (Z)-7: C25H23NO6S2; M = 497.58; monoclinic, space group P21/c (#14), Z = 4 with a = 9.83 (3) Å, b = 10.22 (4) Å, c = 24.60 (2) Å, β = 96.03° (16); V = 2455.9 (126) Å3, and Dcalc. = 1.346 g/cm3. All calculations were performed using CrystalStructure.16 The structure was solved by a direct method (SIR92).17 The non-hydrogen atoms were refined anisotropically, and the hydrogen atoms were attached at the idealized position and not refined. The final R- and Rw-factors after full-matrix least-squares refinements were 0.118 and 0.090 for 2704 (I>2.00s(I)) observed reflections, respectively. Crystal data of (E)-7: C25H23NO6S2; M = 497.58; triclinic, space group P-1 (#2), Z = 2 with a = 10.26 (3) Å, b = 12.64 (2) Å, c = 10.113 (18) Å, α = 113.26 (12); β = 98.6° (2); γ = 85.21 (19); V = 1190.9 (40) Å3, and Dcalc. = 1.388 g/cm3. All calculations were performed using CrystalStructure.16 The structure was solved by a direct method (SIR92).17 The non-hydrogen atoms were refined anisotropically, and the hydrogen atoms were attached at the idealized position and not refined. The final R- and Rw-factors after full-matrix least-squares refinements were 0.063 and 0.041 for 2632 (I>2.00s(I)) observed reflections, respectively.
14. A. Kakehi, S. Ito, J. Hakui, Bull. Chem. Soc. Jpn., 1993, 66, 3475. CrossRef
15. The transformation from thiazepine 9d to the corresponding thiazocines 4d+5d was confirmed in CDCl3 at 50−55 °C for 5 h, while that from adduct 3d to thiazepine 9d could not be detected under the same conditions even for 3 d.
16. CrystalStructure 3.8: Crystal Structure Analysis Package, Rigaku and Rigaku/MSC (2000−2006). 9009 New Trails Dr. The Woodlands TX 77381 USA.
17. SIR92: A. Altmare, G. Cascarano, C. Giacovazzo, A. Guagliardi, M. Burla, G. Polridori, and M. Camalli, J. Appl. Cryst., 1994, 27, 435 CrossRef