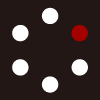
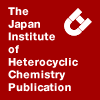
HETEROCYCLES
An International Journal for Reviews and Communications in Heterocyclic ChemistryWeb Edition ISSN: 1881-0942
Published online by The Japan Institute of Heterocyclic Chemistry
e-Journal
Full Text HTML
Received, 24th November, 2011, Accepted, 26th December, 2011, Published online, 10th January, 2012.
DOI: 10.3987/COM-11-12399
■ Synthesis of 3-Cyanopyrazoles from 3-Trifluoromethyl-pyrazoles via Direct Ammonolysis Reaction
Tao Yan, Yanhong Chen, Jia Wang, Yuyuan Xie, and Chunhao Yang*
State Key Laboratory of Drug Research, Shanghai Institute of Materia Medica, SIBS, Chinese Academy of Science, 555 Zu Chong Zhi Road, Shanghai 201203, China
Abstract
A simple and green method to prepare 3-cyanopyrazoles in aqueous ammonia from easy-obtained 3-trifluoromethylpyrazoles was explored. Most substrates got acceptable yields. The hydrogen position of N1-H on cyanopyrazoles was assigned by X-ray crystal structure analysis.3-Trifluoromethylpyrazoles play an important role in medicinal chemistry and agrochemicals and have been well studied.1-12 However, 3-cyanopyrazoles have not been studied adequately. Cyano group is very important in medicinal chemistry as a functional group13 and could be converted to amino methyl, amide, thioamide, and many other functional groups. Although 3-Cyanopyrazoles also serve as useful building blocks14 and important insecticides such as Fipronil, only few reports described the synthesis of N1-unsubstituted 3-cyanopyrazoles.15, 16 These methods have many disadvantages: starting materials are not easy obtained such as (Z)-3-p-tolylsulfinylacrylonitriles, N-phenylsydnone, and toxic agents as chlorosulphonyl isocyanate, POCl3 are inevitable, and the procedure is tedious. As the facile synthesis and bioevaluation of cyanopyrazoles are still limited, the development of novel useful methods for their construction remains an urgent task. As far as we know some papers/patents disclosed the formation of cyano products through ammonolysis of trifluoromethyl derivatives, including 2-trifluoromethy1imidazoles,17-19 4-trifluoromethy1imidazoles,20 3-(trifluoromethyl)-1H-pyrazolo[3,4-b]pyridine,21 and trifluoromethy1benzene.22, 23 The ammonolysis of 3-trifluoromethyl-pyrazoles has not been explored. Herein we report the synthesis of 3-cyanopyrazoles from 3-trifluoro-methylpyrazoles in aqueous ammonia without using any toxic reagent.
A series of 3-trifluoromethylpyrazoles were easily prepared by reported procedures.24-29 At first, we chose the ammonolysis of 5-(furan-2-yl)-3-(trifluoromethyl)-1H-pyrazole 1a as a model experiment (Table 1) to optimize the reaction.
Initially, the effect of temperature was examined. Ammonolysis of 1a gave the product only in the conversion of 41% at 40 oC (entry 1). Elevating the temperature to 60 oC could improve the yield and the conversion to 55% and 94% respectively (entry 2). But further boosting the temperature to 100 oC, no cyanopyrazole was detected because the desired product was hydrolyzed to corresponding amide or acid (entry 3). Secondly, pH value of the reaction solution was considered. Ammonium chloride and ammonia were used as buffer, but it turned to be useless (entries 4 and 5).
Then, the concentrations of aqueous ammonia were taken into consideration. The yields and the conversions were similar when the volume concentration of ammonia ranged from 20 to 12% (entries 7- 10). To our pleasure, the yield increased to 70% when the volume ratio of ammonia to water was 1: 2 (entry 11). Surprisingly the yield decreased dramatically to 6% at the more diluted solution (entry 12).
Next, reaction time was investigated. Reducing the reaction time from 24 h (entry 11) to 12 h (entry 13), the same yield was obtained , while prolonging the time to 36 h the yield decreased to 39% (entry 14).
Finally, the effect of organic solvent was explored. We expected that yield and the conversion could be increased due to the improved solubility of 1a in organic solvent. However, adding 0.5 mL of THF, Et2O or EtOH into the reaction system decreased the yield slightly (entries 15-17).
Thus, the optimized procedure was as follows: 3-trifluoromethylpyrazoles in 8% aqueous ammonia was heated for 12 h at 60 °C in a sealed tube. Under the optimized condition, we investigated the scope and the limitations of the reaction employing a variety of easily prepared 3-trifluoromethyl-pyrazoles, the results were summarized in Table 2.
Considering the diversity of substrates, we chose 4- or 5-substituted 3-trifluoromethylpyrazoles and saturated cyclic alkyl fused 3-trifluoromethylpyrazole to explore the reaction. Functional groups at position 5 included heterocycles such as 2-furyl and 2-thienyl, phenyl, substituted phenyl with electron-donating group or electron-withdrawing group. Most substrates got moderate yields (20% to 69%) except for 2i and 2n. To our surprise 2i could be obtained almost quantitatively (entry 9). As described above, 1a could be converted to 2a with good yield and conversion (entry 1). However, when 2-furyl group was replaced by 2-thienyl group, the yield and conversion decreased dramatically. To our surprise, the yield of 2b could be increased greatly to 80% when the volume ratio of ammonia to water was adjusted to 1:4 (entry 2), under this condition, the yield of 2m was also improved obviously from 48 to 60%. Although the yield of 2n is very low, this approach could provide estrogenic derivative rapidly for drug screening. From Table 2, no obvious rules for ammonolysis could be drawn out and the electrical properties of functional groups had little effect on the yield (entry 5 vs entry 11, entry 6 vs entry 12). The concentration of the ammonia has great effect on the yield for different compounds.
It was well known that two tautomers existed for pyrazoles. For our substrates, the tautomer was was demonstrated by X-ray crystal structure of 2c (Figure 1). This result was similar to the tautomerism of 3-trifluoromethylpyrazoles.30 Kimoto and Cohen proposed the corresponding mechanism in their preparation of 2-cyanoimidazoles17, 19. So, we reasonably proposed the mechanism of this reaction as Scheme 1.
An eco-friendly route to prepare 3-cyanopyrazoles through ammonolysis of 3-trifluoromethyl-pyrazoles was explored. Most of functional 3-trifluoromethylpyrazoles afforded 3-cyanopyrazoles in acceptable yields. Electrical properties seem no effect on this reaction, while the concentration of ammonia makes great sense. Although the yields of some substrates are moderate, this method provides a rapid and simple approach to 3-cyanopyrazoles as building blocks or bioactive compounds for drug screening. The hydrogen on N1 was assigned by X-ray single crystal diffraction.
EXPERIMENTAL
All solvents and others reagents were used without further purification as acquired from commercial sources, the concenteation of commercial ammonia solution is 25%. 1H and 13C NMR spectra were recorded on Varian Mercury-300 and Varian Mercury-500 spectrometers. IR spectra were recorded on FT-IR NICOLET 6700 spectrometer. HRMS spectra were performed on a Finnigan MAT 95 spectrometer. Melting points were measured by Büchi 510 melting point apparatus and were uncorrected. HPLC detections were done on an Agilent 1100 instrument equipped with a DAD detector, using a C18 reverse phase column. Conditions were as follows: flow rate, 1.0 mL/min; mobile phase, H2O : MeCN=65 : 35; column temperature, 25 °C, wavelength, 260 nm.
A typical experimental procedure for 3-cyanopyrazoles (2a–2n): In a sealed tube, 3-trifluoromethylpyrazole (100 mg) and prepared aqueous ammonia (15 mL) was stirred at 60 °C for 12 h, then the mixture was cooled to rt and extracted with 10 mL AcOEt each for three times. The combined organic phase was dried with anhydrous Na2SO4, and concentrated in vacuum. The crude product was purified through column chromatography on silica gel to give white powder. HPLC detection was performed with reaction solution.
5-(Furan-2-yl)-1H-pyrazole-3-carbonitrile (2a)
White powder, mp 108–110 °C. 1H NMR (CDCl3, 300 Hz): δ 6.50– 6.57 (m, 1H), 6.72 (d, 1H, J = 3.4 Hz), 6.84 (s, 1H), 7.51–7.60 (m, 1H), 11.93 (brs, 1H). 13C NMR (DMSO-d6, 126 MHz): δ 143.95, 142.79, 135.36, 124.63, 114.57, 112.05, 108.76, 106.803. IR (KBr) 3426, 2919, 2254, 1014, 565 cm-1. MS (EI) m/z (%): 159 (M+, 100). HRMS (EI) calcd. for C8H5N3O, 159.0433; found 159.0436.
5-Phenyl-1H-pyrazole-3-carbonitrile (2c).
White powder, mp 108–110 °C. 1H NMR (CDCl3, 300Hz): δ 6.73 (s, 1H), 7.45–7.50 (m, 3H), 7.57 (dd, 2H, J = 1.8, 7.3 Hz), 11.10 (brs, 1H). 13C NMR (DMSO-d6, 126 MHz): δ 144.34, 129.67, 129.65, 127.96, 126.05, 125.21, 115.32, 108.39. IR (KBr) 3434, 3143, 3004, 2246, 1567, 1465, 1014, 761, 688, 516 cm-1. MS (EI) m/z (%): 169 (M+, 100). HRMS (EI) calcd. for C10H7N3, 169.0640; found 169.0644.
4-Phenyl-1H-pyrazole-3-carbonitrile (2d).
White powder, mp 162–163 °C. 1H NMR (CDCl3, 300Hz): δ 7.88 (s, 1H), 7.65 (dd, 2H, J = 8.8, 1.5), 7.38-7.49 (m, 3H). 13C NMR (DMSO-d6, 126 MHz): δ 130.23, 129.60, 128.97, 128.32, 126.78, 126.17, 121.20, 115.71. IR (KBr) 3430, 3261, 2923, 2246, 1629, 1444, 1164, 1087, 574, 480 cm-1. MS (EI) m/z (%): 169 (M+, 100). HRMS (EI) calcd. for C10H7N3,169.0640; found 169.0637.
5-(p-Tolyl)-1H-pyrazole-3-carbonitrile (2e).
White powder, mp 210–212 °C. 1H NMR (CDCl3, 300Hz): δ 2.40 (s, 3H), 6.87 (s, 1H), 7.29 (d, 2H, J = 8.1 Hz), 7.46 (d, 2H, J = 7.7Hz). 13C NMR (DMSO-d6, 126 MHz): δ 144.54, 139.32, 130.20, 126.39, 125.87, 125.02, 115.33, 107.98, 20.99. IR (KBr) 3428, 2954, 2852, 2242, 1631, 1461, 1186, 1079, 518 cm-1. MS (EI) m/z (%): 183 (M+, 100). HRMS (EI) calcd. for C20H21N3O, 183.0796; found 183.0791.
5-(4-Methoxyphenyl)-1H-pyrazole-3-carbonitrile (2f).
White powder, mp 93–95 °C. 1H NMR (DMSO-d6, 300Hz): δ 3.78 (s, 3H), 7.00 (d, 1H, J = 8.6 Hz), 7.01 (d, 1H, J = 8.4 Hz), 7.69 (d, 1H, J = 8.8 Hz), 7.75 (d, 1H, J = 8.8 Hz), 13.36 (brs, 1H). 13C NMR (DMSO-d6, 126 MHz): δ 160.38, 144.32, 127.54, 125.08, 120.52, 115.43, 115.05, 107.39, 55.75. IR (KBr) 3400, 3145, 2948, 2864, 2242, 1619, 1515, 1465, 1255, 1180, 1018, 833, 526 cm-1. MS (EI) m/z (%): 199 (M+, 100). HRMS calcd. for C11H9N3O, 199.0746; found 199.0745.
5-(4-Chlorophenyl)-1H-pyrazole-3-carbonitrile (2g).
White powder, mp 160–162 °C. 1H NMR (DMSO-d6, 300Hz): δ 7.17 (s, 1H), 7.52 (d, 2H, J = 6.7 Hz), 7.79 (2H, d, J = 6.7 Hz), 13.67 (brs, 1H). 13C NMR (DMSO-d6, 126 MHz): δ 142.96, 133.75, 129.24, 129.06, 127.31, 126.19, 114.58, 108.32. IR (KBr) 3432, 3141, 2927, 2848, 2244, 1608, 1490, 1187, 1059, 1012, 819, 516 cm-1. MS (EI) m/z (%): 205 (31), 203 (M+, 100). HRMS (EI) calcd. for C10H6N3Cl. 203.0256; found 203.0246.
5-(4-Cyanophenyl)-1H-pyrazole-3-carbonitrile (2h).
White powder, mp 270–272 °C. 1H NMR (DMSO-d6, 300Hz): δ 7.64 (s, 1H), 7.98 (d, 2H, J = 8.0 Hz), 8.09 (d, 2H, J = 8.0 Hz). 13C NMR (DMSO-d6, 126 MHz): δ 143.11, 133.67, 133.65, 130.12, 126.73, 125.21, 118.49, 111.27, 109.68. IR (KBr) 3430, 2917, 2242, 2227, 1614, 1502, 997, 842, 551 cm-1. MS (EI) m/z (%): 194 (M+, 100). HRMS (EI) calcd. for C11H6N4, 194.0592; found 194.0604.
5-(4-Nitrophenyl)-1H-pyrazole-3-carbonitrile (2i).
White powder, mp 231-233 °C. 1H NMR (DMSO-d6, 300Hz): δ 7.67 (s, 1H), 8.33 (dd, 2H, J = 7.1, 2.0 Hz), 8.36 (dd, 2H, J = 8.9, 1.2 Hz). 13C NMR (DMSO-d6, 126 MHz): δ 147.31, 141.83, 133.48, 126.58, 125.13, 124.52, 114.48, 107.59. IR (KBr) 3428, 3143, 2919, 2850, 2244, 1606, 1513, 1349, 1113, 995, 856, 568 cm-1. MS (EI) m/z (%): 214 (M+, 100). HRMS (EI) calcd. for C10H6N4O2, 214.0491; found 214.0491.
5-(3-Nitrophenyl)-1H-pyrazole-3-carbonitrile (2j).
White powder, mp 190–192 °C. 1H NMR (DMSO-d6, 300Hz): δ 7.67 (s, 1H), 7.80 (t, 1H, J = 8.0 Hz), 8.25 (t, 2H, J = 8.5 Hz), 8.70 (s, 1H). 13C NMR (DMSO-d6, 126 MHz): δ 148.89, 142.90, 132.37, 131.33, 129.9, 124.10, 124.09, 120.49, 114.66, 109.95. IR (KBr) 3517, 3421, 2252, 1629, 1517, 1361, 1012, 800, 740, 572 cm-1. MS (EI) m/z (%): 214(M+, 100). HRMS (EI) calcd. for C10H6N4O2, 214.0491; found 214.0488.
5-(4-(Trifluoromethyl)phenyl)-1H-pyrazole-3-carbonitrile (2k).
White powder, mp 170–172 °C. 1H NMR (DMSO-d6, 300Hz): δ 7.60 (s, 1H), 7.86 (d, 2H, J = 8.2 Hz), 8.10 (d, 2H, J = 8.2 Hz). 13C NMR (DMSO-d6, 126 MHz): δ 143.58, 132.27, 126.75, 129.13, 126.75, 126.61, 125.56, 114.86, 109.81. IR (KBr) 3430, 2919, 2250, 1627, 1326, 1062, 557, 472 cm-1. MS (EI) m/z (%): 237(M+, 100). HRMS (EI) calcd. for C11H6N3F3, 237.0514; found 237.0514.
5-(4-(Methylsulfonyl)phenyl)-1H-pyrazole-3-carbonitrile (2l).
White powder, mp: 205–207 °C. 1H NMR (DMSO-d6, 300Hz): δ 3.27 (s, 3H), 7.63 (s, 1H), 8.05 (d, 2H, J = 8.1 Hz), 8.11 (d, 2H, J = 8.3 Hz). 13C NMR (DMSO-d6, 126 MHz): δ 143.41, 141.15, 133.05, 128.29, 126.91, 125.39, 114.84, 110.09, 43.83. IR (KBr) 3430, 3143, 2921, 2240, 1633, 1298, 1153, 779, 536 cm-1. MS (EI) m/z (%): 247 (M+, 100). HRMS (EI) calcd. for C11H6N3O2S, 247.0514; found 247.0416.
4,5,6,7-Tetrahydro-1H-indazole-3-carbonitrile (2m).
White powder, mp 145–147 °C. 1H NMR (CDCl3, 300Hz): δ 2.60–2.64 (m, 4H), 2.71–2.75 (m, 4H), 7.26 (s, 1H), 11.77 (brs, 1H). 13C NMR (DMSO-d6, 126 MHz): δ 140.56, 121.94, 120.58, 115.32, 22.52, 22.17, 20.62, 19.58. IR (KBr) 3432, 2919, 2237, 1633, 1068, 468 cm-1. MS (EI) m/z (%): 147 (M+, 100). HRMS (EI) calcd. for C8H9N3, 147.0796; found 147.0797.
3-Hydroxy-estra-1,3,5(10)-triene[17,16-c]pyrazole-5'-carbonitrile (2n).
White powder, mp 221–222 °C. 1H NMR (CDCl3, 300Hz): δ 7.14 (d, 1H, J = 9.2 Hz), 6.60 - 6.72 (m, 2H), 1.00–3.00 (m, 13H), 0.93 (s, 3H). 13C NMR (DMSO-d6, 126 MHz): δ 167.43, 159.88, 155.51, 137.45, 132.15, 132.00, 129.13, 127.92, 118.36, 115.46, 44.07, 41.84, 37.43, 31.62, 30.43, 27.23, 23.97, 22.57, 19.12, 14.03. IR (KBr) 3426, 2923, 2242, 1616, 1079, 561 cm-1. MS (EI) m/z (%): 319 (M+, 80), 159(M+, 100). HRMS (EI) calcd. for C20H21N3O, 319.1685; found 319.1678.
Crystallography. Colorless crystals of 2c were obtained by recrystallization in MeOH, The crystal data was collected on a Bruker SMART CCD detector employing graphite monochromated Mo-Kα radiation (λ = 0.71073 Å) at 293 K and operating in the φ-ω scan mode. The structure was solved by direct methods and refined with full-matrix least-squares calculations on F2 using SHELX-97. All non-hydrogen atoms were refined anisotropically. The hydrogen atom positions were geometrically idealized and allowed to ride on their parent atoms. Crystal data for 5-Phenyl-1H-pyrazole-3-carbonitrile (2c): C10H7N3, M = 169.19, white needle, 0.285 x 0.127 x 0.115 mm3, Rhombohedral, space group R3, a = 32.877 (5) Å, b = 32.877 (5) Å, c = 4.4202 (8) Å, α = 90 °, β = 90 °, γ = 120 °, V = 4137.6 (11) Å3, Z = 18, Dcalc = 1.275 mg/m3, 1816 unique reflections, R1 = 0.0573, wR2 = 0.1296, GOF = 0.937. CCDC-848480 contains the supplementary crystallographic data for this compound. Copies of these data can be obtained free of charge via www.ccdc.cam.ac.uk/conts/retrieving.html (or from the Cambridge Crystallographic Data Centre, 12, Union Road, Cambridge CB21EZ, UK. [fax: (+44) 1223-336-033; or email: deposit@ccdc.cam.ac.uk).
ACKNOWLEDGEMENTS
The authors are grateful to the National Natural Science Foundation of China (20772138, 90713034), and Shanghai Municipality Science and Technology Development Fund (09JC1416600) for financial support.
References
1. A. Sun, N. Chandrakumar, and J. J. Yoon, Bioorg. Med. Chem. Lett., 2007, 17, 5199. CrossRef
2. A. Wahhab, M. Allan, S. Manku, and E. Therrien, Bioorg. Med. Chem. Lett., 2009, 19, 1218. CrossRef
3. A. V. Purandare, T. Huynh, and Z. Chen, Bioorg. Med. Chem. Lett., 2009, 19, 2924. CrossRef
4. D. J. P. Pinto, M. J. Orwat, and M. L. Quan, Bioorg. Med. Chem. Lett., 2006, 16, 4141. CrossRef
5. G. Daidone, B. Maggio, D. Raffa, and M. V. Raimondi, Eur. J. Med. Chem., 2008, 43, 2386. CrossRef
6. J. L. Masferrer, J. M. O'Neal, and J. M. Oneal, WO2004/014352 A2.
7. D. M. Bradley, W. N. Chan, S. Harrison, and R. Thatcher, WO2007/107539 A1.
8. D. M. Bradley, W. N. Chan, K. M. Thewlis, and S. E. Ward, WO2008/053031 A1.
9. D. M. Bradley, K. M. Thewlis, and S. E. Ward, WO2008/148832 A1.
10. Y. Yonetoku, H. Kubota, and Y. Miyazaki, Bioorg. Med. Chem., 2008, 16, 9457. CrossRef
11. Y. Yonetoku, H. Kubota, and Y. Okamoto, Bioorg. Med. Chem., 2006, 14, 4750. CrossRef
12. K. Ohsumi, H. Matsueda, and T. Hatanaka, Bioorg. Med. Chem. Lett., 2003, 13, 2269. CrossRef
13. F. F. Fleming, L. H. Yao, P. C. Ravikumar, and L. Funk, J. Med. Chem., 2010, 53, 7902. CrossRef
14. R. L. Dow, J. R. Hadcock, and D. O. Scott, Bioorg. Med. Chem. Lett., 2009, 19, 5351. CrossRef
15. A. M. Gonzalez-Nogal, M. Calle, P. Cuadrado, and R. Valero, Tetrahedron., 2007, 63, 224. CrossRef
16. J. L. G. Ruano, S. A. A. de Diego, and D. Blanco, Org. Lett., 2001, 3, 3173. CrossRef
17. H. Kimoto and L. A. Cohen, J. Org. Chem., 1979, 44, 2902. CrossRef
18. H. Kimoto and L. A. Cohen, J. Org. Chem., 1980, 45, 3831. CrossRef
19. P. M. Donald, P. W. Jeffrey, and R. M. James, J. Org. Chem., 1986, 51, 3228. CrossRef
20. D. P. Matthews, J. R. McCarthy, J. P. Whitten, and P. R. Kastner, J. Med. Chem., 1990, 33, 317. CrossRef
21. C. Ferstner, H. Schirok, ans N. Griebenow, US2010113507 A1. CrossRef
22. R. G. Pews, J. A. Gall, and J. C. Little, J. Fluorine Chem., 1990, 50, 365. CrossRef
23. Yagupol'skii, Zh. Org. Khim., 1977, 13, 2388.
24. J. C. Sloop, C. L. Bumgardner, and W. D. Loehle, J. Fluorine Chem., 2002, 118, 135. CrossRef
25. J. C. Sloop, C. L. Bumgardner, and G. Washington, J. Fluorine Chem., 2006, 127, 780. CrossRef
26. T. Nishiwaki, Bull. Chem. Soc. Jpn., 1969, 42, 3024. CrossRef
27. C. Fuerstner, H. Schirok, N. Griebenow, J. Mittendorf, and J. Stasch, WO2007/128454 A1.
28. H. Berber, M. Soufyane, C. Mirand, and S. Schmidt, Tetrahedron., 2001, 57, 7369. CrossRef
29. C. Mirand, A. Touzot, M. Soufyane, and H. Berber, J. Fluorine Chem., 2004, 125, 1299. CrossRef
30. M. A. Martins, N. Zanatta, H. G. Bonacorso, and F. A. Rosa, ARKIVOC, 2006 , iv, 29.